Translate this page into:
Fluorine-free foaming extinguishing agent: Design route, fire extinguishing performance, foam stability mechanism
⁎Corresponding author. jiahailin@hpu.edu.cn (Hailin Jia)
-
Received: ,
Accepted: ,
This article was originally published by Elsevier and was migrated to Scientific Scholar after the change of Publisher.
Peer review under responsibility of King Saud University.
Abstract
The development of fluorine-free foaming extinguishing agent, abbreviation FFEA, has become an urgent scientific challenge in the field of fire protection engineering due to the fact that the key component of existing aqueous film-forming foams is listed as a persistent organic pollutant. A study was conducted on foam performance and fire extinguishing efficiency of FFEA prepared by hydrocarbon and silicone surfactants with low-carbon alcohol modulation, using three selected silicone surfactants. The results show that the FFEA, including low-carbon alcohol-modulated Silok8141/SDS and Silok8008/SDS, exhibit low surface tension, good foam stabilization coefficient and long foam drainage time. Laboratory foam extinguishing experiments demonstrate that the FFEA produced by Silok8141/SDS/Isobutanol ternary system has better cooling effect and fire extinguishing performance. And the study of FFEA mechanism reveals that the addition of isobutanol blocks the foam liquid channel and increases the foam stability. The wide applicability of the design route for FFEA prepared by low-carbon alcohol-regulated hydrocarbon and silicone surfactants has been demonstrated.
Keywords
Fluorine-free foaming extinguishing agent
Silicone surfactant
Design route
Extinguishing efficiency
Foam stability mechanism
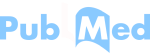
Nomenclature
- FFEA
-
fluorine-free foaming extinguishing agent
- CMC
-
critical micelle concentration
- ST
-
surface tension /nM·m−1
- MF
-
mass fraction /wt%
- FH
-
foaming height /mm
- FSC
-
foam stabilization coefficient
- 25 %DT
-
25 % drainage time /s
- ER
-
expansion ratio /%
- FDP
-
foam drainage percentage /%
- ADR
-
average drainage rate /%·s−1
Abbreviations
1 Introduction
Fire safety plays a crucial role in people's daily lives, where foam extinguishing agents are primarily utilized to extinguish Class B fires and some Class A fires(Khan et al., 2021; Pan et al., 2020; Wu et al., 2021). The foam extinguishing agents work by creating an oxygen barrier, heat barrier through the foam cover layer and cooling effect of water(Ananth et al., 2019; Jia et al., 2022; Sheng et al., 2018). To gain a better understanding the characteristics of foam extinguishing agents, the researchers primarily investigate the properties such as the surface tension(Baek and Yong, 2020; Ma et al., 2023; Shojaei et al., 2021), foaming ability(Yang et al., 2023) and fire extinguishing performance(Kang et al., 2019; Szymczyk et al., 2021; Szymczyk and Jańczuk, 2010; Xu et al., 2020).
Fluorocarbon surfactants(Szymczyk et al., 2022; Yang et al., 2023; Yu et al., 2022); (Peng et al., 2018; Sheng et al., 2020) a crucial constituent of current aqueous film-forming foams (AFFF) - have been classified as persistent organic pollutants (POPs) due to their challenging degradability, long-range migration and bioaccumulative effects(Alshemmari, 2021; Fuentes et al., 2006; van der Veen et al., 2023). These factors potentially impact the subsequent use of AFFFs. Consequently, the AFFF containing predominantly fluorocarbon surfactants are gradually being phased out by fire services(Hetzer et al., 2014). This will inevitably impact the petrochemical industry, posing significant implications and challenges for fire safety and emergency response. Therefore, there is an urgent need to search for environmentally friendly fluorine-free foams with better firefighting capabilities.
In order to address the issues of environmental pollution, inefficiency in foam fire extinguishing agents(Bourgeois et al., 2015; Wang, 2015; Yu et al., 2020), researchers have been continuously exploring solutions. Andrei Bureiko(Bureiko et al., 2015) found that foams used in everyday life such as shampoos and detergents were mainly made from a mixture of different ingredients. The correct choice of two or more active ingredients could accelerate foam formation and improve foam stability. Sodium dodecyl sulfate (SDS) is widely used as a foaming agent in many fields because of its low price, biodegradability, and good foaming ability(Lu et al., 2022; Qiao et al., 2016; Sett et al., 2015; Wang et al., 2015; Zhou and Ranjith, 2021). For example, Zhou et al.(Zhou et al., 2022) found that the compound of anionic hydrocarbon surfactant, SDS, and nonionic short-chain fluorocarbon surfactant had good physical and chemical properties and wetting ability. Kang et al.(Kang et al., 2021) found that the addition of xanthan gum and gelatin resulted in a more homogeneous foam with a less negative impact on surface tension. Shah et al.(Kumar Shah et al., 2022) investigated that the cmc values of binary mixtures containing SDS were lower than those of single surfactant solutions and that the binary mixtures showed better foaming ability and good foam stability than single surfactant. Zhang et al.(Zhang et al., 1999) tested the role of a series of silicone surfactants with different structures in flexible polyurethane foam. The results showed that silicone could reduce the surface tension of the system and the drainage rate of cell windows. Sheng et al.(Sheng, 2018) found that the foaming ability and foam stability of silicone surfactant combined with hydrocarbon surfactant were stronger than those of single surfactant.
The binary system foam compounded with hydrocarbon and silicone still suffers from short half-life, poor liquid-holding capacity, and rapid foam aggregation(Hetzer et al., 2014). However, low-carbon alcohols as additives can slow down the drainage process of the compound system, Bhattarai(Bhattarai, 2015)found that at different temperatures, with the increase of methanol concentration of the mixed solution cmc will be increased. Zdziennicka(Zdziennicka and Jańczuk, 2020) found that the addition of short-chain alcohols to the aqueous surfactant solution can create a lower polarity and better solubility environment for surfactants. Jia et al. (Jia et al., 2022) found that appropriate concentrations of low-carbon alcohols could enhance the foam stability of the binary system of silicone surfactant LS-99 and hydrocarbon surfactant SDS. Based on this, we intend to study an environmentally friendly and efficient fluorine-free foam extinguishing agent with high foam stability and strong liquid-holding capacity with low-carbon alcohols, silicone surfactants and hydrocarbon surfactants as the basis.
The main objective of this study was used to verify the wide applicability of the design route for the preparation of non-fluorinated foam fire extinguishing agent with low carbon alcohol modulated silicone/hydrocarbon surfactant. The author intends to introduce three low-carbon alcohols, ethanol, n-propanol and isobutanol, select three silicone surfactants and three hydrocarbon surfactants, from which two FFEA formulations are preferred. And then analyzed the surface tension, foaming and foam stabilizing ability, foaming times, drainage time and other foam parameters of the low-carbon alcohol/silicone/hydrocarbon surfactant mixture system. Conducted experiments with two FFEA formulations to extinguish large-scale oil pool fires. Finally, the mechanism study of low-carbon alcohol-modified silicone/hydrocarbon surfactant compounding system was carried out. The foam stability of the two FFEA formulations was quantitatively investigated, and the extinguishing mechanism of FFEA for rapidly extinguishing oil pool fires was verified.
2 Raw materials and experimental equipment
2.1 Raw materials
The aim of this study is to replace the fluoride component of traditional foam extinguishing agents with a fluorine-free foam extinguishing agent (hereinafter collectively referred to as FFEA) using a low-carbon alcohol-moderated hydrocarbon surfactant and silicone surfactant compound system. SDS, BS-12 and 1227 were selected for the hydrocarbon surfactants. SDS was purchased from Guangdong Gaoshun Chemical Import & Export Co., Ltd, China. BS-12 was purchased from Shanghai Handeyunjia New Material co., Ltd, China. 1227 was purchased from Shandong Xinsunjie Chemical Technology Co., Ltd, China. Silicone surfactants (Silok8141、Silok8000、Silok8008), were purchased from Guangzhou Silok Polymers Co., Ltd, China. Silok8141 and Silok8000 both contain polyether modified silicone oil (molecular formula C12H32O4Si3). But C12H32O4Si3 in Silok8141 accounted for 65 % −70 %, auxiliary materials for propylene oxide accounted for 2 % −5%. While Silok8000 has a greater concentration of C12H32O4Si3 accounting for more than 90 %, the auxiliary material is C15H32O2 accounting for 1 %-5%.Silok8008′s main ingredient is Octamethyltrisiloxane accounting for 90 %-95 %, the auxiliary material is C15H40O5Si4 accounting for 3 %-10 %.Based on previous research, it was known that the surface tension of alcohols increased as the length of the carbon chain decreased(Zdziennicka and Jańczuk, 2020) which affected the compounded system's surface activity. The alcohols chosen for the experiment were ethanol, n-propanol and isobutanol. The specific composition is shown in Table 1.
Raw materials
Main components (Mass fraction%)
Silok8141
C12H32O4Si3 65–70 %
Propylene oxide 2 %-5%
Silok8000
C12H32O4Si3 90–95 %
C15H32O2 1–5 %
Silok8008
Octamethyltrisiloxane 90–95 %
C15H40O5Si4 3–10 %
SDS
Sodium dodecyl sulfate 98.5 %
BS-12
2-(Dodecyl dimethyl ammonio)acetate 98 %
1227
Dodecyl dimethyl benzyl ammonium chloride 98.5 %
Ethanol
Ethanol 99.7 %
n-Propanol
n-Propanol 99 %
Isobutanol
Isobutanol 99 %
2.2 Surface tension meter and Roche foam meter
Surface tension was measured by means of the surface tension meter named BZY-101. The surface tension meter was purchased from Shanghai Fang Rui Instrument Co., Ltd., China. The foaming height and foam stabilization capacity were determined using Roche foam meter named 2151. Roche foam meter was purchased from Beijing Hifuda Technology Co., Ltd., China. Metrological characteristics are shown in Table 2.
Experimental equipment
Measurement range
Measurement accuracy
Accuracy error
Temperature range of specimen
Surface tension meter
0-600mN/m
0.1mN/m
± 0.1mN/m
0-120 °C
Roche foam meter
0–900 mm
1 mm
± 1mm
0-100 °C
Electronic balance
0–500 g
0.01 g
± 0.02 g
0-70 °C
The surface tension was determined by placing the solution to be measured on the observatory. The table was raised by turning the knob and stops rising when the liquid level was just in contact with the platinum plate. At this point the data on the screen was the surface tension.
For the determination of foaming height and foam stability coefficient, 50 ml of configuration solution was pre-absorbed in a glass tube, and 200 ml of configuration solution was absorbed by using a quantitative funnel, and the solution in the quantitative funnel was completely discharged at a height of 1 m from the liquid surface. At this point the foam height in the tube was recorded as h0, and after standing for 5mins the foam height in the glass tube was recorded again as h5, and the resulting h5 divided by h0 was the foam stabilization coefficient.
2.3 Measuring devices of 25 % drainage time and expansion ratio
Measuring device of 25 % drainage time is shown in Fig. 1, consisting of an electronic balance, a beaker, a conical glass funnel, a glass beads and a computer. Firstly, the foam is completely foamed using an air compressor. 20 g of foams were placed in a glass funnel filled with glass beads, and a beaker was placed in its lower part to receive the liquid precipitated by the foam. The electronic balance was connected to a computer and the change in mass of the precipitated liquid was automatically read and recorded by software.Measuring device of 25 % drainage time.
The expansion ratio was determined by means of a measuring cylinder and an electronic balance. The foam was first completely foamed using an air compressor, a volume of foam was measured using a measuring cylinder, the mass of foam was weighed using an electronic balance and the resulting volume was divided by the mass to obtain the expansion ratio.
2.4 Test platform of fire extinguishing effect
The fire extinguishing system and the fire extinguishing experiment site are shown in Fig. 2 and Fig. 3. The fire extinguishing platform is mainly composed of a foam generation system and a flame temperature acquisition device. In the experiment, the foam premix was stored in the water tank and brought into the pipeline through the power provided by the air compressor, the driving pressure of the air compressor was 0.7Mpa, and the maximum flow rate of the foam gun was 5 L/min. The premixed liquid generates foam by impact in the foam gun and is sprayed to the center of the burning oil pan. The distance between the foam gun and the oil pan is 3 m. The flame temperature acquisition device is mainly composed of thermal imager, camera, thermocouple and paperless recorder. During the experiment, 2000 ml of water and 5000 ml of gasoline were poured into an oil pool with a diameter of 920 mm in turn. And 30000 ml of foam premix is pre-configured in the tank for foam fire extinguishing. After ignition, the oil fire was fully pre-burned for 60 s. Then the air compressor valve was opened and the fire was extinguished. Type K thermocouples are arranged on the center axis of the flame and the ends are connected to a computer to collect the flame temperature. The thermocouples and infrared camera will continue to collect the flame temperature from the time before ignition begins until the end of the experiment.Foam extinguishing system.
Fire extinguishing experiment site.
2.5 Quantitative analysis of foam stability
The quantitative analysis of foam experiments used in the experimental apparatus Automatic FOAMSCAN as shown in Fig. 4, purchased from TECLIS SCIENTIFIC, France. 60 ml of foam was foamed using an air compressor and taken in a beaker, the time interval of the automatic photo was set to 2 s in the FOAMSCAN, and the bubble size and distribution were analyzed in the CSA software after the completion of the picture acquisition. The experimental environment was at room temperature, and the foam size was collected from 35 μm to 2000 μm.Automatic FOAMSCAN.
3 Study of low-carbon alcohol modulated ternary systems
3.1 Research on hydrocarbon/ silicone surfactant binary system
3.1.1 Screening of hydrocarbon surfactants
The variation of surface tension of Silok8141 compounded with hydrocarbon surfactants were shown in Fig. 5. Observation of Fig. 5(a) reveals that when the mass fraction of silicone surfactant was 0 %, the solution was a single system of hydrocarbon surfactant 1227, and the surface tension decreased rapidly with the increase of the mass fraction of 1227 when the mass fraction was less than 0.05 %, and then the surface tension almost no longer varied with the increase of the mass fraction of 1227, and was stabilized at 32.4 mN/m, and the critical micelle concentration cmc was 0.033 %. The trends of single hydrocarbon surfactant curves in Fig. 5(b) and 5(c) were similar to those in Fig. 5(a), in which the surface tension of BS-12 was stabilized at 30.1 mN/m with a cmc of 0.015 %, and that of SDS was stabilized at 29.6 mN/m with a cmc of 0.0392 %. Observing Fig. 5(a), Fig. 5(b) and Fig. 5(c), it can be found that the trends of the three curves were similar with the increase of hydrocarbon surfactant mass fraction at different silicone surfactant mass fractions. Therefore, taking Fig. 5(a) as an example, when the mass fraction of silicone was 0.001 % and the mass fraction of 1227 was less than 0.01 %, the surface tension of the binary system gradually decreased with the increase of the mass fraction of hydrocarbon surfactant. This is because when the concentration of silicone surfactant is less than cmc, the number of surfactant molecules adsorbed on the foam liquid film is less, and the addition of hydrocarbon surfactant molecules can make up for the lack of silicone active agent molecules, so that the surface tension further decreases(Bhattarai, 2020; Jiang et al., 2015). When the mass fraction of organosilicon surfactant was 0.01 % and 0.1 % with the increase of 1227 mass fraction, the surface tension curve of the binary system is stable at first and then increases slowly. This is because the mass fraction of silicone surfactant, which is dominant in the adsorption process, is getting higher and higher, while the addition of hydrocarbon surfactant and silicone surfactant forms competitive adsorption. (Graciaa et al., 2003; Yu et al., 2022). With the increase in the mass fraction of hydrocarbon surfactant, the competitive adsorption effect is enhanced, and the surface tension rises slowly. The lowest surface tension of the formulated systems was found when the silicone surfactant mass fraction was 0.1 % and the hydrocarbon surfactant mass fraction was 0.01 %. The lowest surface tension was 22.7 mN·m−1 for the 1227 compound system, 22.8 mN·m−1 for the SDS compound system and 22.7 mN·m−1 for the BS-12 compound system.Surface tension of Silok8141/Hydrocarbon surfactants.
The changes of foaming height of Silok8141 and hydrocarbon surfactant compound system and hydrocarbon surfactant single system were shown in Fig. 6. The black line represented the hydrocarbon surfactant single system, the three hydrocarbon surfactant foaming height with the change of mass fraction trend was similar to Fig. 6 (a), for example, when the 1227 additive amount was lower than 0.1 %, with the increase in 1227 mass fraction of the monomer system foaming height increased rapidly, when the 1227 additive amount was greater than 0.1 %, with the increase in 1227 mass fraction of the foam height increase slowed down, the foaming height curve tends to stable. 1227 single system foaming height increase rate slowed down. Height increase rate slows down, the image tended to flatten. 1227 single system foaming height of up to 151 mm, BS-12 up to 147 mm, SDS up to 158 mm. With the increase of silicone surfactant the foaming height of the binary system changed. Observing Fig. 6 (a), it can be seen that when the mass fraction of 1227 was less than 0.5 % the addition of silicone surfactant was able to increase the foaming height of the compounded system, and when it was over 0.5 %, there was not much difference in foaming ability of the binary system and that of the single system of 1227.Foaming heights of Silok8141/Hydrocarbon Surfactants.
Observation of Fig. 6(b) revealed that when the mass fraction of silicone surfactant was 0.001 % and 0.01 %, and the mass fraction of BS-12 was less than 0.01 %, the addition of silicone surfactant had less effect on the foaming performance of BS-12. When the mass fraction of BS-12 was greater than 0.5 %, the foaming ability of the binary system was stronger than that of the single system of BS-12, and the maximum foaming heights reached 156 mm, 157 mm and 161 mm at the additions of 0.001 %, 0.01 % and 0.1 % of silicone surfactants, respectively, with the enhancement of 6.12 %, 6.80 % and 9.52 %, respectively, in comparison with that of the single system.
Observing Fig. 6(c), it was found that the foaming performance of the binary system was significantly improved compared with that of the SDS single system, and the foaming height of the binary system exceeded that of the SDS single system at a mass fraction of SDS greater than 0.01 %.At 0.001 %, 0.01 % and 0.1 % silicone surfactant additions, the maximum foaming heights reached 182 mm, 165 mm and 191 mm, respectively, which were 15.19 %, 4.43 % and 20.89 %, compared with that of the SDS single system, respectively.
The foam stability coefficient is used to evaluate the stability of the foam, and a sufficiently stable foam layer can ensure the shielding effect on combustibles for a long period of time and improve the fire extinguishing efficiency(Ping et al., 2023). The variation of foam stabilization coefficient of Silok8141/hydrocarbon surfactant compound system was shown in Fig. 7. Observing Fig. 7(a), it can be seen that the foam stabilization of single 1227 is poor when the mass fraction is less than 0.001 %, and the foam stabilization coefficient increases and then decreases with the increase of the mass fraction of 1227. With the addition of organosilicon surfactant, the foam stability of the binary system was significantly improved, and the stabilization coefficient of the binary system showed a slow increase when the mass fraction of 1227 was less than 0.05 %, and a significant decrease in the stabilization coefficient occurred when the mass fraction of 1227 was greater than 0.01 %. Observation of Fig. 7(b) reveals that the foam stability of the single system BS-12 is equally poor at low mass fraction. When the mass fraction of BS-12 was greater than 0.001 %, the foam stabilization ability increased dramatically and eventually stabilized around 0.8420. With the addition of Silicone surfactants, when the mass fraction of BS-12 was less than 0.1 %, all the three additions of Silicone surfactants could improve the foam stabilization ability of BS-12, and the stabilization coefficient increased dramatically. When the addition of Silicone surfactants was 0.001 % versus 0.01 % and the mass fraction of BS-12 was greater than 0.1 %, the stabilization coefficient of foam stabilization decreased slightly.Foam stabilization coefficient of Silok8141/Hydrocarbon Surfactants.
Observation of Fig. 7(c) showed that the foam stability of single SDS solution with low mass fraction was poor, and the stabilization coefficient of single system SDS solution reached 0.9000 only when the mass fraction of SDS was 0.5 % and 1 %.The addition of 0.001 %, 0.01 % and 0.1 % mass fraction of Silicone surfactants can greatly improve the stabilization coefficient of the binary system, which solved the phenomenon that the stabilization coefficient of the single system with low mass fraction of SDS was too low. When the mass fraction of SDS reached 0.01 %, the stabilization coefficient of the binary system under the three silicone surfactants exceeded 0.9310, and the single SDS was only 0.117, the stabilization coefficient was increased by more than 695.7 %, and the stabilization ability was greatly improved. At the same time, when the SDS mass fraction was higher, the binary system could still maintain strong foam stability, up to 0.9890.
3.1.2 Research on hydrocarbon/ silicone compound system
From the experiments in 3.1.1, it was found that all the three hydrocarbon surfactants showed good synergistic effect on surface tension when compounded with silicone, while SDS showed better foaming ability as well as foam stability when compounded with silicone. Therefore, in this section, SDS was used in combination with three silicones (Silok8141, Silok8008 and Silok8000), Where the concentration of SDS is taken as 0.04 % mass fraction at the value of CMC(Hailin Jia et al., 2022).
According to the experimental determination, the surface tension, foaming height and foam stabilization coefficient of the three silicone surfactants compounded with SDS are shown in Table 3. Observation of Table 3 reveals that with the increase of silicone surfactant mass fraction, the compounding system foam surface tension also decreased, when the silicone mass fraction of close to 0.1 %, the rate of decline slows down. When the mass fraction of Silok8141 and Silok8008 is 0.1 %, the lowest surface tension of the compound system is 22.4 mN·m−1 and 23.6 mN·m−1, respectively, and the lowest surface tension of the compound system is 21.7 mN·m−1 when the mass fraction of Silok8000 reaches 0.5 %.
Mass concentration of silicone
Silok8141/SDS
Silok8008/SDS
Silok8000/SDS
Tension /mN·m−1
Height /mm
Foam stability coefficient
Tension /mN·m−1
Height /mm
Foam stability coefficient
Tension /mN·m−1
Height /mm
Foam stability coefficient
0.0001 %
28.0
52
0.844
29.3
32
0.875
27.9
27
0.889
0.0005 %
26.7
73
0.853
27.9
36
0.889
25.6
30
0.900
0.001 %
25.3
91
0.870
25.7
39
0.897
24.7
56
0.893
0.005 %
24.3
107
0.896
25.4
58
0.912
23.8
58
0.914
0.01 %
23.1
126
0.925
25.1
54
0.926
22.4
62
0.919
0.05 %
22.7
134
0.960
24.6
55
0.949
21.9
44
0.932
0.1 %
22.4
145
0.958
23.6
56
0.951
21.8
32
0.938
0.5 %
22.4
152
0.976
23.5
47
0.953
21.7
16
0.938
1 %
22.3
154
0.969
23.7
46
0.952
21.8
21
0.932
The three formulations showed differences in foaming height. When the silicone surfactant mass fraction in the 0.0001 % to 0.01 % interval, with the increase in silicone mass fraction Silok8000/SDS system foaming height also increased, to the maximum of 62 mm. When the mass fraction of silicone is more than 0.01 %, with the increase in the mass fraction of Silok8000, the foaming capacity of binary system is reduced, and the foaming height is reduced to varying degrees. The foam trend of Silok8008 system is similar to that of Silok8000, when the silicone mass fraction is 0.005 %, the foam height of the compound system is 58 mm, then with the increase of silicone mass fraction, the foam height of the compound system decreases slowly and finally stabilizes at 42 mm. While Silok8141/SDS system foam with the increase of silicone mass fraction, when Silok8141 mass fraction between 0.0001 % and 0.05 %, the binary system foaming capacity grows faster, when the Silok8141 mass fraction is greater than 0.05 %, with the increase of silicone mass fraction (0.1 %, 0.5 %, 1 %) binary system foaming height tends to stabilize.
The trend of stability coefficient of the three silicone surfactants and SDS compounded systems can be seen that with the increase of the mass fraction of Silicone surfactants, the binary system of the three Silicone surfactants compounded with SDS showed an upward trend with the increase of the mass fraction of Silicone surfactants. When the mass fraction of Silicone surfactants was 0.0001 %, the foam stability of Silok8141/SDS system was the worst, and the stabilization coefficient was the lowest at 0.844. With the increase of the mass fraction of Silicone surfactants, the stabilization coefficient of Silok8141/SDS system increased rapidly. When the mass fraction of silicone surfactants reached 0.05 %, the foam stabilization coefficient exceeded that of Silok8008/SDS and Silok8000/SDS systems and continued to increase with the increase of mass fraction. When the mass fraction of silicone surfactants for 1 %, Silok8141/SDS, Silok8008/SDS and Silok8000/SDS system stabilization coefficients were 0.969, 0.952 and 0.932, respectively.
3.2 Ternary systems
According to the experimental data in 3.1, it could be found that the foam foaming ability and the foam stability of Silok8000-containing system was poor. In contrast, the synergistic effect of Silok8141 and Silok8008 compounded with SDS was better, with lower surface tension, better foaming height and better foam stability, especially when Silok8141 and Silok8008 mass fraction of 0.1 % with SDS (mass fraction of 0.04 %) compounding effect is more effective, therefore, the mass fraction of the two silicone surfactants in the subsequent experiments was chosen to be 0.1 %, and the mass fraction of SDS was 0.04 %. From Table.4, after the introduction of low carbon alcohols into the Silok8141/SDS system, the surface tension of the foam of the ternary system decreased slightly with the increase of the mass fraction of low-carbon alcohols, and the maximum value of the reduction of the surface tension was 0.7 mN·m−1 when the mass fraction of isobutanol was 0.05 %. Similarly, the surface tension of the Silok8008/SDS system decreased more significantly after the introduction of the lower carbon alcohols. When the mass fraction of isobutanol was 0.05 %, the maximum decrease of surface tension was 2.0 mN·m−1. This is due to the fact that the addition of low-carbon alcohols reduces the electrostatic repulsion between surfactant molecules, which leads to a small amount of surfactant molecules adsorbed on the foam liquid film.
Mass concentration /wt%
Ethanol
n-Propanol
Isobutanol
Tension /mN·m−1
Height /mm
Foam stability coefficient
Tension /mN·m−1
Height /mm
Foam stability coefficient
Tension /mN·m−1
Height /mm
Foam stability coefficient
0.001
22.6
136
0.972
22.3
162
0.969
22.1
183
0.967
0.005
22.5
138
0.971
22.2
163
0.967
21.9
180
0.983
0.01
22.3
134
0.978
22.2
170
0.972
21.9
181
0.978
0.05
22.3
136
0.979
22.0
166
0.967
22.0
184
0.978
0.1
22.4
135
0.973
22.0
167
0.969
21.7
182
0.984
0.5
22.6
132
0.972
22.1
165
0.966
21.8
177
0.972
1
22.6
134
0.972
22.2
167
0.959
21.9
179
0.972
Comparing the addition of different low-carbon alcohols in the two compound systems and observing the change of foam height with alcohol mass fraction, it can be found that the addition of ethanol inhibited the foaming ability of the Silok8141/SDS system, and the foaming height of the ternary system was lower than that of the binary system. With the increase of n-propanol mass fraction, the foaming height of the ternary system decreased at first and then increasing, and finally stabilized at about 166 mm. In contrast, the foaming height of the ternary system with isobutanol was slightly higher than that without alcohol, and stabilized at about 180 mm. As can be seen in Table 5, with the increase of the mass fraction of low carbon alcohol, the foaming height of Silok8008/SDS system regulated by n-propanol and isobutanol decreased, while the ternary foam containing ethanol increased. The addition of low mass fraction of n-propanol and isobutanol promoted the foaming height of the binary system, and with the increase of n-propanol mass fraction, the foaming height was lower than that of the binary system when the mass fraction of n-propanol was greater than 0.05 %, while the foaming height was similar to that of the binary system when the mass fraction of isobutanol was 0.1 %.
Mass concentration /wt%
Ethanol
n-Propanol
Isobutanol
Tension /mN·m−1
Height /mm
Foam stability coefficient
Tension /mN·m−1
Height /mm
Foam stability coefficient
Tension /mN·m−1
Height /mm
Foam stability coefficient
0.001
23.8
48
0.893
23.5
62
0.966
22.7
68
0.967
0.005
23.7
53
0.897
23.4
60
0.957
22.2
66
0.939
0.01
23.5
55
0.947
23.2
60
0.956
22.0
65
0.939
0.05
23.5
60
0.939
23.0
54
0.962
21.6
59
0.955
0.1
23.4
61
0.941
22.5
51
0.959
21.7
58
0.932
0.5
23.5
57
0.947
22.3
45
0.964
21.7
57
0.930
1
23.3
53
0.943
22.4
46
0.939
21.7
54
0.926
Observing the foam stability coefficients in Table 4, it was found that the foam stability coefficients of the ternary system changed less with the increase in the mass fraction of lower carbon alcohol. The addition of isobutanol and ethanol promoted the foam stability coefficient of the Silok8141/SDS system. When the mass fraction of isobutanol was greater than 0.01 %, the foam stability coefficient tended to be stable, with the maximum value reaching 0.984. On the contrary, in Table 5, the addition of low-carbon alcohol obviously inhibits the foam stability of the Silok8008/SDS system, and the foam stability coefficients of the ternary system are smaller than those of the binary system.
3.3 Study on the performance of ternary foams regulated by low-carbon alcohol
In order to further investigate the effect of the addition of low-carbon alcohols on the FFEA, the author added 25 % drainage time and expansion ratio to the experiment on the basis of 3.2. 25 % drainage time (Marinova et al., 2017; Schofield et al., 2021) refers to the time used from the beginning of foam generation to the foam drainage of 25 % of the mass of the liquid, the longer the 25 % drainage time, indicating that the foam is more stable, baked by the fire, the combustion of the fire more easily destroyed. Expansion ratio (Hinnant et al., 2022; Wang et al., 2023) is a measure of the foam foaming ability of the index, a high number of foams can quickly fill a large area of the fire area. The laboratory measured Silok8141/SDS and Silok8008/SDS 25 % drainage time were 91 s and 85 s, the expansion ratio was 10.34 and 5.71.
3.3.1 Determination of expansion ratio and 25 % drainage time
Using the method of determining the expansion ratio and 25 % drainage time in section 1.2, the changes in expansion ratio and 25 % drainage time of different types and mass fraction of ternary system foams were measured as shown in Fig. 8 and Fig. 9. Fig. 8(a) shows that the expansion ratio shows a steady increase with the increase in the mass fraction of alcohols, especially isobutanol shows the most significant increase in expansion ratio at a mass fraction of 0.1 %, with an expansion ratio of 16.46, and in Fig. 8(b), when the ethanol addition is 0.1 %, the maximum expansion ratio is 9.06. However, they all exceeded the binary system. This is mainly due to the fact that the addition of low-carbon alcohol can be effectively adsorbed at the foam gas–liquid interface, which can be effectively interspersed between the active agent molecules and help to enhance the foaming ability of the solution(Bielawska et al., 2013).Expansion ratio of ternary systems.
25 % drainage time of ternary systems.
Fig. 9(a) shows that the 25 % drainage time tends to decrease with the increase of ethanol and n-propanol mass fraction. When the ethanol mass fraction is 0.001 %, the 25 % drainage time of Silok8141/SDS system is 110 s, and with the increase of isobutanol mass fraction, the 25 % drainage time increases, and when the mass fraction is 0.1 %, the maximum value is 108 s. From Fig. 9(b), it can be seen that the trend of 25 % drainage time of Silok8008/SDS system with increasing mass fraction of ethanol and isobutanol is similar to that of Fig. 9(a). When the isobutanol mass fraction is 0.1 % the 25 % drainage time is maximized to 105 s.When the mass fraction of n-propanol was 0.05 %, the 25 % drainage time of the ternary system increased compared to the mass fraction of 0.01 %, and the 25 % drainage time decreased when the mass fraction of n-propanol was greater than 0.05 %.
3.3.2 Analysis of foam drainage percentage and drainage rate
In accordance with the experimental scheme in section 2.2, 25 % drainage time of the ternary system was measured, and the moment of the change of the electronic balance reading was set as the 0 point, i.e., the starting point, and the change curves of percentage of drainage corresponding to different ternary system foams are plotted against time, as shown in Fig. 10 and Fig. 11. In order to further analyze the influence of the addition of low-carbon alcohols on the drainage rate of the foam system within the same drainage time, the curves in Fig. 10 and Fig. 11 were fitted by using the Origin software, and the average slope of each curve was derived as the average liquid solution rate, and the average drainage rate of the foam system under different conditions was plotted, as shown in Fig. 12.Percentage of drainage for Silok8141/SDS/alcohol ternary system.
Percentage of drainage for Silok8008/SDS/alcohol ternary system.
Average drainage rate of ternary system.
Observing Fig. 10 and Fig. 12 (a), it was found that when the addition of ethanol was 0.001 %, the average drainage rate of the ternary system containing Silok8141 was the lowest, which was 0.2424 %·s−1, and the drainage process was inhibited. Beyond this mass fraction, the increase in ethanol addition accelerates the drainage process of the ternary system, and the average drainage rate gradually increases with the increase in ethanol addition. When the n-propanol addition reaches 0.1 %, the average drainage rate reaches a maximum of 0.2911 %·s−1. When the isobutanol addition is 0.001 %, the average drainage rate of the ternary system drainage process exceeds that of the other two types of ternary system foams, reaching 0.2712 %·s−1. And the average drainage rate is also greater than the corresponding characteristic values of the other two types of ternary system foams when the mass fraction of isobutanol is 0.01 % and 0.05 %. This indicates that the introduction of isobutanol at mass fractions of 0.001 %, 0.01 % and 0.05 % into the foam compounding system is detrimental to the foam stability and promotes the foam drainage process. When the isobutanol addition reaches 0.1 %, the average rate is 0.2395 %·s−1, which is a large decrease. Observing Fig. 11 and Fig. 12(b), it can be found that the trend of the average drainage rate of the ternary system containing Silok8008 is similar to that of the ternary system of Silok8141, with a minimum of 0.2212 %·s−1 when n-propanol is added at 0.001 %, and the average drainage rate of the ternary system decreases slowly when isobutanol is added at a level greater than 0.005 %, with a minimum of 0.2279 %·s−1.
4 Fire extinguishing experiments and mechanistic analysis
Based on the research in Chapter 3, two FFEAs with Silok8141/SDS/isobutanol and 8008/SDS/isobutanol were configured. The fire extinguishing ability of the two ternary system FFEAs was evaluated by recording the flame temperature change, flame cooling rate and fire extinguishing time of the two FFEAs to extinguish the oil pool fire. The basic formulations of FFEA are shown in Table 6 and Table 7. APP, Urea, ethylene glycol butyl ether and xanthan gum are used as auxiliaries to provide anti-freeze, flame retardant and enhanced liquid holding capacity.
Reagent
SDS
Silok8141
Isobutanol
APP
Urea
Ethylene glycol butyl ether
Xanthan gum
Water
Percentage /%
0.04
0.1
0.1
0.15
0.3
0.03
0.03
99.05
Reagent
SDS
Silok8141
Isobutanol
APP
Urea
Ethylene glycol butyl ether
Xanthan gum
Water
Percentage /%
0.04
0.1
0.05
0.15
0.3
0.03
0.03
99.05
4.1 Analysis of flame temperature change
Based on the flame temperature collection method in section 2.3, the change in flame temperature at different heights during the application of FFEA is plotted as shown in Fig. 13. Observation of Fig. 13 shows that before the foam extinguishing agent was applied, the flame temperatures at 0 cm, 50 cm and 100 cm above the oil pool increased rapidly, this was due to the continuous generation of combustible gases during the oil burning process, which mixed with the surrounding air and burned. The main height at which the combustion process occurs is around 0 cm above the oil surface, while the combustible vapour content formed at the 50 cm and 100 cm heights decreases and the combustion intensity is correspondingly weaker, while the heat exchange with the environment is enhanced, resulting in the smallest peak flame temperature at 100 cm.Flame temperature variation curve with time during fire extinguishing experiments.
Under the effect of the application and continuous supply of foam extinguishing agent, the flame temperature at 0 cm, 50 cm and 100 cm overall decreases rapidly until the pool fire is extinguished, after which the temperature tends to room temperature. In order to study the flame cooling rate at different heights, the derivatives of different points of the FFEA flame temperature curve in Fig. 13 were solved using the Tangent plug-in of the Origin software, and the maximum flame cooling rates at different heights were obtained, as shown in Table 8.
Flame height
0 mm
50 mm
100 mm
Maximum cooling rate/°C·s−1
8141/SDS/ Isobutanol
15.54
13.57
12.52
8008/SDS/ Isobutanol
15.46
13.15
12.39
Average cooling rate/°C·s−1
8141/SDS/ Isobutanol
7.84
6.05
4.30
8008/SDS/ Isobutanol
6.49
5.36
3.62
From Table 8, it can be seen that the FFEA of Silok8141/SDS/isobutanol system has better cooling effect on the oil pool, and the maximum cooling rate is slightly larger than that of Silok8008/SDS/isobutanol system. The average cooling rate varies greatly due to the difference in fire extinguishing time, and the average cooling rate of FFEA of Silok8141/SDS/isobutanol system is greater than that of Silok8008/SDS/isobutanol system in the same position.
4.2 Thermal imaging of the fire extinguishing process
Multiple time periods of infrared flames and their temperature changes were plotted through the shooting of FFEA extinguishing agent to extinguish the flame process of the oil pool by a handheld infrared thermal imager, as shown in Fig. 14. By observation it can be found that with the release of the two FFEA flame temperatures decreased rapidly. Comparing Fig. 14 (a) and Fig. 14 (b), it can be found that the flame temperature under the action of Silok8008/SDS/isobutanol foam system at 20 s and 40 s of continuous release of FFEA is lower than that of Silok8141/SDS/isobutanol foam system in this time period. This is because the surface tension of the FFEA of Silok8008/SDS/isobutanol system is lower, and the lower surface tension in the pre-foam spraying stage allows more foam to spread rapidly on the oil surface, and the cooling effect is more significant in extinguishing the flame of the oil pool. The flame temperature under the action of the Silok8141/SDS/isobutanol foam system was lower when the FFEA was continuously released for 60 s and the flame was extinguished when the release time reached 74 s, while the Silok8008/SDS/isobutanol foam system extinguished the flame of the oil pool for 89 s. This is because Silok8141/SDS/Isobutanol foam system has more good foam stability, as well as a larger expansion ratio, the same quality of foam premix can spray more foam can better cover the surface of the pool and better foam stability makes the foam is not easy to rupture can be more durable to the pool of flame cooling, isolation and a series of operations. The two FFEA fire extinguishing time meets the national standard demand, that is, the low-foam liquid needs to be completed within 180 s to extinguish the fire, which proves that the hydrocarbon surfactant and organosilicon surfactant prepared by low-carbon alcohol modulation of FFEA has a better cooling effect can be effectively extinguish the oil pool fire of the medium scale.Thermal image of FFEA extinguishing oil fire.
5 Mechanistic analysis
In order to clarify the foam stability principle and fire extinguishing mechanism of FFEA, the mechanism analysis of ternary foam system is carried out.
5.1 Analysis of the mechanism of the foam drainage process
The foam drainage coalescence process was filmed by a programme-controlled camera at 2 s intervals. The ternary system foam drainage process was quantitatively analyzed using CAS software, as shown in the Fig. 15, with PG1, 2, 3 and 4 representing the areas of the four bubbles, respectively. Under the action of gravity and capillary force, the trace liquid in the foam flows in the channel network formed by plateau border and intersections of thin films. From 0 s onwards, due to the different bubble sizes in the foam, the gas in the small bubbles will enter the large bubbles through the liquid film due to the pressure difference between the bubbles, and the average diameter of the bubbles will increase with time. By observing Fig. 15(a) and Fig. 15(b), it can be found that PG4 is a smaller bubble surrounded by three larger bubbles. In Fig. 15(a), the initial area of PG4 is 0.0239 mm2, which disappears completely in the 52 s, and the ratio of area to time is used to represent its defoaming rate, that is, 4.59 × 10-4 mm2/s. Similarly in Fig. 15(b) the initial area of PG4 is 0.0357 mm2 and after 74 s the PG4 disappeared and the defoaming rate can be calculated as 5.07 × 10-4 mm2/s. Comparison of the defoaming rates of the two systems shows that the Silok8008/SDS/Isobutanol system defoamed faster, meaning that more foam was coalescence at the same time, which ultimately led to faster foam break-up. Quantitative observation of the change in foam area between the two formulations demonstrates that the Silok8141/SDS/Isobutanol system has a longer liquid drainage time and better foam stability.Quantitative analysis of foam drainage process.
Fig. 16 shows the adsorption state of the surfactant molecules and the foam drainage path at the plateau border for different additions of isobutanol. Silicone surfactant (Silok8141)/hydrocarbon surfactant (SDS)/alcohol (isobutanol) as the main base agent of FFEA through the air compressor and the role of the foam gun to fully foam, in the process of foam formation, the silicone surfactant molecules and the hydrocarbon surfactant molecules through the physical adsorption of the role of the van der Waals force and gas molecules combined to form a layer of molecular film on the gas–liquid interface. In combination with sections 3.2 and 3.3, it can be seen that the foam drainage rate of FFEA containing isobutanol decreases as the addition of isobutanol increases. When there is no isobutanol added, the foam drainage process at the plateau border is shown in Fig. 16 (a), where the liquid flows freely at the channel and water is only adsorbed by the hydrophilic group of the surfactant, which results in a rapid drainage process. The addition of a small amount of polar liquid disturbs the molecular distribution at the plateau border, which reduces the adsorption capacity of the liquid and does not form a dense structure at the plateau border, resulting in a slight increase in the rate of foam drainage compared to the binary system. Fig. 16 (b) shows the molecular distribution at the plateau border and the drainage pathway after the addition of high concentrations of isobutanol. The water solubility of the alcohol decreases as the number of carbon molecules increases. The experiments found that the addition of isobutanol reduced the rate of foam drainage, slowed down the process of foam drainage and increased foam stability.Schematic diagram of the adsorption state of surfactant molecules and the drainage path at the plateau border.
5.2 Mechanistic analysis of extinguish oil fires
Combined with the FFEA to put out the oil fire experiment and section 5.1 on the foam precipitation polymerization mechanism to make the silicone surfactant (Silok8141)/hydrocarbon surfactant (SDS)/alcohol (ethanol) as the main base agent of FFEA to put out the oil fire mechanism diagram. As shown in Fig. 17, SDS molecules and Silok8141 molecules are combined at the foam gas–liquid interface through physical adsorption, the interaction of hydrocarbon surfactant and silicone surfactant can effectively reduce the surface tension, while the addition of isobutanol blocked the foam drainage channel of the foam to delay the process of foam liquid drainage aggregation and increase the stability of the foam. Lower surface tension and better foam stability allow the foam to spread quickly, increasing the blocking effect on the oil surface, preventing the entry of oxygen into the air while suppressing the concentration of volatile flammable gases and small flammable solid particles. The water in the foam is able to play a role in heat absorption and cooling, the foam's ability to hold liquid is greatly enhanced by the action of low-carbon alcohols and surfactants, and the amount of heat lost in the transfer process to the oil surface is increased, while the water vapour produced mixes with the combustible gas, reducing the concentration of combustible gas and the intensity of combustion.Diagram of the mechanism of foam extinguishing oil fires.
6 Conclusions
To find an effective replacement for the traditional fluoride-containing components of AFFF, a new type of FFEA with alcohol-regulated silicone surface activity and hydrocarbon surfactant compounding was designed, and the following conclusions were drawn from the design route of FFEA, the experimental analysis of extinguishing oil fires, and the mechanistic study of the foam analysis of the polymerisation process.
(1) The low-carbon alcohol-modulated Silok8141/SDS and Silok8008/SDS had lower surface tension, better foaming ability, foam stability coefficient, and could delay the foam 25 % drainage time. The Silok8141/SDS foam formula with 0.1 % isobutanol addition (the surface tension is 21.7 mN·m−1, the foaming height is 182 mm, and the foam stability coefficient is 0.984) compared with the fluorine-containing ternary foam extinguishing agent (the surface tension is 17.3 mN·m−1, the foaming height is 117 mm, and the foam stability coefficient is 0.980), the surface tension needs to be further reduced, but the foaming height has a significant increase, and the foam stability coefficient is slightly better.
(2) By carrying out real fire experiments of large-scale foam fire extinguishing, it is proved that the two FFEAs based on Silok8141/SDS/Isobutyl alcohol and Silok8008/SDS/Isobutyl alcohol have the same good fire extinguishing ability, and they can quickly reduce the flame temperature of large-scale oil pool fire. The fire extinguishing time of the two formulations is 74 s and 89 s respectively, which is much lower than the requirement of GB 15308–2006 to complete the fire extinguishing in 180 s. However, the oxidation, reburning capacity and frost resistance of FFEA are still unknown and need to be supported by more experiments.
(3) By observing the change of foam area and the quantitative analysis of foam rupture rate in the process of foam liquation coalescence, combined with the fire extinguishing mechanism of FFEA. It is found that the addition of isobutanol delays the coalescence process of foam liquation, and its excellent liquation blocking mechanism prolongs the foam rupture time and further improves the foam stability. Comparison of the two silicone formulations shows that the Silok8141/SDS/isobutanol formulation has a slower defoaming rate and better foam stability. When applied to oil fire, the Silok8141/SDS/isobutanol system foam with good stability has a long duration in the fire field, and has a strong sealing effect on the oil surface, which can effectively block the contact between oxygen, flammable volatiles and fire sources, and finally achieve effective cooling and fire extinguishing.
CRediT authorship contribution statement
Hailin Jia: Conceptualization, Methodology, Investigation, Writing – original draft, Funding acquisition. Jinxiang Zeng: Investigation, Writing – original draft. Qian Zou: Investigation. Ligang Zheng: Investigation. Rongkun Pan: Validation.
Acknowledgements
This research work was supported by the General Program of the National Natural Science Foundation of China (52274185), the National Key R&D Program of China (2018YFC0807900), the Program for Innovative Research Team in the University of Ministry of Education of China (IRT_16R22) and Innovative scientific research team of Henan Polytechnic University, China (T2021-4).
Declaration of competing interest
The authors declare that they have no known competing financial interests or personal relationships that could have appeared to influence the work reported in this paper.
References
- Inventories and assessment of pops in the State of Kuwait as a basis for Stockholm convention implementation. Emerg. Contam.. 2021;7:88-98.
- [Google Scholar]
- Synergisms between siloxane-polyoxyethylene and alkyl polyglycoside surfactants in foam stability and pool fire extinction. Colloids Surf. A: Physicochem. Eng. Asp.. 2019;579:123686
- [Google Scholar]
- Impact dynamics on slips: effects of liquid droplet’s surface tension and viscosity. Appl. Surf. Sci.. 2020;506:144689
- [Google Scholar]
- Studies of the micellization of cationic–anionic surfactant systems in water and methanol–water mixed solvents. J. Solution Chem.. 2015;44:2090-2105.
- [Google Scholar]
- Studies of aggregation properties of surfactant with and without polyelectrolyte in water and binary mixture of methanol-water from the surface tension measurements. J. Mol. Liq.. 2020;312:113438
- [Google Scholar]
- Determination of ctab cmc in mixed water+short-chain alcohol solvent by surface tension, conductivity, density and viscosity measurements. Colloids Surf. A: Physicochem. Eng. Asp.. 2013;424:81-88.
- [Google Scholar]
- Biodegradability of fluorinated fire-fighting foams in water. Chemosphere. 2015;131:104-109.
- [Google Scholar]
- Current applications of foams formed from mixed surfactant–polymer solutions. Adv. Colloid Interface Sci.. 2015;222:670-677.
- [Google Scholar]
- Interactions in developmental toxicology: concurrent exposure to perfluorooctane sulfonate (pfos) and stress in pregnant mice. Toxicol. Lett.. 2006;164:81-89.
- [Google Scholar]
- Competitive adsorption of surfactants at air/water interfaces. J. Colloid Interface Sci.. 2003;261:233-237.
- [Google Scholar]
- Hetzer RH, Kummerlen F, Wirz K, Blunk D, 2014. Fire testing a new fluorine-free afff based on a novel class of environmentally sound high performance siloxane surfactants, Canterbury, New zealand, International Association for Fire Safety Science, pp. 1261-1270.
- Fire testing a new fluorine-free afff based on a novel class of environmentally sound high performance siloxane surfactants. Fire Saf. Sci.. 2014;11:1261-1270.
- [Google Scholar]
- Exploring synergistic fire suppression of siloxane-glycoside firefighting foam using sulfonated hydrotrope additives to alter surfactant aggregation in solution. Colloids Surf. A: Physicochem. Eng. Asp.. 2022;655:130219
- [Google Scholar]
- Analysis of ternary foam of hydrocarbon/silicone/low carbon alcohol and the inhibition effect on coal spontaneous combustion. Ciesc J.. 2022;73:470-479.
- [Google Scholar]
- Foam performance analysis of fluorine-free foam modified by low carbon alcohol and experimental study on extinguishing oil pool fire. Ciesc J.. 2022;73:4235-4244.
- [Google Scholar]
- Foam performance analysis of fluorine-free foam modified by low carbon alcohol and experimental study on extinguishing oil pool fire. CIESC J.. 2022;73(09):4235-4244.
- [Google Scholar]
- Equilibrium and dynamic surface tension properties of salt-free catanionic surfactants with different hydrocarbon chain lengths. J. Mol. Liq.. 2015;204:126-131.
- [Google Scholar]
- Experimental study on fire-extinguishing efficiency of protein foam in diesel pool fire. Case Stud. Therm. Eng.. 2019;16:100557
- [Google Scholar]
- Effect of polysaccharide polymers on the surface and foam properties of aqueous film-forming foam. Colloid Interface Sci. Commun.. 2021;45:100540
- [Google Scholar]
- Effects of unsafe workplace practices on the fire safety performance of ready-made garments (rmg) buildings. Saf. Sci.. 2021;144:105470
- [Google Scholar]
- Synergistic and antagonistic effects in micellization of mixed surfactants. J. Mol. Liq.. 2022;368:120678
- [Google Scholar]
- Cryo-tem and rheological study on shear-thickening wormlike micelles of zwitterionic/anionic (ahsb/sds) surfactants. J. Colloid Interface Sci.. 2022;608:513-524.
- [Google Scholar]
- On the role of surface charge and surface tension tuned by surfactant in stabilizing bulk nanobubbles. Appl. Surf. Sci.. 2023;608:155232
- [Google Scholar]
- New surfactant mixtures for fine foams with slowed drainage. Colloids Surf. A: Physicochem. Eng. Asp.. 2017;523:54-61.
- [Google Scholar]
- Establishment of aqueous film forming foam extinguishing agent minimum supply intensity model based on experimental method. J. Loss Prev. Process Ind.. 2020;63:103997
- [Google Scholar]
- One-step synthesis, wettability and foaming properties of high-performance non-ionic hydro-fluorocarbon hybrid surfactants. Appl. Surf. Sci.. 2018;433:264-270.
- [Google Scholar]
- Effect of temperature on stability and film thinning behavior of aqueous film forming foam. J. Mol. Liq.. 2023;370:120978
- [Google Scholar]
- Surface modification to produce hydrophobic nano-silica particles using sodium dodecyl sulfate as a modifier. Appl. Surf. Sci.. 2016;364:103-109.
- [Google Scholar]
- Fluorosurfactant retention in the foam blanket during gravitational drainage of an aqueous film-forming foam. Colloid Interface Sci. Commun.. 2021;42:100404
- [Google Scholar]
- Sheng Y, Jiang N, Lu S, Li C, 2018. Fluorinated and fluorine-free firefighting foams spread on heptane surface. Colloids and Surfaces a: Physicochemical and Engineering Aspects 552: 1-8.
- Role of nanoparticles in the performance of foam stabilized by a mixture of hydrocarbon and fluorocarbon surfactants. Chem. Eng. Sci.. 2020;228:115977
- [Google Scholar]
- Sheng Y., 2018. Inverstigation on fire-fighting foam based on mixture of hydrocarbon and silicone surfactants, University of Science and Technology of China.
- Combined effects of nanoparticles and surfactants upon foam stability. Chem. Eng. Sci.. 2021;238:116601
- [Google Scholar]
- The wettability of poly(tetrafluoroethylene) by aqueous solutions of ternary surfactant mixtures. Appl. Surf. Sci.. 2010;256:7478-7483.
- [Google Scholar]
- Properties of some nonionic fluorocarbon surfactants and their mixtures with hydrocarbon ones. Adv. Colloid Interface Sci.. 2021;292:102421
- [Google Scholar]
- Effect of fluorocarbon surfactants on the adsorption of hydrocarbon surfactants mixture at the water-air interface. J. Mol. Liq.. 2022;345:117832
- [Google Scholar]
- Assessment of the per- and polyfluoroalkyl substances analysis under the stockholm convention – 2018/2019. Chemosphere. 2023;313:137549
- [Google Scholar]
- Application of green surfactants developing environment friendly foam extinguishing agent. Fire Technol.. 2015;51:503-511.
- [Google Scholar]
- High-expansion-ratio plla/pdla/hnt composite foams with good thermally insulating property and enhanced compression performance via supercritical co2. Int. J. Biol. Macromol.. 2023;236:123961
- [Google Scholar]
- Effects of different anionic surfactants on methane hydrate formation. Chem. Eng. Sci.. 2015;137:896-903.
- [Google Scholar]
- Preparation of new gel foam and evaluation of its fire extinguishing performance. Colloids Surf. A: Physicochem. Eng. Asp.. 2021;629:127443
- [Google Scholar]
- Fire-extinguishing performance and mechanism of aqueous film-forming foam in diesel pool fire. Case Stud. Therm. Eng.. 2020;17:100578
- [Google Scholar]
- Evaluation of interfacial, micellar, and foaming properties of the solutions comprising fluorocarbon surfactant, cocamidopropyl betaine, and gleditsia saponin as fire-extinguishing agents. Chem. Eng. Sci.. 2023;272:118590
- [Google Scholar]
- Comparative studies on foam stability, oil-film interaction and fire extinguishing performance for fluorine-free and fluorinated foams. Process Saf. Environ. Prot.. 2020;133:201-215.
- [Google Scholar]
- Influence of seawater on interfacial properties, foam performance and aggregation behaviour of fluorocarbon/hydrocarbon surfactant mixtures. J. Mol. Liq.. 2022;359:119297
- [Google Scholar]
- Stability and thinning behaviour of aqueous foam films containing fluorocarbon and hydrocarbon surfactant mixtures. J. Mol. Liq.. 2022;359:119225
- [Google Scholar]
- Modification of adsorption, aggregation and wetting properties of surfactants by short chain alcohols. Adv. Colloid Interface Sci.. 2020;284:102249
- [Google Scholar]
- Role of silicone surfactant in flexible polyurethane foam. J. Colloid Interface Sci.. 1999;215:270-279.
- [Google Scholar]
- Adjustable surface activity and wetting ability of anionic hydrocarbon and nonionic short-chain fluorocarbon surfactant mixtures: effects of li+ and mg2+. J. Mol. Liq.. 2022;350:118538
- [Google Scholar]
- Self-assembly and viscosity changes of binary surfactant solutions: a molecular dynamics study. J. Colloid Interface Sci.. 2021;585:250-257.
- [Google Scholar]