Translate this page into:
Characterization of polycyclic aromatic hydrocarbon from open burning of disposable COVID-19 facemask: Spatial distribution and risk assessment
⁎Corresponding author. adesinaolusola50@yahoo.com (Olusola Adedayo Adesina) adesinao@abuad.edu.ng (Olusola Adedayo Adesina)
-
Received: ,
Accepted: ,
This article was originally published by Elsevier and was migrated to Scientific Scholar after the change of Publisher.
Abstract
The use of facemasks as a preventive measure against infection of the COVID-19 virus has some associated environmental impacts, especially in Subs-Saharan African countries where the used facemasks are being disposed of using open burning. Hence this study characterized Polycyclic Aromatic Hydrocarbons (PAHs) emissions from different COVID-19 facemask materials and determined the associated risk of open burning of facemask. Facemasks of different materials were sourced from open dump sites. Each material was combusted in an open reactor and the emissions were collected using A filter-sorbent sampling system. Analysis of PAHs in the sample was carried out using GCMS. The associated health risk was assessed using Incremental Life Cancer Risk (ILCR) and Hazard Quotient (HQ). The result showed the concentration of PAHs emission ranged ∑ PAHs 73.33 –368.16 µg/m3 while carcinogenic PAHs ranged ∑ CarPAHs 26.79–179.74 µg/m3. The result also showed the surgical and N95 facemask emits the highest concentration of PAHs. The modeled annual ground-level PAHs Isopleth reveals the presence of a high concentration of PAHs around the vicinity of the burning site after a year. Proper disposal of these facemask using controlled medical incinerator is highly recommended.
Keywords
COVID-19
Emission
Facemask
PAHs
Risk
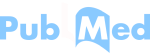
1 Introduction
The outbreak of coronavirus (COVID-19) has affected the world economy and the way of life. Unfortunately, this disease is going to be around for a long time. There are 609,848,852 confirmed cases of COVID-19 infections globally and 6,507,002 deaths have been recorded globally (WHO, 2022). Although a lower death rate from this virus is recorded in Africa compared to other continents, about 9,318,590 were recorded in this continent (WHO, 2022). After the lockdown in the year 2020, various measures are being put in place to curtail the spread of the virus, which include, frequent washing of hands, social distancing, travel restriction, isolation, avoiding crowded spaces, and as well as the mass wearing of face masks (Lin et al., 2020; Chintalapudi et al., 2020).
A facemask decreases the excretion of respiratory droplets from infected individuals and helps reduce the spread of infections (ECDC, 2020). It also reduces the number of times a person touches the face/mouth/nose with unwashed hands, which can significantly reduce the chance of infection (Fadare and Okoffo, 2020). Research has also shown the effectiveness of face masks in preventing the virus from entering the respiratory system (Elachola et al., 2020; Barasheed et al., 2016). The use of face masks as one of the precautionary measures has led to a global shortage of these disposable facemasks (Wu et al., 2020). Between 2019 and 2021 about 2.5 billion N95 face mask was produced (statistica.com) and The United Nations has forecasted the demand for surgical masks alone to be in the region of 2.4 billion (unicef.org). Due to the high demand for the COVID-19 disposable facemask, the government in the developing countries of Africa such as Nigeria is encouraging the production of large quantities of locally made facemask produced from local fabrics and materials.
However, the use of facemasks has created environmental challenges, especially in the developing world with poor waste management policies. These masks are dumped indiscriminately in the environment which also blocks the water channels, and some of these masks find their way to the open incineration site where they are being combusted openly. Also, most countries of Sub-Saharan Africa practice open burning in disposing of their wastes where used masks from hospitals and public places are combusted in open incineration sites (Awodele and Adewoye, 2016; Chisholm et al., 2021). However burning wastes such as facemasks is associated with the emission of Polycyclic Aromatic Hydrocarbon (PAHs) (Mugica-Alvarez et al., 2015; Abdel-Shafy and Mansour, 2016; Kalisa et al., 2018; Adesina et al., 2020).
PAHs are products of incomplete combustion formed as a result of the fusion of two or more Benzene rings and they are carcinogenic, teratogenic, and mutagenic (Pongpiachan et al., 2015; Wang et al., 2015; Patel et al., 2020). There are also non-carcinogenic risks associated with exposure to PAHs to human which can be long-term health effects such as liver damage, and cardiopulmonary death (Zhao et al., 2023). PAHs can persist in the environment and can also accumulate in vascular plants and have health effects along the food chain (Zha et al., 2018).
There had been studies on open incineration of waste (Adesina et al., 2020; Cheng et al., 2022) but no focus of disposable COVID-19 facemask. However, studies on combustion of facemask in which levels of Particulate Matter was the focus (Pastukhov et al., 2023; Szefer et al., 2021).
However none of these studies focused on the characterization of PAHs from combustion of different COVID-19 disposable facemasks either from incinerator or open burning. Hence this study characterized PAHs from the open burning of different types of disposable COVID-19 facemask and the risk associated with inhalation of PAHs from the open burning of facemask. The study also modeled the ground concentrations of PAHs around the point source emission of PAHs from the burning of PAHs.
2 Materials and methods
Different facemasks of different materials were sourced at the incineration site of Afe-Babalola University Multi-system Hospital. Safety precaution was taken to avoid infection of the virus during sampling. A total number of 10 different COVID-19 facemasks made from different materials were used for the study. This Includes Surgical, Cotton, Scarf, Plastic shield, and N95 Nose masks. Others are Linen, Spandex, and Organiza nose masks.
2.1 Emission sampling
50g of each facemask material was put in an open reactor of 35cm in diameter and 25cm height. The materials were combusted and emission from each materials was taken. Particulate plus vapor-phase PAHs were collected using A filter-sorbent sampling system of European standard stationary source emission and determination of concentration method (EN- 1948 –SS) with Polyurethane Foam (PUF) (4cm diameter, 5cm thick) as an adsorbent material. The samples were collected isokinetically withdrawn from the gas stream and collected into the train of a sample probe, a filter paper, and a packed column consisting of absorbent material (Hoyos et al., 2008; Adesina et al., 2017). The filter paper and PUF were wrapped in aluminum foil and taken to the laboratory for analysis.
2.2 Extraction and samples analysis
PAHs in PUF and filter paper were extracted into Dichloromethane (Sigma–Aldrich) using a Soxhlet extractor placed on the heating mantle, the temperature was set to 60 °C and extraction was done for 7 hr. The extracts were then subjected to a Clean-up procedure using conventional liquid–solid adsorption chromatograph in open glass columns at atmospheric pressure. The column was prepared by adding about 10 to 15-mm plug of glass wool to a chromatograph column and alumina and silica (200–400 mesh, Zico-Tech) were added to the column in the ratio (1:2). The column packing was slightly deactivated using methanol- DCM solution (1:3) for better recovery. The sample extract was decanted into the column and eluted with 40mL 1:1, DCM: n-Hexane (Sigma–Aldrich) (Adesina et al., 2017). The extracted samples were then concentrated using a rotary evaporator (Infitex Co. Ltd) which was later analyzed for PAHs.
2.3 PAHs quantification
The analysis of PAHs was done using A gas chromatograph (Agilent Model 7890A) coupled to a mass selective detector (Varian 3800/4000 GC–MS) (Agilent Technologies, Pato Alto, CA) with column size (60 m x 0.32 mm) using selected ion monitoring mode with Nitrogen as the carrier gas. The GC–MS was calibrated by standard solutions containing all 16 target compounds at different concentrations. 2.0 µL of sample extract was injected into the gas chromatograph using the splitless mode at a temperature of 250 OC. The initial column temperature was 70 OC, at 10 OC min−1 to 260 OC. It was increased to 300 OC min−1 and for 8 min. The solvent delay was 7 min, dwell time of 0.1 s was used for each m/z value. The MS transfer line was maintained at 250 OC, and quantification was based on calibration with the standard analyte using the mass spectrometer in selective ion monitoring (SIM) mode. The obtained concentrations of the different PAH constituents were subjected to source identification and diagnosis using the appropriate mathematical ratios. Quantitative analysis was performed using calibration data from the PAH standard, which contained the sixteen PAHs specified by the EPA.
2.4 Quality assurance/ quality control (QA/QC)
Field and laboratory blanks were analyzed along with the samples, and the determination of instrument detection limits (IDLs) and method detection limits (MDLs) followed (Norlock et al., 2002) procedure. Concentrations of PAHs in the field blanks were below the detection limit for all targeted compounds and no blank correction was carried out. Prior to extraction, 20 ng of Phenanthrene d10 recovery standard (RS) was used to spike the sample and the recovery range of the PAH was between 80% and 90%.
2.5 Health implications
2.5.1 Toxic equivalent
The Toxicity equivalent which is the toxicity potential of individual PAHs is calculated by multiplying PAHs concentration with the toxicity equivalence factor (TEF)(eq. i) (Van den Berg et al., 2006). Where
is the individual concentrations of PAHs
2.5.2 Incremental lifetime cancer risk and Hazard Quotient
Incremental Lifetime Cancer Risk (ILCR) from human contact by inhalation of emission from the exhaust of the generator is calculated using eq. ii. Also, the non-carcinogenic risk, Hazard Quotient (HQ) is calculated using eq, iii
2.6 Spatial distribution
Environmental Protection Agency Regulatory Model (ISCST3 AERMOD) software was used to predict the spatial distribution of PAHs from the burning of different face masks. The concentration of PAHs at different distances from open burning sources was predicted using the software. Data inputted were Source PAHs concentration, Wind direction, Emission temperature, and Emission height. A uniform Cartesian grid was used in this study, and a point source emission type was selected at the source pathway (Adepoju et al., 2018). The PAHs emissions dispersion outputs were carried out for 1 hr, 8hrs, 24-hr and 1 year averaging period for all facemasks materials.
3 Result and discussion
In this study 16 PAHs were analyzed: naphthalene (Naph), acenaphthalene (Acy), acenaphthene (Ace), fluorene (Fln), phenanthrene (Phe), anthracene (Ant), fluoranthene (Flt), pyrene (Pyr), benz[a]anthracene (BaA), chrysene (CHR), benzo[b]fluoranthene (BbF), benzo[k]fluoranthene (BkF), benzo[a]pyrene (BaP), indeno[1,2,3-cd]pyrene (IcP), dibenz[a,h]anthracene (DhA), benzo[ghi]perylene (BgP). Table 1 shows the concentration of PAHs at different sampling locations. The surgical nose mask has the highest sum of PAHs concentration of 368.16 ± 39.14 µg/m3 with Pyr as the most dominant having concentration of 69.75 µg/m3. Other materials with high concentrations of PAHs are cotton and N95 face masks with ∑ PAHs of 353.31 ± 51.6 µg/m3 and 352.89 ± 34.91 µg/m3, respectively. However, facemask materials with the lowest ∑ PAHs are spandex and organza material with concentrations of 73.33 ± 14.3 µg/m3 and 72.86 ± 17.88 µg/m3, respectively. The only concentration of PAHs observed from the emission of two materials is close to the observation of studies by Cheng et al. (2022) and Adesina et al. (2020) which was done on the characterization of PAHs from open burning Municipal Solid Waste (MSW). The concentrations of PAHs emission from otherfacemask materials are way higher than the results from these studies. This implies combustion of facemasks especially N95, Surgical, and cotton facemasks will elevate the concentration of PAHs from the open burning of MSW. It is also observed that open burning of locally made facemasks emits fewer PAHs compared with conventional surgical and N95 facemasks, this could be a result of the simple structure of the composition of locally made facemasks.
μg/m3
surgical
Std
cotton
Std
Towel
Std
scarf
Std
Face Shield
Std
N95
Std
linen
Std
Spandex
Std
Organza
Std
Nap
28.23
3.2
13.22
2.3
5.65
0.6
4.35
0.8
5.3
0.21
27.00
2.2
0.11
0.3
0.11
0.02
0.11
0.01
Acy
1.80
0.6
27.7
5.3
0.4
0.1
6.43
0.9
0.04
0.01
0.95
0.2
5.05
0.3
4.95
0.3
5.12
1.2
Acen
40.35
3.7
21.41
4.6
9.72
0.7
6.96
1.2
8.9
0.21
39.47
4.6
5.97
1.7
4.70
0.3
4.52
1.6
Fln
11.02
1.3
47.88
6.2
4.17
0.3
8.23
1.3
4.2
0.23
10.35
2.3
9.96
3.2
9.22
1.5
9.47
2.1
Phe
8.27
0.8
62.37
8.9
3.89
0.1
3.99
0.6
3.99
0.13
8.27
2.1
12.3
3.1
12.08
2.1
12.4
2.3
Ant
7.35
0.6
15.12
1.8
3.39
0.6
7.70
1.3
3.82
1.1
8.30
3.2
3.75
1.3
3.75
1.2
3.6
1.3
Flt
12.86
1.2
35.27
3.1
3.89
0.2
7.00
1.2
4.17
1.3
12.44
2.2
7.84
1.2
7.95
2.1
8.02
2.3
Pyr
69.75
5.7
8.20
2.1
14.63
0.6
6.89
0.9
13.75
1.23
70.67
4.8
2.61
0.3
2.72
1.1
2.79
1.3
BaA
67.92
8.2
33.36
3.1
14.28
2.1
0.88
0.2
14.24
2.4
68.59
0.3
7.67
1.32
7.60
1.32
7.56
2.1
CHR
67.00
8.9
8.83
1.3
13.43
2.3
3.39
1.2
13.22
2.6
67.53
5.5
3.53
1.5
3.46
1.4
3.39
1.2
Bbf
35.23
3.2
17.63
3.4
7.21
0.6
7.17
1.2
7.42
0.23
20.74
3.2
4.06
1.2
4.45
0.12
3.89
1.21
Bfk
0.92
0.5
5.65
0.5
0.04
0.03
7.07
2.2
0.04
0.1
1.02
0.4
0.53
0.2
0.67
0.3
0.57
0.01
Bap
2.76
0.4
30.21
4.5
0.11
0.09
4.20
1.3
0.11
0.1
2.01
0.1
7.39
1.21
7.28
1.21
7.17
0.01
Icp
3.67
0.5
20.14
3.7
0.14
0.08
3.82
0.8
0.14
0.12
3.11
0.3
3.89
1.4
3.82
1.2
3.82
1.2
DHa
1.84
0.3
5.05
0.6
0.07
0.01
3.85
1.3
0.07
0.21
2.05
1.1
0.39
0.01
0.53
0.1
0.39
0.01
Bgp
9.19
0.3
1.27
0.2
0.14
0.06
5.16
1.3
3.46
1.2
10.39
2.4
0.04
0.3
0.04
0.01
0.04
0.02
∑ PAHs
368.2
39.4
353.31
51.6
81.2
8.5
87.1
17.7
82.87
11.4
352.9
34.9
75.09
18.6
73.33
14.3
72.86
17.9
∑ CarPAHs
179.34
22
120.87
17.1
35.28
5.21
30.38
8.2
35.24
5.76
165.05
10.9
27.46
6.84
27.81
5.65
26.79
5.74
The United States Environmental Protection Agency has identified 7 compounds of PAHs to be carcinogenic (Benzo[a]anthracene, Chrysene, Benzo[b]fluoranthene Benzo[k]fluoranthene, Benzo[a]pyrene, indeno [1, 2, 3-cd] pyrene and Dibenzo (a,h) anthracene). Fig. 1 shows the sum of carcinogenic PAHs while Fig. 2 shows the distribution of carcinogenic PAHs. Among all carcinogenic PAHs Benzo[a]pyrene is usually used as an indication of PAHs toxicity, the result reveals cotton facemask emitted the highest concentration of Benzo[a]pyrene of 30.21± 4.50µg/m3. Surgical and N95 facemasks have the highest concentration of ∑ carcinogenic PAHs of 179.34 ± 22.00 µg/m3 and 165.05 ± 10.90 µg/m3, respectively. This is worrisome as these facemasks are the ones recommended by WHO as most effective in the prevention of COVID-19. Benzo[a]anthracene and Chrysene are the most dominant carcinogenic PAHs in all the materials studied this may be connected with the combustion temperature which favors the emission of these compounds. Open burning usually takes place at a lower temperature than incineration (Zhang et al., 2011).Sum of carcinogenic PAHs concentration.
Distribution of Carcinogenic PAHs.
The distribution of PAHs based on the number of rings is shown in Fig. 3. 2-ring PAHs (Nap), 3-ring PAHs (Ace, Acy, Flu, Phen, and Anth), 4-ring PAHs (Flt, Pyr, BaA, and Chr), 5-ring PAHs (BbF, BkF, BaP, and DahA), and 6-ring PAHs (BghiP and Ind) (Hassanvand et al., 2015; Rostami et al., 2019; Adesina et al., 2021). 2-ring PAH compounds are the least dominant in all the materials contributing between 2 and –8%. This implies the PAH emission from the burning of used facemasks is mostly in the particulate phase. while 6-ring PAHs contribute between 1 and 9 %. 3-ring, 4-ring, and 5-ring PAHs are in high concentration this could only be linked to the low temperature at open burning occurs (Prakash and Singh, 2011; Zhang et al., 2011). PAHs are also classified as Low molecular weight (LMW), 4 ring PAHs are middle molecular weight (MMW) while high molecular weight (HMW) consist of 5 and 6-ring PAHs (Chen et al., 2017; Rostami et al., 2019; Adesina et al., 2021). Fig. 4 shows the distribution of PAHs based on molecular weight. Generally, MMW and HMW PAHs are in the highest concentration due to temperature and airflow during the burning of these materials. It is however of great concern because MMW and HMW PAHs are the most hazardous.Distribution Based on PAHs ring.
Distribution of PAHs based on Molecular Weight.
3.1 Risk assessment
Toxicity equivalent (TEQ) is used to assess the toxicity of the individual PAHs (Nisbet and Lagoy, 1992). TEQs of PAHs emitted from open burning of facemasks are shown in Table 2. Bap has the highest toxicity with the range of 0.11–7.39 µg/m3. Other compounds with high toxicity are BaA and CHR with the range of 0.09–6.86 and 0.34–6.80 µg/m3. The total toxicity equivalent TTEQ is the summation of all TEQs of various PAH compounds. The result showed surgical face mask has a TTEQ of 25.45 µg/m3. Other materials with high TTEQ are N95 and cotton face masks with values of 23.72 and 20.62 µg/m3, respectively. This implies that emissions from open burning of surgical, N95, and cotton facemasks have a higher tendency to cause harm than other locally made facemasks.
surgical
cotton
Towel
scarf
Face Shield
N95
linen
Spandex
Organza
Nap
0.282
0.132
0.057
0.044
0.053
0.270
0.001
0.001
0.001
Acy
0.018
0.277
0.004
0.064
0.000
0.010
0.051
0.050
0.051
Acen
0.404
0.214
0.097
0.070
0.089
0.395
0.060
0.047
0.045
Fln
0.110
0.479
0.042
0.082
0.042
0.104
0.100
0.092
0.095
Phe
0.083
0.624
0.389
0.040
0.040
0.083
0.123
0.121
0.124
Ant
0.735
1.512
0.339
0.770
0.382
0.830
0.375
0.375
0.360
Flt
0.129
0.353
0.039
0.070
0.042
0.124
0.078
0.080
0.080
Pyr
0.698
0.082
0.146
0.069
0.138
0.707
0.026
0.027
0.028
BaA
6.792
3.336
1.428
0.088
1.424
6.859
0.767
0.760
0.756
CHR
6.700
0.883
1.343
0.339
1.322
6.753
0.353
0.346
0.339
Bbf
3.523
1.763
0.721
0.717
0.742
2.074
0.406
0.445
0.389
Bfk
0.092
0.565
0.004
0.707
0.004
0.102
0.053
0.067
0.057
Bap
2.760
3.210
0.110
4.200
0.110
2.010
7.390
7.280
7.170
Icp
0.367
2.014
0.014
0.382
0.014
0.311
0.389
0.382
0.382
DHa
1.840
5.050
0.070
3.850
0.070
2.050
0.390
0.530
0.390
Bgp
0.919
0.127
0.014
0.516
0.346
1.039
0.004
0.004
0.004
TTEQ
25.451
20.621
4.817
12.008
4.818
23.720
10.565
10.606
10.271
The incremental lifetime cancer risk (ILCR) from inhalation of PAHs emitted from open burning of facemask was calculated using adult and children USEPA cancer Unit Risk factor of (6
. This implies 6 cancer cases per 10,000 people with chronic inhalational exposure to BaPeq (1 ng/m3) within their lifetime of 70 years (Rostami et al., 2019). The calculated ILCR and HQ are shown in Table 3. The calculated ILCR based on the USEPA cancer Unit Risk factor range
for towel and N95 face mask, respectively for adults, while ILCR calculated for children range
for face shield and surgical face mask, respectively. Using the WHO cancer risk factor, the ILCR values for adults range from
for towel and surgical facemasks, respectively while ILCR for children
for towel and surgical facemasks, respectively. It is however worrisome that the values of ILCR obtained are all higher than the permissible limit of
and
, respectively for WHO and USEPA. Also, the calculated HQ range was 4.40–22.19 for Adults while the 4.70–24.86 was obtained for children. The high HQ implies high non-carcinogenic risk is associated with inhalation of emission of PAHs from open burning of facemasks.
Surgical
cotton
Towel
scarf
Face Shield
N95
linen
Spandex
Organza
ILCR Adult
ILCR Children
HQ Adult
22.19
17.99
4.20
10.47
4.20
20.69
9.22
9.25
8.96
HQ Children
24.86
20.14
4.70
11.73
4.70
23.17
10.32
10.36
10.03
3.2 Spatial distribution
Fig. 5 (a) shows the dispersion model of PAHs from the open burning of the facemask. The color ranges in legends show the distribution of PAH concentrations around the vicinity of the open burning of the facemask. The region with pink coloration revealed the presence of a high concentration of PAHs while the region with purple implies the lowest PAHs concentration. Generally, the effects of the emission of the burning are noticed within 600m of the eastern direction of the site. Within 1 h of emission (Fig. 5a) of PAHs from the burning of the masks PAHs highest concentration of PAHs is noticed around the northeastern direction from the burning source, this could only be linked to the prevalent wind direction around the burning site. At 8 hrs and 24 hrs (5b and c), a decrease in concentration within 100m from the burning site was observed due to the diffusion and dilution by the wind. Fig. 5d shows the Isopleth Annual Ground Level PAHs. It is observed that there is still the presence of an appreciable concentration of PAHs around the vicinity of the burning site even after a year, this is linked to the persistent nature of PAHs.Dispersion model of pahs from the open burning of facemask.
4 Conclusion
The study characterizes PAHs from open burning of different COVID-19 facemask materials and also calculated the risk associated with inhalation of PAHs emission using some health indexes. The result showed the concentration of PAHs emission ranged ∑ PAHs 73.33–368.16 µg/m3 while carcinogenic PAHs ranged ∑ CarPAHs 26.79–179.74 µg/m3. The result also showed the surgical and N95 facemask emits the highest concentration of PAHs. Proper disposal of these facemask using controlled medical incinerator is highly recommended.The value of ILCRs calculated is higher than the permissible limit of and , respectively for WHO and USEPA. The calculated HQ values are all greater 1, which implies associated high non-carcinogenic risk. The annual ground-level PAHs Isopleth reveals the presence of a high concentration of PAHs around the vicinity of the burning site after a year. Proper disposal of these facemask using controlled medical incinerator is highly recommended.
CRediT authorship contribution statement
Olusola Adedayo Adesina: Conceptualization, Methodology, Software, Data curation, Writing – original draft preparation, Writing – review & editing. Raymond Opara: onceptualization, Methodology, Software, Data curation, Writing – original draft preparation, Supervision. Abiodun John Adewale: Visualization, Investigation. Mayowa Adeoye Lala: Visualization, Investigation. Jacob Ademola Sonibare: Software, Validation, Writing – review & editing.
Acknowledgment
The author thanks the technical staff of the department of Chemical and Petroleum Engineering. Afe-Babalola University Ado-Ekiti.
Declaration of competing interest
The authors declare that they have no known competing financial interests or personal relationships that could have appeared to influence the work reported in this paper.
References
- A review on polycyclic aromatic hydrocarbons: source, environmental impact, effect on human health and remediation. Egypt. J. Pet.. 2016;25(1):107-123.
- [Google Scholar]
- Estimation of gaseous criteria air pollutants from road transport system in Lagos metropolis of Nigeria. Environ. Qual. Manag.. 2018;1–8
- [CrossRef] [Google Scholar]
- Spatiotemporal distributions of polycyclic aromatic hydrocarbons close to a typical medical waste incinerator. Environ. Sci. Pollut. Res.. 2017;25:274-282.
- [Google Scholar]
- Level of polycyclic aromatic hydrocarbon emission in the ambient air and residual ash from a typical municipal solid waste open burning site in Nigeria. Waste Dispos. Sustain. Energy. 2020;2:105-111.
- [CrossRef] [Google Scholar]
- Indoor levels of polycyclic aromatic hydrocarbons (PAHs) from environment tobacco smoke of public bars. Ecotoxicol. Environ. Saf.. 2021;208:111604
- [Google Scholar]
- Oparah AC assessment of medical waste management in seven hospitals in Lagos, Nigeria. BMC Public Health. 2016;16:1-11.
- [Google Scholar]
- Uptake and effectiveness of facemask against respiratory infections at mass gatherings: a systematic review. Int. J. Infect. Dis.. 2016;47:105-111.
- [Google Scholar]
- PM2.5-bound PAHs in three indoor and one outdoor air in Beijing: concentration, source and health risk assessment. Sci. Total Environ.. 2017;586:255-264.
- [CrossRef] [Google Scholar]
- Characterization and Risk Assessment of Airborne Polycyclic Aromatic Hydrocarbons From Open Burning of Municipal Solid Waste. Front. Environ. Sci.. 2022;10:861770.
- [CrossRef] [Google Scholar]
- COVID-19 virus outbreak forecasting of registered and recovered cases after a sixty-day lockdown in Italy: a data driven model approach. J. Microbiol. Immunol. Infect.. 2020;53:396-403.
- [Google Scholar]
- Sustainable waste management of medical waste in African developing countries: a narrative review. Waste Manag. Res.. 2021;39(9):1149-1163.
- [CrossRef] [Google Scholar]
- ECDC, 2020. Using Face Masks in the Community. European Centre for Disease Prevention and Control, pp. 1–6 (April).
- Elachola, H., Ebrahim, S.H., Gozzer, E., 2020. COVID-19_Facemask use prevalence in international airports in Asia, Europe and the Americas, March 2020. Travel Med. Infect. Dis., 101637.
- COVID-19 face masks: a potential source of microplastic fibers in the environment. Sci. Total Environ.. 2020;737:140279
- [Google Scholar]
- Characterization of PAHs and metals in indoor/outdoor PM10/PM2. 5/PM1 in a retirement home and a schooldormitory. Sci. Total Environ.. 2015;527:100-110.
- [Google Scholar]
- Total suspended particulate (TSP), polychlorinated dibenzodioxin (PCDD) and polychlorinated dibenzofuran (PCDF) emissions from medical waste incinerators in Antioquia, Colombia. Chemosphere. 2008;73:137-142.
- [Google Scholar]
- Characterization and risk assessment of atmospheric PM2. 5 and PM10 particulate-bound PAHs and NPAHs in Rwanda, Central-East Africa. Environ. Sci. Tech.. 2018;52(21):12179-12187.
- [Google Scholar]
- Google searches for the keywords of “wash hands” predict the speed of national spread of COVID-19 outbreak among 21 countries. Brain Behav. Immun. 2020
- [Google Scholar]
- Emissions of PAHs derived from sugarcane burning and processing in Chiapas and Morelos México. Sci. Total Environ.. 2015;527:474-482.
- [Google Scholar]
- Toxic equivalency factors (TEFs) for polycyclic aromatic hydrocarbons (PAHs) Regul. Toxicol. Pharm.. 1992;16(3):290-300.
- [Google Scholar]
- Large volume injection PTV-GC-MS analysis of polycyclic aromatic hydrocarbons in air and sediment samples. J. Air Waste Manag. Assoc.. 2002;52:19-26.
- [Google Scholar]
- Disposable facemask waste combustion emits neuroactive smoke particulate matter. Sci. Rep.. 2023;13:17771.
- [CrossRef] [Google Scholar]
- Polycyclic aromatic hydrocarbons: sources, toxicity, and remediation approaches. Front Microbiol.. 2020;5(11):562813 PMID: 33224110; PMCID: PMC7674206
- [CrossRef] [Google Scholar]
- Assessing risks to adults and preschool children posed by PM2. 5-bound polycyclic aromatic hydrocarbons (PAHs) during a biomass burning episode in Northern Thailand. Sci. Total Environ.. 2015;508:435-444.
- [Google Scholar]
- Effect of combustion variables on PAHs emission from incineration of blood contaminated cotton. Int. J. Environ. Sci. Res.. 2011;1(2):44-49.
- [Google Scholar]
- Exposure and risk assessment of PAHs in indoor air of waterpipe cafés in Ardebil, Iran. Build. Environ.. 2019;155:47-57.
- [Google Scholar]
- Characterization and combustion behavior of single-use masks used during COVID-19 pandemic. Materials.. 2021;14(13):3501.
- [CrossRef] [Google Scholar]
- United States Environmental Protection Agency, 2011. USEPA: Exposure factors handbook (Final). Washington.
- The 2005 World Health Organization re-evaluation of human and mammalian toxic equivalency factors for dioxins and dioxin-like compounds. Toxicol. Sci.. 2006;93:223-241.
- [Google Scholar]
- Variations of emission characterization of PAHs emitted from different utility boilers of coal-fired power plants and risk assessment related to atmospheric PAHs. Sci. Total Environ.. 2015;538:180-190.
- [CrossRef] [Google Scholar]
- WHO Coronavirus (COVID-19) Dashboard | WHO Coronavirus (COVID-19) Dashboard with Vaccination Data.
- Wu, H., Huang, J., Zhang, C.J.P., He, Z., Ming, W., 2020. EClinicalMedicine Facemask Short-age and the Novel Coronavirus Disease (COVID-19) Outbreak: Re Fl Ections on PublicHealth Measures. p. 21.
- Status, sources, and human health risk assessment of PAHs via foliar dust from different functional areas in Nanjing, China. J. Environ. Sci. Health A. 2018;53:571-582.
- [CrossRef] [Google Scholar]
- Emissions of unintentional persistent organic pollutants from open burning of municipal solid waste from developing countries. Chemosphere. 2011;84:994-1001.
- [CrossRef] [Google Scholar]
- Recent evidence on polycyclic aromatic hydrocarbon exposure. Healthcare (basel).. 2023;11(13):1958. PMID: 37444793; PMCID: PMC10341535
- [CrossRef] [Google Scholar]