Translate this page into:
Advancement in oral pharmacokinetics of an antibiotic Cefixime using amphiphilic 4-armed macrocyclic surfactant based niosomes
⁎Corresponding author. raza.shah@iccs.edu (Muhammad Raza Shah),
-
Received: ,
Accepted: ,
This article was originally published by Elsevier and was migrated to Scientific Scholar after the change of Publisher.
Peer review under responsibility of King Saud University.
Abstract
The four -armed long carbon chains supramolecular amphiphilic macrocycles are able to self-assemble into nanosized architectures called niosomes that have a membrane bilayer structure. In this study, amphiphilic macrocycle (MCC10) was synthesized by the cyclization of alkylated 4-hydroxybenzaldehyde with resorcinol, in acidic medium acting as a catalyst as well as a solvent, and Cefixime (Cef) was used as model entrapment drug. The drug loaded MCC10 noisomes were characterized by various spectroscopic techniques including; Maldi Toff MS, 1HNMR and FTIR. MCC10 niosomes were subjected to biocompatibility and blood haemolysis using NIH/3T3 cell line and fresh human red blood cells (RBCs), respectively. Acute toxicity study was conducted in mice. The drug loading efficiency was studied by HPLC while shape and size of MCC10 macrocycle was investigated using atomic force microscope (AFM), scanning electron microscopy (SEM) and dynamic light scattering (DLS), respectively. In-vivo oral pharmacokinetic was evaluated in rabbits. The shape of drug loaded macrocycle (Cef-MCC-10) was spherical with average size of 200.1 ± 2.24 nm, and zeta potential −18.0 ± 0.8 mV, having polydispersity index (PDI) of 0.07 and showing greater drug entrapment efficiency (%EE) of 72.86 ± 3.31%. The average size of unloaded vesicles of MCC10 was 157.7 ± 2.02 nm, the zeta potential value for unloaded formulation of MCC10 was −11.5 ± 0.71 mV, and whereas, the polydispersity index was found to be 0.24. MCC10 was screened for cell cytotoxicity study using NIH/3T3 and Hela cells at the highest concentration of 1000 μg/mL where they better cell viability of 71.00 ± 2.10% and 68.00 ± 2.15%, respectively. MCC10 niosomes showed in-vivo drug plasma concentration (Cmax) of10.29 ± 4.81 μg/mL for Cef that was higher than commercially available suspension and capsules. Therefore, MCC10 is presented as effective and safe nano-carrier for Cef to improve the oral pharmacokinetics in rabbits.
Keywords
Amphiphilic 4-armedmacrocycle
Self-assembly
Vesicles
Oral pharmacokinetics
Drug delivery
Cefixime
1 Introduction
Amphiphilic molecules with four-armed carbon chains have an ability to self-assemble in unique macrocyclic structure comprising well-defined architectures and ordered arrangement of hydrophilic and hydrophobic chains on the macrocyclic backbone, in contrast to the traditional linear amphiphiles (Ren 2011, Yu et al., 2012, Zhao et al., 2021). Basically, these amphiphilic macrocycles have surfactant-like properties which are highly useful to stabilize, transport or protect small hydrophobic drug molecules in the aqueous media, therefore, these macrocycles may also find applications in drug delivery systems (Bong et al., 2001, Förster and Plantenberg 2002). Currently, many drugs have poor solubility in water because of high lipophilicity and/or high molecular weight of drug, thus, resulting in reduced oral bioavailability and low therapeutic efficiency (Hauss 2007). Several approaches have been devised to address the problem, (Singh and Lillard Jr 2009) but, the vesicle system has been found more fascinating than the other medication delivery systems, due to their non-toxic character, biodegradability, and lipophilic arms (Uchegbu and Vyas 1998, Moghassemi and Hadjizadeh 2014). Vesicles serve as solubilizing carriers that helps in persistent discharge of drug active agents. Moreover, the size, shape and lamellar structure of vesicles can be controlled to enhance their functioning, as well as, prolonging time of circulation of drug in the blood stream and keep active agents of drug from chemical and enzymatic degradation (Heppner et al., 2001, Manosroi et al., 2004, Celentano et al., 2014, El-Ridy et al., 2015).
Niosomes are synthetic non-ionic surfactants or liposomes which has the ability to form self-assembled closed bilayer structure, comprising inner hollow aqueous compartments (Ballas et al., 2014, Asmat et al., 2016). They lead more potential than traditional liposomes because of high purity of the surfactants, economical, high physical and chemical stability, prolonged shelf-life, inclusive versatility and superior potential sterilization (Bozkurt et al., 2015). Nonetheless, the main advantage of these advanced carriers is to functionalize them accordingly to interact with specific biological targets (Eisenhauer et al., 2009, Celentano et al., 2014, Martínez and Morales 2014).
Cefixime, a third generation cephalosporin, is commonly used against various gram-negative and gram-positive bacteria, such as Escherichia coli, Haemophilus influenzae, Klebsiella pneumoniae and Neisseria gonorrhoeae that can develop resistance towards cefaclor, cephalexin, trimethoprim-sulfamethoxazole and ampicillin (Neu 1992). Cefixime is normally administered orally and being a weak acid (pKa 2.5), it is slightly soluble in water. It has been noticed that about forty to sixty percent of the drug taken orally is eliminated through the renal and biliary routes which result in low bioavailability and thus lower clinical and therapeutic efficacy. In current study, amphiphilic 4-armed macrocycle (MCC10) was synthesised as a drug delivery vehicle to boost the oral bioavailability of hydrophobic drug, Cefixime (Cef). A novel MCC10 was synthesized by cyclization of 4-hydroxyl benzaldehyde with resorcinol resulting in a macrocycle with four lipophilic chains comprising ten carbons in each arm. Cefixime loaded niosomes (Cef-MCC10) were investigated for persistent discharge of the drug, increase oral pharmacokinetics and biocompatibility.Cef-MCC10 niosomes were characterized for morphology, particle size, and precent efficiency of drug encapsulation. The oral pharmacokinetic efficacy of Cef-MCC10 was studied in rabbits and cefixime solution as a control (Varia 2010).
2 Experimental
2.1 Materials and instruments
Dimethyl sulfoxide (DMSO), Acetone, and glacial acetic acid were used as a solvent in the reaction, n-Hexane and ethyl acetate were used as mobile phase in thin layer chromatography to purify intermediate compound, whereas,dichloromethane (DCM) was used as a solvent to extract the organic compound from water. Concentrated Sulphuric acid (Conc.H2SO4) was used as a catalyst in cyclization of resorcinol and alkylated aldehyde, chloroform and methanol used as solvent. Potassium carbonate (K2CO3) was used as a mild base to abstract proton, 4- hydroxybenzaldehyde, resorcinol, 1-bromodecane were used as a chemical reagent. All the chemicals were HPLC grade and purchased from Sigma Aldrich, Germany except potassium carbonate which was purchased from local merchant. Fetal Bovine Serum (FBS), Dulbecco’s Modified Eagle’s medium (DMEM), Poly-(L)lysine (PLL), and 3-(4,5-dimethylthiazol-2-yl)-2,5-diphenyltetrazolium bromide (MTT) were purchased from sigma Aldrich, USA. Tween80 (Merck, Germany) and Cholesterol (BDH, UK) both were used as standard (reference).The deionized water was used to clean the glassware’s and dried in an oven before use. A digital pH meter (Oakton, Eutech) model 510, with an 1‘Ag/AgCl reference electrode and a glass working electrode was used to maintain the physiological pH (1.2 and 7.4) during study.
Rotary evaporator (BUCHI, 131 Rotavapor, Switzerland) was used to evaporate solvents. While, the ultrasonicator (LABSONIC L, B. Braun Biotech International, USA) was used to reduce the size of synthesized vesicles and Centrifuge (Universal 16, Hettich, Germany) to concentrate the solution and vertex (HeidolphReax Top; Heidolph Instruments, Germany) to vertex the solution.
UV–visible spectra were recorded with a double beam UV spectrophotometer (Shimadzu, UV-240, Hitachi U-3200, Japan) using quartz cuvette of 1 cm path length at a wavelength range of 190–800 nm.
Mean diameter and PDI of Cef-MCC10 were determined by Zetasizer (Malvern Instruments, UK). 1 mg/mL of nanosuspension was taken and then diluted with extra pure water before reading. After preparation, the data was collected in triplicate manner at a scattering angle of 90° at 25 °C. To obtain IR spectra of MCC10, the MCC10 was mixed with KBr separately and grinded it to make disks and scanned at 400 to 4000 cm−1.
Morphology of drug loaded niosomal vesicles of MCC10 were measured by AFM (atomic force microscopy) (Agilent, 5500, USA) and SEM (scanning electron microscopy). A drop of cefixime loaded suspension of MCC10 was placed on a mica slides, air-dried at ambient temperature and imagined in non-contact mode. For scanning electron microscopy, powder of drug loaded vesicles of MCC10 were visualized.
For the optimization of chromatographic parameters, reversed phase column (Phenomenex C8, 5 μ, 50 × 4.6 mm) was used on HPLC (Shimadzu, LC20A, Japan).
2.2 Synthesis of amphiphilic macrocyclic MCC10
HPLC grade acetone (25 mL), 690 mg K2CO3 and 488 mg (4 mmol) 4-hydroxy benzaldehyde were mixed in 100 mL flask and the reaction mixture was heated for 40 min at 60 °C under reflux, then added 4 mmol of C10H21Br (1-bromodecane) and proceeded the response at alike temperature for 12 h. The reaction mixture was cooled and washed with water to remove K2CO3 salt. The required product 4-(decyloxy)benzaldehyde (DXBH) was extracted with DCM and purified by column chromatography using 99.9 % hexane as mobile phase. The product was obtained in the form of liquid with percent yield of 91.6 %.
40 mL of glacial acetic acid was taken in a round bottom flask of 250 mL and 440.16 mg (4 mmol) resorcinol was added in it. Few drops of Conc.H2SO4 were added and refluxed the reaction mixture for 5 min at 70 °C. Then, 4 mmol of alkylated benzaldehyde (intermediate) was mixed in a response mixture and continue refluxing for 18 h at same temperature. The reaction mixture was cooled at ambient temperature, filtered it and finally solid required product MCC10 (Fig. 1) was obtained with percent yield of 86.15 %.It represents the synthesis scheme of MCC10.
Fig. 1 to be placed here.
2.3 Synthesis of Cefixime-loaded niosomal vesicles (Cef-MCC10)
Cef-MCC10 was prepared by a thin film hydration method (Wang et al., 2019). The synthesized amphiphilic macrocycle MCC10 and Cholesterol were taken in a ratio of 2:1 w/w and dissolved in combined solvents system (20 mL) of methanol and chloroform (4:6 v/v). In 25 mL methanol 10 mg of Cefixime was dissolved, and then, mixed with the solution of cholesterol and newly synthesized supramolecular amphiphilic macrocycle MCC10. The rotator evaporator was used to evaporate the organic solvent to form thin lipid bilayer under the vacuum at 40 °C temperature. To hydrate the film, the resultant lipid film was dipped in to Phosphate Buffer saline, pH 7 (10 mL) for 30 min at 60 °C. The size of niosomal vesicles of synthesized amphiphilic supramolecular macrocycle was reduced with the help of ultrasonicator.
2.4 HPLC quantification methodology
-
Preparation of Cefixime stock solution
Cefixime stock solutions were prepared in methanol (250 μg/mL) and stored in light-resistant containers at − 20 °C.
-
b.
Optimization of chromatographic parameters of Cefixime in plasma
The elution rate in the column was adjusted at various wave-lengths using UV detector to detect the Cefixime. For the better partition and recognition of Cefixime, the HPLC parameters i.e. peak resolution, peak height, asymmetry or tailing factor, peak width at half height, retention time were optimized by the used of mobile phase consisting of methanol and 0.4 M H3PO4 (15:85 v/v; pH 3), with flow rate of 1.0 mL/min at 50 °C. The above mentioned optimized conditions were used for further study. The optimum wavelength and retention time for drug (Cefixime) detection were set up at 13.5 ± 1.5 min and 288 nm, respectively. Calibration curve was obtained by standard addition method to find unknown Cefixime concentration in test samples.
-
c.
Plasma drug extraction optimization method
The extraction of drug from plasma was done by precipitation of protein, following the oral administration of Cef-MCC10. Plasma (200 μL) was mixed in to acetonitrile (600 μL), vortexed for 1 min and then, centrifuged the resultant mixture at 6000 rpm for 10 min. To determine the concentration of Cef-MCC10, an aliquot of above solution (50 μL) was injected into HPLC.
-
d.
Validation method
Calibration curve of plasma was obtained by examined the samples in triplicate manner with three dissimilar days to evaluate accuracy, precision, recovery, stability, linearity, correlation coefficient, limit of quantification (LOQ), and limit of detection (LOD).
-
e.
Evaluation of drug entrapment efficiency (%EE)
MCC10 Entrapment efficiency for Cefixime was evaluated by HPLC.Cef-MCC10 solution (1 mL) consisting of 1 mg of cefixime was taken and centrifuged for 25 min at 12,000 rpm to separate untrapped Cefixime in a form of pellets. This process was repeated thrice to remove the total untrapped drug from the niosomal vesicular suspension. Pellets consisting of cefixime encapsulated in niosomal vesicular suspension were dissolved in methanol (10 mL volume), then vertexed it for one minute and for ten minutes at10,000 rpm was centrifuged. A small volume about 50 μL of supernatant was injected into the HPLC using methanol and 0.4 M H3PO4 (15:85 v/v; pH 3) as mobile phase having flow rate of 1.0 mL/min at50°C temperature. The retention period for cefixime was observed at 13.5 ± 1.5 min with wavelength of 288 nm. Calibration curve was obtained by cefixime (1–10 μg/mL) solution used as a standard prepared in methanol using same parameters on HPLC.
The entrapment efficiency in percent was calculated by:
Where, Aen is amount of Cefixime entrapped in MCC10 formulation and At represents the total drug in the niosomal suspension of MCC10.
2.5 Drug release behaviour of Cef-MCC10
The cefixime loaded niosomal vesicular suspension of MCC10 consisting of 5 mg drug having a volume of 5 mL was added in poured in to a dialysis membrane with 12x103 KDa. The 50 mL of PBS having different pH i.e 1.2 and 7.4 were added in two different 100 mL beakers separately and then dialysis membrane filled with cefixime loaded niosomal vesicular suspension of MCC10 was put in the beakers separately. Placed these beaker having PBS of two pH in a shaker (Thermo Scientific MAXQ 430 HP) having a stirring speed of 100 rpm at 37 °C. After specific time interval, 2 mL of PBS present in media was withdrawn and added same amount of fresh PBS to avoid cefixime saturation. The released of cefixime in the PBS was measured with the use of UV–visible spectroscopy at a wavelength of 288 nm.
2.6 Using experimental animals for bioavailability and biocompatibility
The reference number and study protocol is given in supplementary materials.
-
In-vivo acute toxicity
The complete description about in-vivo acute toxicity studies is given in our previous published articles (Akuodor et al., 2013, Imran et al., 2016, Ali et al., 2022).
-
b)
Blood haemolysis assay
The blood haemolysis of synthesized MCC10 was performed using fresh human blood. For the separation of erythrocytes from plasma, it was centrifuged at 700g for ten minutes, therefore, the erythrocyte pellets were settled down. The erythrocytes pellets were collected, washed thrice with PBS (pH 7.4), centrifuged (700g) for 10 min. A suspension was prepared by dissolving 1g erythrocytes in 10 mL PBS. In 0.2 mL erythrocytes suspension the known volume (4 mL) of test samples with concentration ranges from 62.5 to 1000 μg/mL were added in it. As a reference tween80 was used during the study. All these samples were left for incubation till 4 hrs and after passing 4 hrs, the samples were centrifuged at 700g about ten minutes to remove non-haemolysed erythrocytes. The liquor was collected, and then analyzed for the released haemoglobin on UV–Visible spectrophotometer at 540 nm. For 100 and 0% haemolysis, the erythrocyte suspension was added to distilled water and PBS, respectively. The percent haemolysis was calculated by:
Where, Abs, Abs0 and Abs100 are the absorbance of the test samples, a solution of 0% haemolysis and a solution of 100% haemolysis, respectively(Devescovi et al., 2008, Granchi et al., 2008).
-
c)
Cytotoxicity study
NIH/3T3 (Mouse embryonic fibroblast) and Hela cell lines were seeded in DMEM consisting of penicillin and streptomycin (100 units/mL) in humidified atmosphere comprising 5% CO2 and PBS (10%) at 37 °C. The synthesized MCC10 against both cell lines NIH/3T3 and Hela cells were seeded in 100 μL of culture medium, into 96-well plates at a density of 5x104 and 6x104 cells/well, respectively. Incubated for 24 h and then original medium was replaced with 200 μL of fresh medium comprising different concentrations from 62.5 to 1000 µg/mL of test samples. Moreover for negative control, cells were incubated with DMEM only for 24 h. Then, in each well, MTT solution (200 μL; 0.5 mg/mL in PBS) was added. After 4 h of incubation, the medium consisting of unreacted dye was separated, while the purple formazan crystals formed were dissolved in 100 μL per well DMSO and the absorbance was measured on micro plate reader (Spectra Max plus, Molecular Devices, CA, USA) at 570 nm wavelengths. PLL and Tween 80 were used as standards and positive control, respectively. Cell viability of test samples was calculated by:
Cell viability (%) = (XAbs of Test Sample) / (XAbs of Negative Control) × 100.
Where, XAbs represents the mean absorbance.
2.7 Oral pharmacokinetic study
A species of rabbits called Oryctolagus Cuniculus were used to study the oral pharmacokinetic of cefixime. Eighteen male rabbits, weighing with 1.9 Kg average weight of were housed in favourable conditions i.e. 12 h day-night cycles having free access to water and at 25 °C. The rabbits were fasted for 12 hrs before use in experiment. After that rabbits were classified in two group having six rabbits in each group. Group I rabbits were treated with Cef-MCC10 niosomal vesicular formulation6 mg/kg body weight orally. While, the Group II rabbits were treated with commercial Cefixime suspension orally at 6 mg/kg per body weight. 1 mL blood samples of each Cef-MCC10 and Commercial Cefixime suspension (Maxpan) treated rabbits were collected in heparinized tubes at time intervals of 0, 0.5, 1, 2, 4, 6, 8, 12, 16 and 24 h from their (marginal) ear vein by the used of insulin plastic syringe. Centrifuged the entire collected blood sample at 6000 rpm for 10 min to separate the plasma from blood and then preserved at − 80 °C for additional studies.
2.8 Statistical analysis
All experiments were carried out in triplicate and results were expressed as mean ± Standard error (SE). P value (null-hypothesis significance testing) a lower than 0.05 were acceptable statistically significant.
3 Results and discussion
3.1 Synthesis and characterization of MCC10
The detail of synthesis and characterization of supramolecular amphiphilic macrocycle MCC10 are given in supplementary file.
3.2 Synthesis and characterization of Cef-MCC10
Cef-MCC10 was prepared by a thin film hydration method and characterized by AFM and SEM. Cef-MCC10 vesicles of newly synthesized nano-carrier (MCC10) were found to be spherical in shape, Fig. 2A, 2B and 2C. The Fig. 2A and 2B are two dimensional and three dimensional images of atomic force microscopy (AFM), whereas; Fig. 2C represents the scanning electron microscopy (SEM). The average size of Cef-MCC10 measured by zeta sizer was 200.1 ± 2.24 nm, having a zeta potential of −18.0 mV with PDI value of 0.07, as shown in, Fig. 3 (A, B). The small particle size and PDI value indicated that most of the particles of Cef-MCC10 vesicles are undispersed and have greater number of Cef-MCC10 vesicles. Similarly, average size of empty niosomal vesicles of newly supramolecular amphiphilic macrocycle MCC10 was found to be 157.7 ± 3.02 nm, zeta potential and polydispersity index values were obtained as −11.5 ± 0.71 mV and 0.24 as depicted in Fig. 3 (C & D).The results showed that due to inner hollow cavity in the structure of MCC10 and four lipophilic carbon chains, it has greater ability to entrap larger amount of drug molecules (Imran et al., 2016).AFM images of drug loaded niosomal vesicles of MCC10 (2A &2B), and SEM image of drug loaded niosomal vesicles of MCC10 (C).
The zeta size (a) and zeta potential (b) graph of drug loaded niosomal vesicles of MCC10 and similarly, zeta size (C) and zeta potential (D) graph of unloaded niosomal vesicles of amphiphilic macrocycle MCC10.
3.3 Calibration curves, linearity, accuracy and precision
The calibration curve showed linearity for Cef in the concentration range of 1–10 μg/mL with correlation coefficient of 0.9978, in the plasma as depicted in Fig. 7. For the determination of inter-day assays, triplicate QC samples were analysed for three different days. While, for intra-day assays each QC (quality control) plasma sample was analysed in triplicate thrice a day. The results of intra and inter-day assays were represented as % CV (percent of coefficient of variation) (precision) and mean percentage (accuracy) of the analyte recovered in the assay, Table 1. It was found that precision of calibration standards; QCs of inter and intra-day and mean accuracy were within the acceptance limits.
Nominal Concentration (μg/mL)
Mean Calculated Concentration (μg/mL)
Accuracy (%)
Precision (%)
Inter-day
3
2.87
95.66
2.09
5
4.69
93.80
2.15
8
7.75
96.87
1.09
3.4 Percent drug encapsulation efficiency (%EE)
The greater capability of nanocarrier basically depend on the nature of drugs, lipophilicity, also interaction of functional group present in the drug, nanocarriers, and cholesterol which give the hardness to the lipid bilayer and enhance the drug loading capability inside niosomal vesicular formulation (Ellis and Kirby 1980, Tavano et al., 2013, Waddad et al., 2013).The presence of cholesterol also gives the greater stability to lipid bilayer. HPLC studies showed that, the niosomal formulation has %EE for Cef as high as 72.86 ± 3.31 %, shown in Table 2. Increase in %EE might be as described to four long carbon chains (HLB = 12.09) (Maserati et al., 2008) attached to the hydrophilic part in a structure of MCC10. The non-ionic surfactants which possess a unique properties to form niosomes have great capacity to encapsulate the drug ranging from 60 to 70% EE (Jemal et al., 2005). Therefore, MCC10 was supposed to be the most appropriate carrier for a drug Cef in future applications. ± 3.31 %
Vesicles
Average size (nm)
Zeta potential (mV)
PDI
%Drug encapsulation efficiency
Drug loaded niosomal vesicles
200.1 ± 2.24
−18.0 ± 0.8
0.07
Unloaded vesicles
157.7 ± 3.02
−11.5 ± 0.71
0.24
--------------------
3.5 In-vitro drug release study
In-vitro drug releasing behaviour of Cef-MCC10 was observed at two different pH i.e.,1.2 and 7.4. It was noted that the Cef-MCC10 are stable at both pH values and did not illustrate any quick release of the drug throughout the study. It is noticed that within first 8 hrs of the study, the maximum drug was released at pH 7.4 which is 59.54 ± 1.34 %, and similarly at pH 1.2, amount of drug released is 52.45 ± 1.42 %, as can be seen in Fig. 4.The % drug released behaviour of cefixime loaded niosomal vesicles of MCC10.
Therefore, the results showed that drug release behaviour was constant at physiological pH and support the suitability of MCC10 for both oral and intravenous administration (Uchegbu and Florence 1995, Bayindir and Yuksel 2010, El-Laithy et al., 2011, Imran et al., 2017, Ali et al., 2018).
3.6 Biocompatibility study
-
Blood Haemolysis
Haemoglobin starts releasing out of the cells to plasma due to leakage in a wall of erythrocytes. This phenomenon of releasing haemoglobin in plasma is called blood haemolysis. A quantitative analysis of this released haemoglobin provides information about potential damage to red blood cells (RBCs). The one of the reasons of haemolysis is, when amphiphiles attached with the wall of erythrocyte made bilayer-to-micelle transition because of lipid/surfactant molar ratio (Partearroyo et al., 1992). Therefore, it is necessary to screen the biomaterials for their haemolytic effect prior in-vivo/in-vitro animal studies. MCC10 was found to be RBCs compatible in the acceptable range of haemolytic toxicity from tested concentrations of 62.5 to 1000 μg/mL, as shown in Fig. 5. It inducedless haemolysis (15.43 ± 1.76%) even at the highest concentration of 1000 μg/mL. The Tween80 showed 25.54 ± 1.80 % at equivalent concentration of 1000 μg/mL, as a positive control (Fig. 5). Low haemolytic toxicity of newly synthesized MCC10 can be attributed to its 4-armed lipophilic chains accompanying hydrophobicity as discussed in previous studies (Paecharoenchai et al., 2014). These results favourMCC10 to be suitable for drug delivery applications with high haemo-compatibility.
-
Cell cytotoxicity
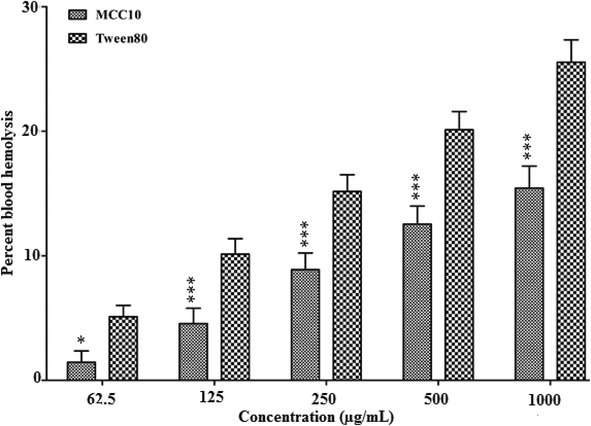
- The % blood hemolysis of newly synthesized nanocarrier MCC10.
Synthesized MCC10 was investigated for its cell viability by screening out for 3 T3 and Hela cell line. It was noted that the MCC10 showed lower toxicity as compared to reference Tween80 even at the highest concentration i.e.,1000 µg/mL and has greater cell viability of 71.00 ± 2.10 % at 1000 µg/mL as compared to standard having cell viability of about 58.13 ± 2.15 % after 24 hrs at same concentration, as shown in Fig. 6 (A). Similarly, the results of Hela cell line indicate that the MCC10 showed lower cell toxicity as compared to Tween80. MCC10 showed greater cell viability of 68 ± 2.15%, as compared to reference, at 1000 µg/mL where a cell viability of 54.76 ± 2.10% was obtained for Tween80 (Fig. 6B).So, these results indicated that the MCC10 was completely biocompatible. Its non-toxicity was mainly due to having four lipophilic carbon chains which imparted inertness in its structure and developed saturation in its nature as reported elsewhere in literature (Aramaki et al., 2015, Imran et al., 2016).The % cell viability at 3 T3 cell line after 24 (A) and HeLa cell line (B) after 24hrsincubation of synthesized amphiphilic macrocycle MCC10.
3.7 In-vivo acute toxicity
It is an essential and vital parameter to develop the in-vivo toxicity assays in the animal model to make the drug delivery system safe and useful (Xu et al., 2013). The study was performed in two phases by injecting the animals intraperitoneally to evaluate the LD50 dose in which the highest amount of nanocarriers were given to the animal until survival is 50%, as depicted in (Table 3). It was noted that in both phases of screening, all animals in all groups survived up to 2 g/kg, following intraperitoneal insertion.Table 4.C.
Dose (mg/kg body weight)
1st stage
Group 1
Group 2
Group 3
(100 mg)
(500 mg)
(1000 mg)
Alive
Alive
Alive
2nd stage
Group 1
Group 2
(1500 mg)
(2000 mg)
Alive
Alive
Pharmacokinetic parameters
Oral Control
CEF-MCC-10 Formulation
Dose (mg/Kg)
5 ± 0.73
5 ± 0.27
Cmax (µg/mL)
4.01 ± 0.48
10.29 ± 4.81***
AUC0-24 (µg.h/mL)
67.06 ± 2.1
139.42 ± 2.7***
AUMC0-24 (µg.h2/mL)
784.53 ± 6.2
2845.86 ± 9.6***
T1/2 (h−1)
16 ± 0.31
23.72 ± 1.43***
MRT (h)
11.69 ± 0.15
13.23 ± 0.13***
Tmax (h)
6
16
Clearance (L/h.Kg)
0.044 ± 0.03
0.017 ± 0.0043 ns
Volume distribution (L)
1.05 ± 0.67
0.59 ± 0.32 ns
3.8 Pharmacokinetic studies
The average concentration of Cef in the plasma, achieved from niosomal formulation of MCC10 and control solution is given in Table 4. Drug plasma concentration curves are represented in Fig. 8. When Cef was administered in the form of Cef-MCC10, relatively higher drug concentration of 10.29 ± 4.81 μg/mL was observed in comparison with control 4.01 ± 0.48 μg/mL, respectively. The Cmax of the Cef-MCC10 was 2.5 folds higher than its control solution. Moreover, Cef-MCC10 showed sustain drug release profile having Tmax of about 16 hrs with reference to its control that was 6 hrs. With controlled release behaviour, Cef-MCC10 vesicles showed stability after oral administration owing to its greater mean residence time (MRT) 13.23 ± 0.13hrs and lesser clearance rate 0.017 ± 0.0043L/h in assessment. The control solution of Cef showed a clearance rate of 0.044 ± 0.03L/h and MRT of MRT 11.69 ± 0.15 h. The improved pharmacokinetics of Cef-MCC10 can be attributed to characteristics like nano size of Cef-MCC10, presence of the inner hollow cavity, its bilayer structure and lipophilic nature that facilitate more efficient hosting and enhanced absorption of the drug via gastric membrane and hence, enhance its bioavailability (Attia et al., 2007, Imran et al., 2016, Imran et al., 2017). Outcomes of the analysis depicted that the synthesized niosomes vesicles would be an effective pharmaceutical matrix for enhancing the oral bioavailability of Cef with controlled drug releasing behaviour.Calibration curve for Cefixime showing linearity in analytical range of 1–10 μg/mL with R2 value of 0.9978.
Plasma drug concentration of Cefixime loaded in MCC10 based niosomal vesicles and its solution at different time intervals, after oral administration at 5 mg/kg body weight dose (n = 5, mean ± SE).
4 Conclusion
Many antibiotics having greater potential against the diseases are available in the market but their oral bioavailability in the body is too low. This leads to erratic pharmacokinetics, low drug availability at the site of infections and development of multi drug resistance. To increase their bioavailability different kinds of niosomal formulations were designed in this study, which are easily synthesized and economical. An amphiphilic4-armed macrocycle MCC10 is a niosomal formulation synthesized with an average hydrodynamic size of 200 nm to enhance the entrapment efficiency of Cef and final formulation presented low blood haemolysis and cytotoxicity. The niosomes sustained the drug release up to 8 hr and only 59 and 52% of the drug was release at pH 7.4 and 1.2, indicating the suitability of the formulation for oral and intravenous administration. The formulation was studied for oral pharmacokinetics in rabbits and oral bioavailability was determined using the pharmacokinetic parameters and quantification of drug in plasma. The maximum plasma concentration achieved was 10 µg/mL as compared to Cef solution that resulted in 4 µg/mL drug concentration. The mean residence time of the drug was also increase from 11 hrs (Cef solution) to 13 hrs (Cef-MCC10). Synthesized amphiphilic macrocycle was found to be more biocompatible and has high capability to entrap the greater amount of antibiotic Cef. Therefore, it is recommended that MCC10 is secure and useful as nanocarrier which can enhance the cefixime oral bioavailability.
Acknowledgements
Authors are thankful to the Researchers Supporting Project Number (RSP2023R110) at king Saud university Riyadh Saudi Arabia for financial support.
Declaration of Competing Interest
The authors declare that they have no known competing financial interests or personal relationships that could have appeared to influence the work reported in this paper.
References
- Gastroprotective effect of the aqueous leaf extract of Guiera senegalensis in albino rats. Asian Pac. J. Trop. Med.. 2013;6:771-775.
- [Google Scholar]
- Hemolytic and cellular toxicology of a sulfanilamide-based nonionic surfactant: a niosomal carrier for hydrophobic drugs. Toxicol. Res.. 2018;7:771-778.
- [Google Scholar]
- Synthetic star shaped tetra-tailed biocompatible supramolecular Amphiphile as an efficient nanocarrier for Amphotericin B. Chem. Phys. Lipids 2022:105257.
- [Google Scholar]
- Formation of bilayer membrane and niosomes by double-tailed polyglyceryl-type nonionic surfactant. Langmuir. 2015;31:10664-10671.
- [Google Scholar]
- Diabetes mellitus and oxidative stress—a concise review. Saudi Pharma. J.. 2016;24:547-553.
- [Google Scholar]
- Influence of a niosomal formulation on the oral bioavailability of acyclovir in rabbits. AAPS PharmSciTech. 2007;8:206-212.
- [Google Scholar]
- Irim at TRECVID 2014: Semantic indexing and instance search. In: Proceedings of TRECVID. 2014.
- [Google Scholar]
- Characterization of niosomes prepared with various nonionic surfactants for paclitaxel oral delivery. J. Pharm. Sci.. 2010;99:2049-2060.
- [Google Scholar]
- Trends in distance education research: A content analysis of journals 2009–2013. The International Review of Research in Open and Distributed Learning; 2015. p. :16.
- Design of an industrially feasible twisted-stack HTS cable-in-conduit conductor for fusion application. IEEE Trans. Appl. Supercond.. 2014;24:1-5.
- [Google Scholar]
- Growth factors in bone repair. La Chirurgia degli organi di movimento. 2008;92:161-168.
- [Google Scholar]
- New response evaluation criteria in solid tumours: revised RECIST guideline (version 1.1) Eur. J. Cancer. 2009;45:228-247.
- [Google Scholar]
- Novel sugar esters proniosomes for transdermal delivery of vinpocetine: preclinical and clinical studies. Eur. J. Pharm. Biopharm.. 2011;77:43-55.
- [Google Scholar]
- A comparison of spring barley grown in England and in Scotland. 2. yield and its components. J. Agric. Sci.. 1980;95:111-115.
- [Google Scholar]
- Niosomal encapsulation of ethambutol hydrochloride for increasing its efficacy and safety. Drug Deliv.. 2015;22:21-36.
- [Google Scholar]
- From self-organizing polymers to nanohybrid and biomaterials. Angew. Chem. Int. Ed.. 2002;41:688-714.
- [Google Scholar]
- Sensitivity to implant materials in patients with total knee arthroplasties. Biomaterials. 2008;29:1494-1500.
- [Google Scholar]
- The tumor suppressor protein menin interacts with NF-κB proteins and inhibits NF-κB-mediated transactivation. Oncogene. 2001;20:4917.
- [Google Scholar]
- Sugar-based novel niosomal nanocarrier system for enhanced oral bioavailability of levofloxacin. Drug Deliv.. 2016;23:3653-3664.
- [Google Scholar]
- Glycoside-based niosomal nanocarrier for enhanced in-vivo performance of Cefixime. Int. J. Pharm.. 2016;505:122-132.
- [Google Scholar]
- Double-tailed acyl glycoside niosomal nanocarrier for enhanced oral bioavailability of Cefixime. Artif. Cells Nanomed. Biotechnol.. 2017;45:1440-1451.
- [Google Scholar]
- Trends in the leading causes of death in the United States, 1970–2002. JAMA. 2005;294:1255-1259.
- [Google Scholar]
- Stability and transdermal absorption of topical amphotericin B liposome formulations. Int. J. Pharm.. 2004;270:279-286.
- [Google Scholar]
- ¿ Puras Groserías?: rethinking the role of profanity and graphic humor in Latin@ Students' Bilingual Wordplay. Anthropol. Educ. Q. 2014;45:337-354.
- [Google Scholar]
- Maserati, E., C. Panarello, C. Morerio, et al., 2008. Clonal chromosome anomalies and propensity to myeloid malignancies in congenital amegakaryocytic thrombocytopenia (OMIM 604498). haematologica. 93, 1271-1273.
- Nano-niosomes as nanoscale drug delivery systems: an illustrated review. J. Control. Release. 2014;185:22-36.
- [Google Scholar]
- Nonionic surfactant vesicles composed of novel spermine-derivative cationic lipids as an effective gene carrier in vitro. AAPS PharmSciTech. 2014;15:722-730.
- [Google Scholar]
- Effective detergent/lipid ratios in the solubilization of phosphatidylcholine vesicles by Triton X-100. FEBS Lett.. 1992;302:138-140.
- [Google Scholar]
- Biodegradable poly (lactic acid): synthesis, modification, processing and applications. Springer Science & Business Media; 2011.
- Transferrin-conjugated pluronic niosomes as a new drug delivery system for anticancer therapy. Langmuir. 2013;29:12638-12646.
- [Google Scholar]
- Non-ionic surfactant vesicles (niosomes): physical and pharmaceutical chemistry. Adv. Colloid Interface Sci.. 1995;58:1-55.
- [Google Scholar]
- Non-ionic surfactant based vesicles (niosomes) in drug delivery. Int. J. Pharm.. 1998;172:33-70.
- [Google Scholar]
- Varia, U. R. K., 2010. Effect of cyclodextrin inclusion complex on dissolution behaviour of cefixime, RGUHS.
- Formulation, characterization and pharmacokinetics of Morin hydrate niosomes prepared from various non-ionic surfactants. Int. J. Pharm.. 2013;456:446-458.
- [Google Scholar]
- Liposome encapsulation of oncolytic virus M1 to reduce immunogenicity and immune clearance in vivo. Mol. Pharm.. 2019;16:779-785.
- [Google Scholar]
- Giant molecular shape amphiphiles based on polystyrene–hydrophilic [60] fullerene conjugates: Click synthesis, solution self-assembly, and phase behavior. J. Am. Chem. Soc.. 2012;134:7780-7787.
- [Google Scholar]
- Soft materials by design: unconventional polymer networks give extreme properties. Chem. Rev.. 2021;121:4309-4372.
- [Google Scholar]