Translate this page into:
Black pepper (Piper nigrum L.) essential oil counteracts dexamethasone-induced hepatic injury via modulating PGC-1α/ PPAR-α pathway in rats
⁎Corresponding authors. mona_pharmacology@yahoo.com (Mona F. Mahmoud), mfabdelaziz@zu.edu.eg (Mona F. Mahmoud), mansour.sobeh@um6p.ma (Mansour Sobeh)
-
Received: ,
Accepted: ,
This article was originally published by Elsevier and was migrated to Scientific Scholar after the change of Publisher.
Abstract
We previously characterized the volatile compounds of black pepper (Piper nigrum) essential oil (BPEO) and demonstrated its mitigating effect against dexamethasone-induced pancreatic damage. Herein, we investigated the protective effect of the oil against dexamethasone-induced hepatotoxicity in rats and pinned the possible underlying mechanisms. In the in vivo experiment, male Wistar rats were divided into five groups. The first group served as the normal control receiving a saline solution (Control). The second group was subjected to hepatic damage through dexamethasone exposure (Dexa) at 10 mg/kg. The third group received both dexamethasone and metformin (Met) at a dose of 50 mg/kg. The remaining two groups were exposed to dexamethasone along with black pepper oil administration at low and high doses (BPEO, 0.5 mL/kg and 1 mL/kg). We showed that BPEO offset toxicity manifestations, as indicated by reducing monocyte infiltration, hepatocyte degeneration and vacuolation, and reduction of ALT and AST levels. Moreover, relative to the dexamethasone-treated rats, BPEO declined the number of PGC-1α positive hepatocytes and activated the expression of PPAR-α in the liver. BPEO showed comparable hepatoprotective potency to the standard drug, metformin, especially at the higher dose tested (1 mL/kg). This study showcases the promising potential of BPEO in thwarting dexamethasone-induced adverse effects on the liver by alleviating hepatic biomarkers hikes and preventing histopathological changes and fibrosis. Finally, it opens a new avenue on the potential role of BPEO in modulating steroid-induced hepatic injury through the PGC-1α/ PPAR-α pathway.
Keywords
Black pepper essential oil
Dexamethasone
Liver injury
Oxidative stress
PGC-1α/PPAR-α pathway
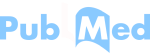
1 Introduction
Glucocorticoids, a subset of corticosteroids, are frequently utilized for managing a spectrum of conditions such as inflammation, allergies, and autoimmune disorders. They exert their effects on immune cells, tissues, and organs through both genomic and nongenomic mechanisms (Stahn and Buttgereit, 2008). While glucocorticoids are highly effective as strong anti-inflammatory, immunosuppressive, and anti-allergic properties, they can lead to significant adverse effects and toxicities (Ingawale and Mandlik, 2020). This can affect many different systems in the body, among them the endocrine, musculoskeletal, cardiovascular, and immune systems, leading to serious complications and negative health outcomes. As a result, it is always important to closely monitor and manage the use of glucocorticoids in medical treatment (Ingawale and Mandlik, 2020, Reichardt et al., 2021).
Dexamethasone is a synthetic glucocorticoid medication frequently used to manage conditions such as allergies, respiratory illnesses, skin disorders, and certain types of cancer (Stahn and Buttgereit, 2008, Mahmoud et al., 2022a, Motafeghi et al., 2023). It has been also included in the treatment regimen of hospitalized patients with coronavirus disease 2019 (COVID-19) (von Mässenhausen et al., 2022). Dexamethasone's anti-inflammatory and immune-suppressing effects are mainly mediated by cytosolic glucocorticoid receptors. This occurs through modulation of gene expression, leading to increased production of anti-inflammatory proteins (transactivation) and decreased production of proinflammatory proteins (transrepression) (Stahn and Buttgereit, 2008). However, the successful therapeutic use of dexamethasone in many inflammatory diseases is limited by the several adverse effects it induces such as insulin resistance and diabetes (Mahmoud et al., 2022b), steatosis (interference with fatty acid metabolism) (Burén et al., 2008), endocrine disorders (Jux et al., 1998) and skeletal muscle atrophy (Abou-Seif et al., 2019, Hasona and Morsi, 2019). As the liver plays a crucial role in metabolizing and detoxifying several xenobiotics and maintaining overall health, it is particularly susceptible to the toxic effects of these synthetic chemicals. The administration of dexamethasone is indeed accompanied by the production of potentially hepatotoxic reactive metabolites, where the substantially reported dexamethasone-induced hepatotoxicity was linked to the excessive formation of free radicals and oxidative stress. The dexamethasone-induced liver injury is often manifested by significant hikes in the functional hepatic biomarkers such as liver enzymes, lipid peroxidation, and hematological and immunohistochemical alterations as well as decreases in antioxidant activities (Hasona and Morsi, 2019). At the cellular level, dexamethasone increases the mitochondrial membrane potential and oxidation. This promotes the cellular metabolism, ATP production, and formation of reactive radical and non-radical species (Cikman et al., 2015).
Currently, intensive research focuses on the development of novel natural products as chemoprotective agents to alleviate the unwanted effects of glucocorticoid therapy. In fact, many dietary compounds and plant constituents have been shown to significantly reduce the manifestations of liver injury induced by dexamethasone and restore the antioxidant machinery in the liver. These include numerous plant extracts and essential oils; among them the garden thyme (Thymus vulgaris) (Abou-Seif et al., 2019) and coriander essential oil (Mahmoud et al., 2022a) as well as several phytocompounds such as betulinic acid (Zhu et al., 2018). The use of phytochemicals as a protective therapeutic strategy is usually regarded as bio-safe and cost-effective, thus standing out as one of the most promising approaches to ameliorate the deleterious effects of the currently in-use synthetic agents (Mahdi et al., 2022).
Black pepper (Piper nigrum L.), the king of spices, is a spice derived from the unripe fruit of the pepper plant. It is native to the Indian subcontinent and has been used for thousands of years for culinary purposes and as a medicinal remedy. It is also a rich source of calcium, magnesium, iron, phosphorus, and potassium, as well as carbohydrates, fats, proteins, and vitamins (B1, B2, B3, and C) (Takooree et al., 2019, Mahmoud et al., 2022a). The plant has a long history of use in traditional medicine to improve appetite and digestion, treat worm infestations, soothe hemorrhoids, relieve coughing, reduce fevers, and treat epilepsy and dysentery. Additionally, it is commonly used in the perfume and preservative industries (Damanhouri and Ahmad, 2014).
In terms of phytochemical composition, black pepper fruits contain essential oil and piperine alkaloid as the predominant constituents, which have been shown to exhibit important biological and pharmacological activities such as antioxidant, antimicrobial, anticancer, antidiabetic, anti-inflammatory, anticonvulsant, analgesic, and neuroprotective effects (Takooree et al., 2019, Mahmoud et al., 2022a). Although the main biologically active ingredient found in P. nigrum is piperine, other compounds such as piperic acid, catechin, piperlonguminine, myricetin, quercetin, β-carotene, lutein, (-)-kusunokinin, piperettine, piperamide, pellitorine, and piperolein B were also detected in significant amounts and demonstrated diverse biological activities (Nwofia et al., 2013, Takooree et al., 2019). In addition, black pepper essential oil was shown to exhibit many biological and pharmacological activities such as antioxidant, anti-inflammatory, antinociceptive (Jeena et al., 2014), antimicrobial and antiproliferative activities (Morsy and Abd El-Salam, 2017). Noteworthy, the EO significantly reduced CCl4-induced injury in mice, suggesting its potential applications as a liver health-promoting product (Zhang et al., 2021). Likewise, the hepatoprotective effects of BPEO is worth studying as it may offer a natural alternative to mitigate and/or counteract the harmful impact of dexamethasone on the liver. In addition, investigating the mechanisms through which BPEO may protect the liver in the context of dexamethasone exposure holds promise for developing natural strategies to protect against drug-induced liver damage.
We previously identified the volatile constituents of the black pepper fruits essential oil (BPEO) using GC–MS analysis and annotated 26 compounds, out of which, 7 were predominant; namely myrcene (2.43 %), α-thujene (2.8 %), α-copaene (5.21 %), α-pinene (6.51 %), β-pinene (12.01 %), sabinene (13.11 %), and E-caryophyllene (20.49 %) (Mahmoud et al., 2022a). As the plant has demonstrated antioxidant and anti-inflammatory effects, we sought to study its potential to mitigate the adverse hepatotoxic effects induced by dexamethasone. Additionally, examining the influence of BPEO on dexamethasone-induced liver injury could offer valuable insights into potential natural interventions to protect liver health during corticosteroid treatment. Furthermore, limited research was carried out using animal models. Hence, in this study, we investigated the potential protective effects of BPEO against dexamethasone-induced liver damage in rats. Using metformin as the reference drug, we monitored the effect of BPEO on the enzymatic parameters of liver, oxidative stress markers, liver tissues, apoptosis biomarkers, hepatic fibrosis, and peroxisome proliferator-activated receptor alpha (PPAR-α) and PPAR-coactivator 1 (PGC-1α) signaling in rats exposed to acute dexamethasone administration.
2 Materials and methods
2.1 BPEO hydro-distillation
Black pepper fruits were purchased from a local market in Zagazig city, Sharqia, Egypt, ground and subjected to hydro-distillation for 2 h using a Clevenger apparatus. The resulting oil was stored in a dark vial at 4 °C for future analysis (Mahmoud et al., 2022a).
2.2 Animals
Male Wistar rats (180–200 g) were used for the in vivo experiments. They were provided by the animal house of the Faculty of Veterinary Medicine in Zagazig, Egypt. The rats were kept in cages (3 animals/cage) made of polypropylene with bedding of wood shavings. They were subjected to a 12-hour light–dark cycle at a temperature of 25–30 °C with free access to food and water (Mahmoud et al., 2022a).
2.3 Experimental design
In this study, rats were randomly divided into 5 groups (n = 6). To induce hepatic injury, dexamethasone (EPICO Co., 10th of Ramadan city, Egypt) was prepared in normal saline solution (10 mg/kg body weight) and daily injected subcutaneously into the rats at the same time for four days. The first group was the normal control group, where the rats only received the vehicle by corresponding routes of administration. The second group was the hepatic damage group, where the rats received dexamethasone (10 mg/kg body weight) for 4 consecutive days. The third group of rats was exposed to dexamethasone injection preceded by oral administration of metformin (GlaxoSmithKline pharmaceutical CO, Cairo, Egypt) prepared in 10 % of gum acacia at 50 mg/kg dose. The fourth and fifth groups were treated with dexamethasone and BPEO at a low dose (0.5 mL/kg/day) and high dose (1 mL/kg/day) prepared in olive oil, respectively. Treatments were given by oral gavage once a day, 1 h before the dexamethasone injection. The rats' weights were measured before and by the end of the experiment (Mahmoud et al., 2022a).
2.4 Sample collection and preparation
On the fifth day of the experiment, the rats were anesthetized with thiopental sodium (40 mg/kg) intraperitoneal injection. The blood samples were then collected and allowed to coagulate, centrifuged to separate the serum that was stored at −80 °C to be used for biochemical analysis (Mahmoud et al., 2022a). Cervical dislocation was then applied to euthanize the rats, where their livers were carefully dissected, washed with saline solution, covered with filter papers to dry, and weighed. Livers were fixed in neutral formalin (10 %) and processed for immunohistochemical and histopathological analysis (Mahmoud et al., 2022a).
2.5 Liver enzymes measurement
As the most common liver injuries are manifested by elevated alanine aminotransferase (ALT) and aspartate aminotransferase (AST), the serum activities of these two enzymes were colorimetrically determined in the collected blood samples using a commercial kit from (Spectrum 111 Diagnostics, Cairo, Egypt).
2.6 Histological examination
To analyze the histological changes in the collected livers, fixed tissue slices (formalin 10 %) were dehydrated in four concentrations (100 %, 90 %, 70 %, and 50 %) of alcohol and then submerged in paraffin. The samples were thinned to 5 μm thickness and stained according to the hematoxylin and eosin (H&E).
2.7 Measurement of PGC-1α and PPAR-α
To perform the immuno-histochemical staining, formalin-fixed, paraffin-embedded tissue sections of 3–4 μm thickness were utilized with monoclonal anti-PPAR-α (1: 200, Service bio) and polyclonal antibodies against PGC1-α (Abcam 1:100) (Liu, 2016). The standard avidin–biotin peroxidase using diamino-benzidine (DAB) and Mayer’s hematoxylin techniques were applied for visualization and counterstaining, respectively.
2.8 Morphometric study
The numbers of PPAR-α and PGC1 α positive hepatocytes in 10 randomly selected high power microscopic fields were determined using a computerized image system composed of a Leica Qwin 500 image analyzer connected to a Leica microscope (Leica, Cambridge, UK). This number was expressed as cell number per μm2 (Yao et al., 2023).
2.9 Molecular docking study
The previously identified compounds in BPEO were docked into the ligand binding pocket of PPAR-α, whose crystal structure along with the co-crystallized agonist, 3-[5-methoxy-1-(4-methoxy-benzenesulfonyl)-1H-indol-3-yl]-propionic acid), was downloaded from the protein data bank (PDB ID: 3ET1). The docking was performed by MOE software (MOE2022.v11.18.1) following our previously reported protocol (Bogari et al., 2022).
2.10 Statistical analysis
Results are expressed as mean ± SE. Statistical analyses were done by GraphPad Prism software (V8, CA, USA). Difference among groups was detected using One-Way ANOVA followed by Tukey post hoc test. A p < 0.05 was considered statistically significant.
3 Results
3.1 Effect of BPEO on dexamethasone-induced liver weight and liver index changes
As represented in Fig. 1, dexamethasone administration caused a significant increase in liver weight (Fig. 1A) and liver index (Fig. 1B), compared to the control group (p < 0.05). Treatment with the low dose of BPEO resulted in a significant increase in the liver weight compared to the dexamethasone group (p < 0.05) (Fig. 1A). Neither metformin nor the high dose of BPEO affected liver weight and liver index (p ˃ 0.05) compared to the dexamethasone group.Effects of black pepper essential oil (BPEO, in a dose of 0.5 and 1 mL/kg) or metformin (Met, 50 mg/kg) on liver weight and liver index in rats. (A) Weight of liver, (B) Liver index (liver weight divided by body weight). Each value represents the mean of six samples ± SE. a,b Significantly different from control and dexamethasone (Dexa) at p < 0.05, respectively.
3.2 BPEO alleviates dexamethasone-induced elevation of liver injury markers
The injection of dexamethasone to the rats induced severe damage to the hepatic tissues, as indicated by notable elevations of serum aspartate aminotransferase (AST) and alanine transaminase (ALT) activities. Additionally, histopathological examination revealed detrimental changes in the liver tissues. As presented in Fig. 2, the enzyme activities of both AST and ALT in the dexamethasone group were remarkably higher than those in the normal control group. Interestingly, treatment with either BPEO or metformin significantly reduced the liver enzyme activities compared to the dexamethasone group, indicating the hepatoprotective effect of BPEO.Effects of black pepper oil (BPEO, in a dose of 0.5 and 1 mL/kg) or metformin (Met, 50 mg/kg) on serum liver function parameters. (A) ALT, (B) AST, n = 6, mean ± SE. a,b Significantly different from control and dexamethasone (Dexa) at p < 0.05, respectively.
3.3 BPEO ameliorates dexamethasone-induced hepatic structural changes
Histopathological examination of the liver tissues from the rats in the dexamethasone group revealed hepatocyte degeneration, vacuolation, and mononuclear cellular infiltration. Furthermore, the animals exhibited dilated and congested blood vessels (Fig. 3B). The isolated liver tissues from both the metformin and the high dose of BPEO-treated groups showed nearly normal liver histological structure (Fig. 3C &E). On the other hand, examination of the liver tissues isolated from the group treated with the low dose BPEO showed many degenerated and vacuolated hepatocytes with some dilated congested blood vessels (Fig. 3D).Histopathological changes recorded in the hepatic tissues from the different treatment groups. (A) A representative photomicrograph of liver tissue section from the normal control group showing normal histological structure with hepatic cords (HC) radiating from the central vein (CV), forming anastomosing plates separated by blood sinusoids (S). Hepatocytes appeared polyhedral with eosinophilic cytoplasm having central rounded and vesicular nuclei (arrow). Notice the portal tract (PT). (B) A representative photomicrograph of a liver section of an adult rat from the dexamethasone group, showing that hepatocytes appeared to be degenerated. The cells were highly vacuolated with dark small nuclei (black arrow). A mononuclear cellular infiltration (white arrow) was observed. Dilated congested blood vessel (Bv) was spotted. (C) A representative photomicrograph of a liver section of an adult albino rat from the metformin group showing that the liver tissue appeared nearly with normal histological structure. The hepatocytes had eosinophilic cytoplasm with central rounded and vesicular nuclei (arrow). (D) A representative photomicrograph of a liver section from the low-dose BPEO group, showing that many hepatocytes appeared to be degenerated. The cells were vacuolated (arrow). Dilated congested blood vessel (Bv) was observed. (E) A representative photomicrograph of a liver section from the high dose BPEO group showing that the liver tissue appeared nearly with normal histological structure. The hepatocytes had eosinophilic cytoplasm with central rounded and vesicular nuclei (arrow). (H&E 200x).
3.4 Effect of BPEO on PGC-1α expression in the hepatic tissue
As presented in Fig. 4, there was a distinguished elevation in the number of PGC-1α positive cells in the liver tissues of the rats which received dexamethasone compared to the control group. Interestingly, BPEO reversed, dose-dependently, this increase in PGC-1α positive cells, compared to the dexamethasone group. Like the pattern recorded in the previous result, both the high dose of BPEO and metformin exerted similar but more potent effects than BPEO low dose (p < 0.05).Immunoexpression of PGC-1 α in the liver tissues from the different treatment groups. (A) A representative photomicrograph of a liver section from the normal control group displaying a few hepatocytes with PGC-1 α positive immunoreaction (arrow). (B) A representative photomicrograph of a liver section from the dexamethasone group displaying numerous hepatocytes with PGC-1 α positive immunoreaction (arrow). (C) A representative photomicrograph of a liver section from the metformin group revealing a few hepatocytes with PGC-1 α positive immunoreaction (arrow). (D) A representative photomicrograph of a liver section from the low-dose BPEO group demonstrating some hepatocytes with PGC-1 α positive immunoreaction (arrow). (E) A representative photomicrograph of a liver section from the high dose BPEO group showing few hepatocytes with PGC-1 α positive immunoreaction (arrow). (Avidin-biotin peroxidase stain with Hx counter stain 400x, scale bar = 50 µm). (F) A bar graph representing the number of PGC-1 α positive cells in the different groups. Each value represents the mean ± SE, n = 10. a,b,c,d significantly different from control, dexamethasone (Dexa), metformin (Met) and high-dose BPEO at p < 0.05, respectively.
3.5 Effect of BPEO on PPAR-α expression in the hepatic tissue
As expected, dexamethasone decreased the expression of PPAR-α relative to the normal control group. While the low dose of BPEO failed to improve PPAR-α expression (p ˃ 0.05), both metformin and the high dose of BPEO were able to significantly increase the protein expression when compared to the dexamethasone group (Fig. 5, p < 0.05).Immunoexpression of PPAR-α in the liver tissues from the different treatment groups. (A) A representative photomicrograph of a liver section from the normal control group displaying many hepatocytes with PPAR-α positive immunoreaction (arrow). (B) A representative photomicrograph of a liver section from the dexamethasone group displaying a few hepatocytes with PPAR-α positive immunoreaction (arrow). (C) A representative photomicrograph of a liver section from the metformin group displaying many hepatocytes with PPAR-α positive immunoreaction (arrow). (D) A representative photomicrograph of a liver section from the low-dose BPEO group displaying some of the hepatocytes with PPAR-α positive immunoreaction (arrow). (E) A representative photomicrograph of a liver section from the high-dose BPEO group displaying many hepatocytes with PPAR-α positive immunoreaction (arrow). (Avidin-biotin peroxidase stain with Hx counter stain 400x, scale bar = 50 µm). (F) A bar graph reporting the number of PPAR-α positive cells in the various groups. Each value represents the mean ± SE, n = 10. a,b,c,d significantly different from control, dexamethasone (Dexa), metformin (Met) and high-dose BPEO at p < 0.05, respectively, detected using One-Way ANOVA followed by Tukey post hoc test.
3.6 Molecular docking results
To obtain more insights, at a molecular level, about the potential of BPEO constituents to activate the PPAR-α receptor, we docked the volatile compounds in BPEO into the ligand binding pocket of PPAR-α. It is well reported that PPAR-α full agonists afford some polar and non-polar interactions with specific amino acid residues in helix 12 (H12) in the ligand binding pocket, mainly Thr279, Ser280, Tyr314, Val332, Ile339, His440, and Tyr464, while partial agonists, on the other hand, do not afford direct interactions with these residues in H12 (dos Santos et al., 2015). Table 1 lists the docking scores of the most stable docking pose obtained from each compound. Self-docking of the co-crystallized agonist showed RMSD value below 2 (1.07) and afforded all the aforementioned characteristic interactions to PPAR-α full agonist, which validates our docking protocol. It showed additional nonpolar interactions with Phe273, Lue321, and Ile354 (Fig. 6). The previously reported PPAR-α agonist carvacrol (Hotta et al., 2010), was chosen as a reference natural compound in this docking study. It showed a binding score of −7.86 kcal/mol, and afforded polar interactions with Tyr314 and His440, Fig. 6.
Compound name
Docking score (kcal/mol)
δ-Cadinene
fail
β-Bisabolene
−8.35
(E)-Caryophyllene
−8.46
α-Muurolene
fail
α-Humulene
fail
β-Cubebene
fail
α-Cubebene
fail
β-Selinene
−8.72
(Z)-caryophyllene
−8.20
α-Copaene
−8.36
δ-Elemene
fail
p-Cymene
−7.07
allo-Ocimene
−7.15
Terpinolene
fail
γ-Terpinene
−7.20
α-Terpinene
fail
(E)-β-Ocimene
−7.12
α-Pinene
fail
α-Phellandrene
fail
Myrcene
−6.79
Limonene
−7.19
Sabinene
−7.13
α-Thujene
fail
β-Pinene
fail
Carvacrol
−7.86
Co-crystallized ligand
−12.89
2D-docking poses of the co-crystallized PPAR-α agonist (left), carvacrol (middle), and β-selinene docked in the agonist binding pocket of PPAR-α receptor.
Out of the docked 24 terpenoids previously identified in the BPEO, 12 compounds were able to fit in the ligand binding pocket of PPAR-α receptor with docking scores ranging from −8.72 to −6.79 kcal/mol, indicating a comparable binding affinity relative to the natural reference, carvacrol. The best binding affinity was shown by β-selinene which had a docking score of −8.72 kcal/mol, Table 1. However, the docked BPEO terpenoids did not afford any of the characteristic agonist interactions in the H12 helix. Rather, 11 compounds were able to show a nonpolar bi-H interaction with Phe273, while p-cymene afforded similar interaction with Ile354 at the agonist binding pocket of the receptor. The co-crystallized ligand afforded these two interactions as well when self-docked at the binding pocket of PPAR-α (Fig. 6). Noteworthy, Capelli et al. (2016), have reported a crucial role played by Phe273 supporting the activity of PPAR-α full agonists. The X-ray crystallographic studies showed that this amino acid changes its confirmation upon the binding of the agonist to the receptor, creating a new hydrophobic pocket between H3 and H11 to be occupied by the agonist (Capelli et al., 2016). The obtained docking results could possibly suggest that BPEO compounds are more likely to function as moderate or partial agonists on PPAR-α receptor rather than full agonists.
3.7 Correlation between ALT, liver injury marker, and both of PPAR and PGC-1α
In accordance with the previously mentioned results, Fig. 7 showed a strong negative correlation between the enzyme activity of the liver injury marker, ALT and PPAR-α (r = −0.6 at p < 0.01, Fig. 7A), whereas a strong positive correlation between the enzyme activity of ALT and PGC-1α (r= +0.7 at p < 0.001, Fig. 7B) was observed.Correlation analysis between ALT and the number of PPAR-α cells (A); Correlation analysis between ALT and the number of PGC-1α positive cells (B).
4 Discussion
This study aimed to investigate the hepatoprotective effects of black pepper essential oil (BPEO) on dexamethasone-induced hepatotoxicity. Dexamethasone is a type of steroid medication that has many applications in medicine, including the treatment of inflammation, autoimmune disorders, and certain types of cancer. However, it can cause side effects, some of which could be serious. As the liver has a central role in metabolizing and detoxifying drugs and xenobiotics, it is one of the most exposed organs to drug-induced toxicities (Sobeh et al., 2018).
According to multiple studies, dexamethasone has been found to increase the infiltration of inflammatory cells in the portal area (Kamanlı et al., 2004), leading to severe necrosis of hepatocytes (Safaei et al., 2012). It has also been demonstrated to decrease the transcriptional and proliferative ability of hepatocytes (Mikiewicz et al., 2017) and to cause deleterious abnormalities such as elevated osmotic pressure, electrolyte imbalances, and an impact on the Na+/K+ pump due to cell vacuolation and blebs formation (Abdelhalim and Jarrar, 2011). In this study, we demonstrated that the administration of dexamethasone to rats resulted in liver injury. However,pretreating the animals by BPEO mitigated this damage as evidenced by reducing mononuclear cellular infiltration, lowering AST and ALT levels, and alleviating hepatocyte degeneration and vacuolation. Concurrently, there was a decrease in the number of PGC-1α positive cells and an improvement in PPAR-α expression within the hepatocytes of rats subjected to dexamethasone injections.
Plant secondary metabolites have been the subject of extensive research due to their potential as novel pharmaceutical and therapeutic agents, offering possibilities for both treatment and chemoprotection (Van Wyk and Wink, 2018). Many of these plants were shown to exhibit hepatoprotective activities upon dexamethasone exposure in experimental animals including parsley, basil, and chicory (Soliman et al., 2016). The aqueous extracts from these plants were found to be effective in regulating the abnormal changes caused by dexamethasone, as seen by the decrease in cholesterol, glucose, TBARS levels and the significant improvement in the antioxidant and biochemical parameters such as AST, ALT, LDH, albumin, total protein, and liver GSH levels (Abou-Seif et al., 2019, Alsadee, 2021).
In the present study, we explored other manifestations of dexamethasone liver toxicity and investigated the possible mode of actions of BPEO in alleviating the adverse effects in liver. Noteworthy, the significant improvement in dexamethasone toxicity manifestations upon the administration of BPEO to rats is most likely to be due to its richness in volatile phytochemicals (26 compounds) with domination of potent ones such as E-caryophyllene, sabinene, β-pinene, α-pinene, α-copaene, α-thujene, and myrcene (Mahmoud et al., 2022a). For instance, α-pinene has been shown to exhibit hepatoprotective and nephroprotective effects by restoring the antioxidant levels and neutralizing intracellular ROS following CCl4-induced toxicity in Wistar Albino Rat (Udavant et al., 2023). Similarly, a recent study showed that β-myrcene pretreatment significantly attenuated acetaminophen-induced liver injury in mice, evidenced by reduced serum ALT and AST, myeloperoxidase activity, and nitric oxide levels. It demonstrated strong antioxidant activity, lowering malondialdehyde and ROS while enhancing reduced glutathione levels. Additionally, β-myrcene restored hepatic levels of superoxide dismutase, catalase, and oxidized glutathione (Cardia et al., 2022). These previous findings support the hepatoprotective effect of BPEO as it contains such compounds and corroborate the involvement of other mechanisms of actions in reversing xenobiotic-induced liver toxicity.
PGC-1α family of transcriptional coactivators functions as a molecular switch in a variety of metabolic pathways. The actions of PGC-1α are primarily boosted during fasting in the liver, where they are activated in various organs under circumstances of increasing energy demands. PGC-1α plays a significant role in several disorders as well as cancer conditions characterized by disrupted energy balance. Moreover, PGC-1α is mostly associated with gluconeogenesis and fatty acid β-oxidation in liver tissues. Previous research demonstrated that glucocorticoids could interact with PGC-1α to enhance hepatic gluconeogenesis and mitochondrial biogenesis (Menconi et al., 2010, Psarra and Sekeris, 2011). Additionally, in diabetic animal models, PGC-1α expression is greatly elevated in the liver, where it mediates gluconeogenesis and glucose production and causes fasting hyperglycemia (Herzig et al., 2001, Yoon et al., 2001). In this study, the hepatoprotective effects of BPEO were accompanied by a dose-dependent decrease in PGC-1α positive cells compared to the dexamethasone group with similar effects to metformin, the reference drug utilized for dexamethasone-induced liver injury.
PPAR-α is a nuclear hormone receptor that functions as a ligand-activated transcription factor to control genes’ expression involved in fatty acid consumption. It has a distinctive type II zinc finger DNA binding motif. The liver, brown adipose tissue, kidney, and muscle express high levels of PPAR-α receptors (Alaynick, 2008). In the liver, PPAR-α receptors have a crucial role in the regulation of lipids metabolism and homeostasis, where its disrupted expression was linked to liver steatosis, and steatohepatitis. These receptors are activated by fatty acids, their metabolites, and pharmacological activators such as fibrates (Forman et al., 1997). In turn, PPAR-α activates genes such as acyl-CoA oxidase and carnitine palmitoyl transferase 1 (CPT1) that are involved in fatty acid oxidation. The level of CPT1 largely controls fatty acid oxidation in the liver. Both PGC-1α and PPAR-α regulate the expression of CPT1 and thus fatty acid oxidation. PGC-1α is a crucial PPAR-α coactivator in tissues that undergo heavy oxidative metabolism and induce mitochondrial expansion. Interestingly, previous studies have shown that PPAR-α is activated in response to decline in blood glucose level, either by exercise or by fasting (Alaynick, 2008). Indeed, we previously showed that dexamethasone increased blood glucose levels and induced insulin resistance (Mahmoud et al. 2022b). In addition, previous research reported that the glucocorticoid-induced steatosis was linked to PPAR-α activity. Where PPAR-α levels were shown to be significantly lower in fatty livers compared to normal livers. This is most likely attributed to the activation of glucocorticoid receptor β that leads, in turn, to the inhibition of PPAR-α and augmenting inflammation (Du et al., 2015). This can be counteracted by PPAR-α agonists such as fibrates, which are effective in lowering plasma TG (triglycerides) and elevating HDL (high-density lipoprotein) in dyslipidemia patients and are commonly utilized in patients with insulin resistance (Lee et al., 2011, Mulvihill and Huff, 2012). Herein, our results confirmed that dexamethasone decreased the expression of PPAR-α. On the contrary, rats treated either with metformin or the high dose of BPEO revealed a significant increase in the expression of PPAR-α compared to the dexamethasone group.
The cross-connection between these biological parameters is obvious. We have observed a strong negative correlation between the liver injury biomarker, ALT, and PPAR-α, which confirms the role of PPAR-α in protecting hepatocytes against dexamethasone insult. Contrary to some other synthetic PPAR-α agonists that showed a positive correlation between PPAR-α activation and ALT-increased serum activity (Thulin et al., 2008), BPEO was able to improve the expression of PPAR-α receptors, and simultaneously decrease the ALT enzymatic activity in serum.
Our in-silico findings indicated that certain key constituents of BPEO exhibited a moderate binding capability, establishing amino acid interactions at the ligand binding site of PPAR-α receptors. This suggests a potential for moderate or partial agonistic activity of these compounds rather than full agonism, although confirmation awaits further validation through biological assays. Molecular docking results corroborate the effect of the high dose of BPEO in improving PPAR-α expression compared to the animal subjected dexamethasone only. This could open new venues for developing novel partial PPAR-α agonists of natural origin to limit the reported adverse effects of the currently used full agonists.
While BPEO exhibits promise in mitigating dexamethasone-induced liver damage, critical research gaps persist. Firstly, the precise molecular mechanisms underlying BPEO's hepatoprotective effects require further elucidation, necessitating in-depth exploration of the other involved pathways. Secondly, optimal dosage and administration protocols for BPEO need considering potential dose-dependent effects and long-term safety assessments. Additionally, transitioning findings from animal models to human applications is vital, emphasizing the need for human clinical trials to validate BPEO's hepatoprotective effects and evaluate its safety and efficacy in humans. Addressing these gaps will enhance our understanding of BPEO's therapeutic potential and open avenues for its clinical application in protecting the liver from dexamethasone-induced damage.
5 Conclusion
In the present study, we showed that black pepper essential oil (BPEO) reversed dexamethasone-induced liver injury. The investigated essential oil alleviated the increase in ALT and AST levels, monocytes infiltration, hepatocytes degeneration and vacuolation. We also demonstrated that these hepatoprotective effects could be attributed to the ability of BPEO to modulate cellular energy metabolism through hindering PGC-1α and potentiating PPAR-α activity. Nevertheless, the utilization of BPEO would require more studies to ensure its biosafety and health benefits using preclinical and clinical assays. Finally, this investigation strongly supports the utilization of BPEO in alleviating dexamethasone-induced unwanted effects on the liver and justifies additional studies on the oil’s other underpinning modes of action and the functional activity of its components.
6 Ethical committee
Animal protocols were approved by the Animal Care and Use Committee at the University of Zagazig to ensure humane treatment of the animals and avoid animal suffering (Approval No- ZU-IACUC/3/F/73 /2020).
Funding
This research received no external funding.
8 Contributions
M.F.M. and N.A. performed the pharmacological experiments. I.Ma. wrote the manuscript. A.M.E. and I.Mo. revised the manuscript. H.D. and M.A.O.A. performed in silico activities. R.A.H. performed histopathology experiments. M.F.M. and M.S. revised the manuscript and designed the study.
Declaration of competing interest
The authors declare that they have no known competing financial interests or personal relationships that could have appeared to influence the work reported in this paper.
References
- Gold nanoparticles induced cloudy swelling to hydropic degeneration, cytoplasmic hyaline vacuolation, polymorphism, binucleation, karyopyknosis, karyolysis, karyorrhexis and necrosis in the liver. Lipids Health Dis.. 2011;10(1):166.
- [Google Scholar]
- Thymus vulgaris extract modulates dexamethasone induced liver injury and restores the hepatic antioxidant redox system. Beni-Suef Univ. J. Basic Appl. Sci.. 2019;8(1):21.
- [Google Scholar]
- Nuclear receptors, mitochondria and lipid metabolism. Mitochondrion. 2008;8(4):329-337.
- [Google Scholar]
- Hepato-nephroprotective role of Lepidium sativum against oxidative stress induced by dexamethasone in rats. Indian J. Forensic Med. Toxicol.. 2021;15(1):2643-2653.
- [Google Scholar]
- Euclea divinorum hiern: chemical profiling of the leaf extract and its antioxidant activity in silico, in vitro and in Caenorhabditis elegans model. Metabolites. 2022;12(11):1031.
- [Google Scholar]
- Insulin action and signalling in fat and muscle from dexamethasone-treated rats. Arch. Biochem. Biophys.. 2008;474(1):91-101.
- [Google Scholar]
- Structural basis for PPAR partial or full activation revealed by a novel ligand binding mode. Sci. Rep.. 2016;6(1):1-12.
- [Google Scholar]
- Cardia, G. F. E., F. M. de Souza Silva-Comar, C. I. Bonetti, E. M. T. da Rocha, M. Zagoto, V. do Amaral, L. Bracht, S. E. Silva-Filho, C. A. Bersani-Amado and R. K. N. Cuman (2022). Hepatoprotective effect of β-myrcene pretreatment against acetaminophen-induced liver injury. Avicenna J. Phytomed. 12(4): 388.
- Antioxidant activity of syringic acid prevents oxidative stress in L-arginine–induced acute pancreatitis: an experimental study on rats. Int. Surg.. 2015;100(5):891-896.
- [Google Scholar]
- A review on therapeutic potential of Piper nigrum L. Black Pepper): the King of Spices. Med. Aromat. Plants. 2014;3(3):161.
- [Google Scholar]
- Different binding and recognition modes of GL479, a dual agonist of peroxisome proliferator-activated receptor α/γ. J. Struct. Biol.. 2015;191(3):332-340.
- [Google Scholar]
- Inhibition of dexamethasone-induced fatty liver development by reducing miR-17-5p levels. Mol. Ther.. 2015;23(7):1222-1233.
- [Google Scholar]
- Forman, B. M., J. Chen and R. M. Evans (1997). Hypolipidemic drugs, polyunsaturated fatty acids, and eicosanoids are ligands for peroxisome proliferator-activated receptors α and δ. Proc. Natl. Acad. Sci., 94(9): 4312-4317.
- Grape seed extract alleviates dexamethasone-induced hyperlipidemia, lipid peroxidation, and hematological alteration in rats. Indian J. Clin. Biochem.. 2019;34(2):213-218.
- [Google Scholar]
- CREB regulates hepatic gluconeogenesis through the coactivator PGC-1. Nature. 2001;413(6852):179-183.
- [Google Scholar]
- Carvacrol, a component of thyme oil, activates PPARα and γ and suppresses COX-2 expression [S] J. Lipid Res.. 2010;51(1):132-139.
- [Google Scholar]
- New insights into the novel anti-inflammatory mode of action of glucocorticoids. Immunopharmacol. Immunotoxicol.. 2020;42(2):59-73.
- [Google Scholar]
- Antioxidant, anti-inflammatory and antinociceptive properties of black pepper essential oil (Piper nigrum Linn) J. Essential Oil Bearing Plants. 2014;17(1):1-12.
- [Google Scholar]
- Jux, C., K. Leiber, U. Hügel, W. Blum, C. Ohlsson, G. n. Klaus and O. Mehls (1998). Dexamethasone impairs growth hormone (GH)-stimulated growth by suppression of local insulin-like growth factor (IGF)-I production and expression of GH- and IGF-I-receptor in cultured rat chondrocytes. Endocrinology 139(7): 3296-3305.
- Plasma lipid peroxidation and antioxidant levels in patients with rheumatoid arthritis. Cell Biochem. Funct.. 2004;22(1):53-57.
- [Google Scholar]
- Efficacy of fibrates for cardiovascular risk reduction in persons with atherogenic dyslipidemia: a meta-analysis. Atherosclerosis. 2011;217(2):492-498.
- [Google Scholar]
- Liu, G. Z., T. T. Hou, Y. Yuan, P. z. Hang, J. j. Zhao, L. Sun, G. q. Zhao, J. Zhao, J. m. Dong and X. b. Wang (2016). Fenofibrate inhibits atrial metabolic remodelling in atrial fibrillation through PPAR‐α/sirtuin 1/PGC‐1α pathway. Br. J. Pharmacol., 173(6): 1095-1109.
- Unraveling the phytochemistry, traditional uses, and biological and pharmacological activities of <i>Thymus algeriensis</i> boiss. & reut. Oxid. Med. Cell. Longev.. 2022;2022:6487430.
- [Google Scholar]
- Black pepper oil (Piper nigrum L.) mitigates dexamethasone induced pancreatic damage via modulation of oxidative and nitrosative stress. Biomed. Pharmacother.. 2022;153:113456
- [Google Scholar]
- Coriander oil reverses dexamethasone-induced insulin resistance in rats. Antioxidants. 2022;11(3):441.
- [Google Scholar]
- Menconi, M. J., Z. P. Arany, N. Alamdari, Z. Aversa, P. Gonnella, P. O'Neal, I. J. Smith, S. Tizio, P.-O. Hasselgren (2010). Sepsis and glucocorticoids downregulate the expression of the nuclear cofactor PGC-1β in skeletal muscle. Am. J. Physiol.-Endocrinol. Metab., 299(4): E533-E543.
- Influence of long-term, high-dose dexamethasone administration on proliferation and apoptosis in porcine hepatocytes. Res. Vet. Sci.. 2017;112:141-148.
- [Google Scholar]
- Antimicrobial and antiproliferative activities of black pepper (Piper nigrum L.) essential oil and oleoresin. J. Essential Oil Bearing Plants. 2017;20(3):779-790.
- [Google Scholar]
- Dexamethasone as an anti-cancer or hepatotoxic. Toxicol. Mech. Methods. 2023;33(2):161-171.
- [Google Scholar]
- Mulvihill, E.E., Huff, M.W., 2012. Protection from metabolic dysregulation, obesity, and atherosclerosis by citrus flavonoids: activation of hepatic PGC1α-mediated fatty acid oxidation. PPAR Res., 2012.
- Nutritional composition of some Piper nigrum (L.) accessions from Nigeria. Int. J. Med. Aromatic Plants. 2013;3(2):2249-4340.
- [Google Scholar]
- Glucocorticoids induce mitochondrial gene transcription in HepG2 cells: role of the mitochondrial glucocorticoid receptor. Biochim. Biophys. Acta (BBA)-Molecular Cell Res.. 2011;1813(10):1814-1821.
- [Google Scholar]
- Niacin ameliorates lipid disturbances due to glucocorticoid Administration in Rats, Iran. J. Basic Med. Sci.. 2012;15(4):997-1002.
- [Google Scholar]
- Phenolic compounds from Syzygium jambos (myrtaceae) exhibit distinct antioxidant and hepatoprotective activities in vivo. J. Funct. Foods. 2018;41:223-231.
- [Google Scholar]
- Hepatoprotective effects of parsley, basil, and chicory aqueous extracts against dexamethasone-induced in experimental rats. J. Intercult. Ethnopharmacol.. 2016;5(1):65-71.
- [Google Scholar]
- Genomic and nongenomic effects of glucocorticoids. Nat. Clin. Pract. Rheumatol.. 2008;4(10):525-533.
- [Google Scholar]
- Takooree, H., M. Z. Aumeeruddy, K. R. R. Rengasamy, K. N. Venugopala, R. Jeewon, G. Zengin and M. F. Mahomoodally (2019). A systematic review on black pepper (Piper nigrum L.): from folk uses to pharmacological applications. Crit. Rev. Food Sci. Nutrit. 59(sup1): S210-S243.
- PPARalpha regulates the hepatotoxic biomarker alanine aminotransferase (ALT1) gene expression in human hepatocytes. Toxicol. Appl. Pharmacol.. 2008;231(1):1-9.
- [Google Scholar]
- Evaluation of hepatoprotective and nephroprotective effect of Α-pinene on wistar albino rat. Biomed. Pharmacol. J.. 2023;16(1):103-112.
- [Google Scholar]
- Van Wyk, B.-E., Wink, M., 2018. Medicinal plants of the world, CABI.
- Dexamethasone sensitizes to ferroptosis by glucocorticoid receptor–induced dipeptidase-1 expression and glutathione depletio. Sci. Adv.. 2022;8(5):eabl8920.
- [Google Scholar]
- Proanthocyanidin alleviates liver ischemia/reperfusion injury by suppressing autophagy and apoptosis via the PPARα/PGC1α signaling pathway. J. Clin. Transl. Hepatol.. 2023;11(6):1329.
- [Google Scholar]
- Control of hepatic gluconeogenesis through the transcriptional coactivator PGC-1. Nature. 2001;413(6852):131-138.
- [Google Scholar]
- Antioxidant, hepatoprotective and antifungal activities of black pepper (Piper nigrum L.) essential oil. Food Chem.. 2021;346:128845
- [Google Scholar]
- Betulinic acid attenuates dexamethasone-induced oxidative damage through the JNK-P38 MAPK signaling pathway in mice. Biomed. Pharmacother.. 2018;103:499-508.
- [Google Scholar]