Translate this page into:
Camellia sinensis: Insights on its molecular mechanisms of action towards nutraceutical, anticancer potential and other therapeutic applications
⁎Corresponding authors. pracheta@banasthali.in (Pracheta Janmeda), javad.sharifirad@gmail.com (Javad Sharifi-Rad), asantini@unina.it (Antonello Santini), rafroman@unina.it (Raffaele Romano), calinadaniela@gmail.com (Daniela Calina), chocs@ha.org.hk (William C. Cho)
-
Received: ,
Accepted: ,
This article was originally published by Elsevier and was migrated to Scientific Scholar after the change of Publisher.
Peer review under responsibility of King Saud University.
Abstract
The Camellia sinensis plant provides a wide diversity of black, green, oolong, yellow, brick dark, and white tea. Tea is one of the majorly used beverages across the globe, succeeds only in the water for fitness and pleasure. Generally, green tea has been preferred more as compared to other teas due to its main constituent e.g. polyphenols which contribute to various health benefits. The aim of this updated and comprehensive review is to bring together the latest data on the phytochemistry and pharmacological properties of Camellia sinensis and to highlight the therapeutic prospects of the bioactive compounds in this plant so that the full medicinal potential of Camellia sinensis can be realised. A review of published studies on this topic was performed by searching PubMed/MedLine, Scopus, Google scholar, and Web of Science databases from 1999 to 2022. The results of the analysed studies showed that the main polyphenols of tea are the four prime flavonoids catechins: epigallocatechin gallate (EGCG), epicatechin gallate (ECG), epigallocatechin (EGC), and epicatechin (EC) along with the beneficial biological properties of tea for a broad heterogeneity of disorders, including anticancer, neuroprotective, antibacterial, antiviral, antifungal, antiobesity, antidiabetes and antiglaucoma activities. Poor absorption and low bioavailability of bioactive compounds from Camellia sinensis are limiting aspects of their therapeutic use. More human clinical studies and approaching the latest nanoformulation techniques in nanoparticles to transport the target phytochemical compounds to increase therapeutic efficacy are needed in the future.
Keywords
Camellia sinensis
Pharmacology
Cellular mechanisms
Molecular targets
Signaling pathways
Clinical applications
- MPTP
-
1-methyl-4-phenyl 1, 2,3,6-tetrahydropyridine
- AMPK
-
5’-adenylic acid-activated protein kinase
- 5’-DI1
-
5’-monodeiodinase type 1
- AIDS
-
acquired immunodeficiency syndrome
- AP-1
-
activator protein-1
- α-Syn
-
alpha-synuclein
- AE2
-
alveolar epithelial type II
- AD
-
Alzheimer’s disease
- AH
-
aqueous humour
- CVD
-
cardiovascular disease
- COMT
-
catechol-O-methyltransferase
- JNK
-
c-jun NH2-terminal kinase
- CLDN1
-
claudin 1
- cAMP
-
cyclic adenosine monophosphate
- COX-2
-
cyclooxygenase 2
- DEX
-
dexamethasone
- DDT
-
dichlorodiphenyltrichloroethane
- DHFR
-
dihydrofolate reductase
- EBOV
-
Ebola virus infection
- EF-2
-
elongation factor for protein translation
- EC
-
epicatechin
- ECG
-
epicatechin gallate
- EGFR
-
epidermal growth factor receptor
- EGC
-
epigallocatechin
- EGCG
-
epigallocatechin gallate
- EBV
-
Epstein-Barr virus infection
- ERK
-
extracellular signal-regulated kinase
- GTF
-
glucan devised from glucosyltransferases
- GTC
-
green tea catechin
- HNF4
-
hepatocyte nuclear factor
- HDAC
-
histone deacetylase
- HMVEC
-
human dermal microvascular endothelial
- HIV
-
human immunodeficiency virus
- GTPs
-
green tea polyphenols
- iNOS
-
inducible nitric oxide synthase
- IBD
-
inflammatory bowel disease
- IRS-1
-
insulin receptor substrate-1
- IGF-1R
-
insulin-like growth factor-1 receptor
- IFN
-
interfereon
- IL
-
interleukin
- IOP
-
intraocular pressure
- LOX
-
lipoxygenase
- LDL
-
low density lipoprotein
- LDL-R
-
low-density lipoprotein receptor
- MDA
-
malondialdehyde
- MAO-B
-
monoamine oxidase B
- MMP
-
matrix metalloproteinase
- MAPKs
-
mitogen-activated protein kinases
- NADH
-
nicotinamide adenine dinucleotide
- NPC1L1
-
Niemann-pickC1-like 1
- NK-κβ
-
nuclear factor-kB
- OCLN
-
occluding
- OS
-
oxidative stress
- PD
-
Parkinson’s disease
- PPAR-γ
-
peroxisome proliferator-activated receptor-γ
- PI3K
-
phosphatidylinositol 3-kinase
- PLCγ2
-
phosphoinositide-specific phospholipase Cγ2
- PPE
-
polyphenon E
- PIA
-
polysaccharide intercellular adhesion capsule
- POAG
-
primary open-angle glaucoma
- PGD2
-
prostaglandin D2
- PTP1B
-
protein tyrosine phosphatase 1B
- ROS
-
reactive oxygen species
- RTK
-
receptor tyrosine kinase
- GSH
-
reduced glutathione
- RGCs
-
retinal ganglion cells
- RT
-
reverse transcriptase
- RA
-
rheumatoid arthritis
- SR-BI
-
scavenger receptor type B class 1
- SWAT
-
subcutaneous white adipose tissue
- SNC
-
substantial pars compacta
- SOD
-
superoxide dismutase
- TPO
-
thyroid peroxidase
- TSH
-
thyrotropin
- T4
-
thyroxine
- TGF
-
transforming growth factor
- T3
-
triiodothyronine
- TNF
-
tumour necrosis factor
- T1DM
-
Type 1 Diabetes Mellitus
- T2DM
-
Type 2 Diabetes mellitus
- UTI
-
urinary tract infections
- VEGF
-
vascular endothelial growth factor
- VWAT
-
visceral white adipose tissue
Abbreviations
1 Introduction
Different types of teas are consumed regularly and are obtained from Camellia sinensis (L.) Kuntze, the main variety is C. sinensis var. assamica (J.W.Mast.) Kitam. Based on fermentation degree, teas are categorized into dark tea (post-fermented tea), black tea (fermented tea), oolong, yellow and white tea (partially fermented), and green tea (completely fermented). Among all, dark tea is unique as it requires microbial fermentation and its production is restricted to the areas of Japan and China (Zheng et al., 2015). Black and green teas are commonly consumed and therefore are more broadly investigated. Yellow tea is the least explored tea but now it’s gaining attention in western countries due to its good quality, refreshing taste, better flavour, and therapeutic benefits (Xu et al., 2018).
However, many of the health care benefits are related to the consumption of green tea since the starting of its history, research-based evaluations on this tea and its components have been ongoing for lesser than three decennaries (Cabrera et al., 2006). Various epidemiological investigations, as well as research in animal models, have established the protection that green tea and other teas can provide, against different types of cancers such as lung, prostate, skin, liver, and breast (Hayat et al., 2015). Besides its anticancer properties, epigallocatechin gallate (EGCG) has been documented to be hypocholesterolemic, anti-angiogenic, anti-thyroidal, anti-thrombotic, and anti-mutagenic. In the case of age-related pathologies, green tea and other teas have been determined to offer remarkable defence against Parkinson’s and Alzheimer’s disease (Chaturvedi et al., 2006, Khadrawy et al., 2017). Tea has also exhibited anti-diabetic effects in pre-clinical trials and encouraged the expenditure of energy. Other valuable credit includes anti-bacterial, anti-obesity, anti-fungal, antiphotoaging, anti-HIV, anti-viral, anti-inflammatory, and life-prolonging activities. Due to its greater antioxidant content, green tea is used as a nutritional supplement or nutraceutical aid, and as an element in anti-ageing, cosmetics, masks, and shampoos emulsions (Gopal et al., 2016). Although, the outcome of clinical and epidemiological investigations of the connection between the consumption of green tea and organism fitness is quite diverse. For example, divergent outcomes among human studies may rise relatively, from disregarding lifestyle, and socioeconomic factors, and by insufficient technique to describe the preparation and consumption of tea (Cabrera et al., 2006). Therefore, this paper aims to review the pharmacological properties, underlying the molecular mechanisms of action and the latest studies on the different types of teas and to examine their promising therapeutic potential.
2 Review methodology
This review is concentrated on the literature in the English language. The literature survey was carried out in databases such us PubMed/MedLine (1999–2022), NCBI (1999–2022), Google scholar (1999–2022) and BMC Complementary and Alternative Medicine (BMC, 1999–2021). The keywords utilized for the search were chosen from the following terms: polyphenols, white tea, black tea, green tea, catechin, health benefits, yellow tea, dark tea, oolong tea, anticancer, and diabetes. More than 200 articles on different forms of tea have been recognized by utilizing the search databases mentioned above. The scientific names of the plants were validated according to World Flora Online (WFO 2021) and the chemical formulas with ChemSpider and PubChem (PubChem 2022). The precise information about these articles is described below.
3 Phytochemical constituents
The processing, origin, taste, colour, and composition of different teas are discussed in Table 1 and Fig. 1 (Graham 1992, Belitz and Grosch 1997, Vinson 2000, Sano et al., 2001, Khokhar and Magnusdottir 2002, Chacko et al., 2010, Skotnicka et al., 2011, Dias et al., 2013, Zheng et al., 2015, Ng et al., 2018, Xu et al., 2018) and the structure of common chemical components that are responsible for the therapeutic value of teas is represented in Fig. 2 and Fig. 3.
Properties
Green tea
Black tea
Oolong tea
White tea
Yellow tea
Brick dark tea
Processing
Involves fixation, rolling & drying processes (Cabrera et al., 2006)
Involves a withering, rolling, fermentation & drying process (Sharma and Rao 2009)
Involves a withering, rocking, rolling & drying process (Chen et al., 2010)
Involves a withering & drying process (Hajiaghaalipour et al., 2015)
Involves fixation, rolling, yellowing & the process of drying (Zhang et al., 2019)
Involves fixation, post-fermentation, shaping & the process of drying (Zhang et al., 2019)
Origin
China (Sinija and Mishra 2008)
Asia and Europe (Skotnicka et al., 2011)
Fujian Province of China (Zheng et al., 2015)
Fujian Province of China (Ning et al., 2016)
China (Xu et al., 2018)
China & Japan (Zheng et al., 2015)
Taste
Bitter (Soni et al., 2015)
Distinct taste (Soni et al., 2015)
Bitter, sweet, brisk & mellow (Liu et al., 2018)
Mild taste (Hilal and Engelhardt 2007)
Caramel flavor (Guo et al., 2019)
Mellow taste (Zheng et al., 2015)
Colour
Green or yellow (Soni et al., 2015)
Red to black (Soni et al., 2015)
Appear red from the edges & green in the center (Chen et al., 2010)
Pale yellow colour (Hilal and Engelhardt 2007)
Yellow color (Xu et al., 2018)
Brick red to brown color (Zheng et al., 2015)
Composition of different forms of tea.
Chemical stuctures of the most representative aminoacids, lipids and steroids from Camellia sinensis (L.) Kuntze.
Chemical structures of carbohydrates and catechins from Camellia sinensis (L.) Kuntze.
4 Pharmacological properties
4.1 Anticancer effect
Cancer is a life-threatening disease with increasing incidences that have generated a sense of concern for the healthy lifestyle of the human being (Ianoși et al., 2019, Sharifi-Rad et al., 2020, Janmeda and Chaudhary 2021). Normal cells divide neatly and die when they are worn out or damaged, and new cells take their place. Cancer occurs when cells start to grow and divide uncontrollably (Buga et al., 2019, Georgiadis et al., 2020). Cancer cells continue to grow and produce new cells that destroy normal cells(Coêlho et al., 2022, Iqbal et al., 2022, Motyka et al., 2023).
Potential green tea catechins mechanisms of action against cancer:
-
Anti-oxidant and pro-oxidant activities
Reactive oxygen species (ROS) are unstable molecules with roles in intracellular processes(Salehi et al., 2019, Tsoukalas et al., 2019, Iordache et al., 2020, Sharifi-Rad et al., 2021a, Sharifi-Rad et al., 2021b). Excess accumulation of ROS is associated with increased oxidative stress and the development of many chronic diseases such as diabetes, cardiovascular disease, neurodegenerative diseases and cancer(Calina et al., 2020, Calina et al., 2020, Islam et al., 2020, Islam et al., 2021, Salehi et al., 2021). Catechins provide anti-oxidant properties by counteracting the unstable free reactive molecules. Among green tea catechins (GTCs), epicatechin gallate (ECG) has the highest potential as a radical scavenger, followed by EGCG, epigallocatechin (EGC), and epicatechin (EC). The antioxidant activity is because of the occurrence of phenolic groups with sensitivity to oxidation which results in the production of quinine. The activity is further intensified due to the presence of a trihydroxy structure in the d-ring of the molecule (Bernatoniene and Kopustinskiene 2018).
Green tea polyphenols can produce ROS which are required for the initiation of the apoptosis process and the restriction of the growth of the tumour cell. Both pro- and anti-oxidant properties of GTCs are found to be the major ones for the control of cancer (Shirakami and Shimizu 2018).
-
Apoptosis induction and cell cycle arrest induction
Apoptosis is an orderly process during which the cell's genome is broken down, the cell is fragmented into smaller pieces and the remains are consumed by nearby cells (phagocytes) that clean up the cell fragments (Sharifi-Rad et al., 2020, Scheau et al., 2021, Taheri et al., 2022). Apoptosis leads to programmed cell death to remove unwanted or abnormal cells from the body and maintain a stable internal environment(Sharifi-Rad et al., 2021c, Dhyani et al., 2022, Semwal et al., 2022). Treatment with EGCG-induced programmed cell death due to the formation of ROS and activation of caspase-9 and −3, majorly to cell-cycle arrest at the G1 phase via regulating the expression of p21, cdk4, and cyclin D1 (Shirakami and Shimizu 2018).
-
Nuclear factor-κB (NK-κB) and activator protein-1 (AP-1) inhibition
NK-κβ is recognized to play a pivotal part in restricting the process of programmed cell death in cancerous cells, leading to tumorigenesis (Jain et al., 2021, Javed et al., 2022, Quetglas-Llabrés et al., 2022, Varela et al., 2022). Treatment with EGCG restrained the activation of NF-κB in the lung, breast, and human neck and head squamous carcinoma. When NF-κB is turned on, it is moved into the nucleus and directs the multiple expression of genes related to tumorigenesis and its progression, further including inflammation, chemo-resistance, radio-resistance, metastasis, invasion, proliferation, and cellular transformation. Another factor is AP-1 which maintains the levels of gene expression associated with cellular proliferation and apoptosis(Pezzani et al., 2023). It is evaluated that AP-1 encourages multiplications via down-regulating the tumour suppressor genes p21 and p53 and up-regulating the expression of the cyclin D2 gene. EGCG was outlined to suppress the activation of AP-1 and transformation of cells and to restrict Ras-activated AP-1 in the epidermal cell line of a mouse (Shirakami and Shimizu 2018).
-
Receptor tyrosine kinase (RTK) pathway inhibition
RTKs plays an important role in the proliferation and apoptosis of cell and are proposed to be targeted by GTCs for the cure of cancer. RTKs and their downstream signals, such as phosphatidylinositol 3-kinase (PI3K)/ Akt signaling and the Ras/extracellular signal-regulated kinase (ERK) pathways, maintain the expression levels of different target genes related to apoptosis and proliferation. Irregularities in a few RTKs, such as vascular endothelial growth factor receptor 2 (VEGF-R2), insulin-like growth factor-1 receptor (IGF-1R), and epidermal growth factor receptor (EGFR) are deeply linked with the properties of malignancy (Cheng et al., 2020). Treatment with EGCG repressed the production of VEGF via restricting the activation of NF-κB, activator transcription STAT-3 and signal transducer in human breast and HNSCC (Shirakami and Shimizu 2018).
Anti-angiogenesis
Angiogenesis involves the growth of new capillaries from pre-existing ones, which is necessitate in various processes such as metastasis, tumour growth, and wound healing(Pezzani et al., 2023, Prasher et al., 2023). Green tea extract may restrict the progression of breast tumours by sealing angiogenesis (Jung and Ellis 2001). Polyphenon E (PPE), an isolated extract of green tea hinders vascular endothelial (VEGF) and matrix metalloproteinase 9 (MMP9) expression and human dermal microvascular endothelial (HMVEC) cell migration in the case of MDA-MB231 breast cancer. Additionally, PPE discouraged VEGF-directed angiogenesis in vivo. Further, it restricted the signal transducers and STAT signaling by restraining interferon (IFN)-γ-directed gene transcription with the help of IFN-γ-activating sequence (GAS) elements and downstream activation of STAT3 by restricting STAT3 and STAT1 dimerization in MDA-MB231 cells. A momentary expression of fundamental active STAT3 considerably limits the inhibitory outcome of PPE on the migration of cells and the expression of MMP9 and VEGF. These results designate that the extract of green tea partly inhibits angiogenesis via the disorganization of STAT3-directed gene transcription, involving VEGF (Leong et al., 2009). EGCG can restrict the angiogenesis of experimental endometriosis in the model of mice, but it encounters poor bioavailability. EGCG prodrug (EGCG octaacetate) is employed to improve the bioavailability and stability of EGCG in vivo. Pro-EGCG markedly limited the growth and angiogenesis in mice and leads to the development of higher potency, superior bio-availability, and greater anti-angiogenesis and anti-oxidation capacities as compared to EGCG (Wang et al., 2013).
Table 2, Table 3 and Fig. 4 summarize the molecular mechanisms of anticancer activities of green tea. Abbreviations and symbols: ↑increase, ↓decrease, mg/kg: milligram per kilogram, i.p.: intraperitoneally, µg/ml: microgram per milliliter, A/J mice: the inbred strain of mice, AH109A cells: cellosaurus cell line; BW: Body weight, i.g.: intragastarically. Abbreviations and symbols: ↓ (decrease), ↑ (increase), histone deacetylase (HDAC), lipoxygenase (LOX), matrix metalloproteinase (MMP-9), nuclear factor kappa light chain enhancer of activated B cells (NF-κB), protein tyrosine phosphatase 1B (PTP1B), peroxisome proliferator-activated receptor gamma (PPAR-γ), protein tyrosine phosphatase 1B (PTP1B), melanocyte inducing transcription factor (MITF), protein tyrosine phosphatases (PTP1B), Phosphorylated AKT(pAKT), Programmed death-ligand 1(PD-L1), Deoxyribonucleic Acid (DNA), N-nitrosodiethylamine (NDEA), epigallocatechin gallate (EGCG).
Tea components
Pharmacological properties
Model
Dose/ routes
Results/Potential mechanisms
References
EGCG/green tea extract
Neuroprotective
mice/ in vivo
2–10 mg/kg
↑neuroprotection against N-methyl-4-phenyl-1,2,3,6-tetrahydropyridine-induced neurodegeneration
(Levites et al., 2001)
0.5–1 mg/kg
↓body weight
orally
↓white adipose tissue weight
Antiobesity
mice/ in vivo
50 mg/kg, i.p.
↓mitochondrial DNA
↓TNF, ↓IL-6, ↓ IL-8(Okuda et al., 2014)
Cardioprotective
rats/ in vivo
10 mg/kg, i.p.
↓ventricular arrhythmia
(Shi et al., 2017)
Anti- Pulmonary fibrosis
rats/ in vivo
25 mg/kg, i.p.
↓ irradiation-directed pulmonary fibrosis
(You et al., 2014)
Caffeine/Polyphenon E (PPE)
Neuroprotective
rats/ in vivo
30 mg/kg, i.p
↑neuroprotection against rotenone-induced Parkinson’s disease
(Khadrawy et al., 2017)
Catechin
Anti-angiogenic
mice/in vivo
50 mg/kg, i.p.
↓initiation of endometriosis
(Xu et al., 2009)
↑anti-angiogenic effect
Black tea extract/water extract of black tea/polyphenols
Neuroprotective
rats/in vivo
1.50 % orally
↑neuroprotection against 6-hydroxydopamine lesioned-directed Parkinson’s disease
(Chaturvedi et al., 2006)
Antiobesity
rats/in vivo
a high-fat rich diet with black tea polyphenols
↑the fatty acid level in faeces, regulates the fat metabolism
(Islam 2011)
Antidiabetic
mice/in vivo
0.01 %, orally
↓ level of blood glucose
(Tang et al., 2013)
Anti-inflammatory
rats/in vivo
0.5–1.0 mg/kg bw orally
↑ anti-inflammatory activity against adjuvant-directed arthritis
(Ramadan et al., 2017)
Pulmonary fibrosis
mice/in vivo
25–50 mg/kg bw orally
↓the effect of fibrotic markers
(Chakraborty et al., 2019)
Antifungal
Candida species/in vitro
MIC = 6.25 mg/ml
↑antifungal activity
(Sitheeque et al., 2009)
Cardioprotective
Humans
150 mg, twice daily, orally
↑ protection to blood vessels
↑increased flow of angiogenic cells(Tang et al., 2019)
Antibacterial
Humans with Helicobacter pylori infection
≥ 1 day/week, orally
↓risk of bacterial infection
(Boyanova et al., 2015)
Oolong tea
Neuroprotective
neuro-2a, HT22 cells/in vitro
IC50 = 5,10, 25 µg/ml
Prove to be a potential one for the cure of neurodegenerative diseases
(Zhang et al., 2020)
Antiobesity
mice/ in vivo
high-fat rich diet with 5 % oolong leaf powder; orally
↓fatty liver
↓obesity(Han et al., 1999)
Antidiabetic
diabetic patients
1500 ml daily, orally
antihyperglycemic effect
(Hosoda et al., 2003)
White tea
Neuroprotective
striatal cells/in vitro
IC50 = 25, 100,200 µg/mL
protect striatal cells line against oxidative stress-directed cell death
(Almajano et al., 2011)
Antiobesity
mice/in vivo
0.5 %, orally
↓oxidative stress,
↓triacylglycerol
↓diabetes-related abnormalities of streptozotocin-induced diabetes(Teixeira et al., 2012)
(Islam 2011)
Antidiabetic
rats/in vivo
0.5 %, i.p.
Cardioprotective
rats/in vivo
1 g/100 ml
↓cardiac glycolytic activity
↑antioxidant activity(Alves et al., 2015)
Antimicrobial
Pseudomonas aeruginosa; Staphylococcus aureus; Escherichia coli
Disc agar diffusion assay
inhibit P. aeruginosa, E.coli, S. aureus
(Enzveiler et al., 2011)
Yellow tea
Hypoglycemic
mice/in vivo
50 mg/kg bw
↑epimerized catechin level
↓alpha-glucosidase(Zhou et al., 2018)
Lipids reducing
mice/in vivo
0.5 %; orally
↑ efficacy in lowering lipid profile
(Xu et al., 2018)
Dark tea
Lipids reducing
rats/in vivo
100, 300, 500 mg/kg bw
↑antioxidant enzymes
(Mao et al., 2018)
↓absorption of lipids
↓hyperlipidemia
Form of tea
Cancer type
Model
Dose/IC50
Anticancer activity
Ref.
Polyphenon E (PPE)/ Caffeine/ Green tea
Adenocarcinoma
mice
Dose = 0.5 %-0.44 %
↓lung adenomas progression to adenocarcinoma
(Lu et al., 2006)
in vivo
orally
Green tea polyphenols
Malignant melanoma
melanoma cells
IC50 = 30 and 60 µg/mL
↓progression of malignant melanoma
(Wu et al., 2021)
in vitro
↓circMITF/miR-30e-3p/HDAC2
EGCG
Breast cancer
4 T1 cells
IC50 = 50 µg/mL
↓ growth of tumor
(Jang et al., 2013)
in vitro
↓tumor-related M2 polarization
↓macrophage infiltration
Cholangiocarcinoma
CCA cells (HuCC-T1)
IC50 = 5 µg/mL
↓growth of cancer cells
(Kwak et al., 2017)
in vitro
↓migration, ↓ invasion
Bladder cancer
SW780 cells
IC50 = 70.2 µM at 24 h
↓migration, ↓proliferation
(Luo et al., 2017)
in vitro
IC50 = 113.6 µM at 48 h
↓MMP-9, ↓NF-kB
Lung cancer
H1299 cells
IC50 = 10 and 50 µM
↓tumourigenicity
(Namiki et al., 2020)
in vitro
↓stemness directed by AXL receptor tyrosine kinase
Colorectal cancer
Rats
Dose = 50,100,200 mg/kg
↓ colorectal cancer induced by dimethylhydrazine
(Wang et al., 2020)
in vivo
orally
Green tea
Cervical adenocarcinoma
HeLa cells
IC50 = 100 µg/ mL
↓ cancer cells growth
(Krstic et al., 2015)
in vitro
Green tea catechins
Breast cancer
MCF-7 cells
IC50 = 500, 100, 50, 10, 1 µM/mL
↓ PTP1B
(Kuban-Jankowska et al., 2020)
in vitro
↓viability of cancer cells
Lung cancer
A549 lcells
IC50 = 600 µmol
↓pAKT
(Sun et al., 2020)
in vitro
↓cyclin E1
↑ cyclin kinase inhibitior p21
Lung cancer
mice
Dose = 0.3 % orally
↓ PD-L1
(Rawangkan et al., 2018)
in vivo
↓growth of tumor
Green tea
Lung cancer
murine model
Dose = 1 % orally
chemopreventive effect against benzo(a)pyrene-induced lung cancer at low concentration
(Farooq and Sehgal 2021)
in vivo
Catechin hydrate
Cervical cancer
SiHa cells
IC50 = 196.07 µM/mL
↓proliferation
(Al-Hazzani and Alshatwi 2011)
in vitro
↑apoptosis
Aqueous extract of oolong tea
Hepatic cancer
AH109A cells
concentration = 0.04 %
↓invasion
(Zhang et al., 1999)
in vitro
↓proliferation
Breast cancer
MDA-MB-468,
concentration = 1 %
↑ damage to DNA
(Shi et al., 2018)
MDA-MB-436,
↓tumorigenesis
MDA-MB-231,
↓growth of cancer cells
SKBR3, T47D,
MCF-7 cells/in vitro
Yellow tea extract
Breast cancer
MDA-MB-231 cells
IC50 = 100–800 µM/ml
↑anticancer activity
(Lin et al., 2013)
in vitro
↓ growth of cancer cells
Hepatic cancer
HepG2 cells
IC50 = 0, 0.125, 0.25, 0.5, 1 mg/mL
↓AKT, ↓PI3K phosphorylation
(An et al., 2019)
in vitro
↑ Bax/Bcl-2
Liver cancer
Rats
Dose = 10 g/kg feed
↑ protection against NDEA-directed liver cancer
(Kujawska et al., 2016)
in vivo
orally
White tea extract
Colon cancer
HT-29 cells
IC50 = 0–500 µg/mL
↓proliferation, ↑caspases
(Hajiaghaalipour et al., 2015)
in vitro
protect the normal cell against oxidative damages
Lung cancer
A549, H521 cells
IC50 = 3.5, 5, 7, 10 µg/mL
↑apoptosis
(Mao et al., 2010)
in vitro
↑PPAR-γ, ↑15-LOX
Black tea extract
Colon and breast cancer
HT-29, MCF-7 cells
IC50 = 5 µg/mL
↑apoptosis, ↑oxidative damage
(Koňariková et al., 2015)
in vitro
↑DNA strand break
Black tea thearubigins and theaflavins
Colon and lung cancer
HT 460, HCT116 cells
IC50 = 50,100,150,200,250 µg/mL
↓proliferation
(Imran et al., 2019)
in vitro
↑cell cycle arrest, ↑apoptosis
Illustrative scheme with the most representative anticancer molecular mechanisms of bioactive compounds from tea. Abbreviations and symbols: ↑increase, ↓decrease, ⊥ inhibition, vascular endothelial growth factor (VEGF), lipoxygenase (LOX), matrix metalloproteinase 9 (MMP9), activator transcription (STAT-3), human dermal microvascular endothelial (HMVEC), epigallocatechin gallate (EGCG).
4.2 Neuroprotective effect
Different components of tea are reported to be used in the treatment of neurodegenerative diseases (Fig. 5). The neuroprotective effect of the tea component along with their mechanism is discussed below.The most important and representative pharmacological effects of bioactive compounds from Camellia sinensis (L.) Kuntze. Abbreviations and symbols: ↑increased, ↓decreased, DNA (Deoxyribonucleic acid), MAO-B (monoamine oxidase B), TNF-α (tumour necrosis factor alfa), IL-1β (interleukin 1beta), ROS (reactive oxidative species), α-Syn (alfa synuclein), DHFR, (dihydrofolate reductase), RT (reverse transcriptase), HIV (human immunodeficiency virus), AD (Alzheimer disease), PD (Parkinson disease).
4.2.1 Alzheimer’s disease (AD)
AD is reported to be the most frequent acute disorder globally. Its occurrence is steadily hiking due to an ageing population vital statistics (Sharifi-Rad et al., 2022). AD is determined by a deduction in thinking and memory, as well as due to some behavioural issues (Calina et al., 2020, Tsoukalas et al., 2021). Some authors explained that the tiny binding monomers of beta-amyloid result in the formation of beta-amyloid oligomers. With time, these oligomers can hold together and direct the generation of toxic beta-amyloid plaques. Researchers found that EGCG block the emergence of beta-amyloid plaques which is a hallmark of AD, by disrupting the outcome of beta-amyloid oligomers (Sharifi-Rad et al., 2022).
4.2.2 Parkinson’s disease (PD)
PD is distinguished by the deterioration of dopaminergic neurons in the pars compact of the substantia nigra of the basal ganglia (Alexander 2004, Islam et al., 2021). The exact source of this disease is not known but it can be stimulated by the mutations in genes and revelation to environmental risk factors such as 1-methyl-4-phenyl 1, 2,3,6-tetrahydropyridine (MPTP), which is an agent able to produce the symptoms of PD in individuals and pesticides like dichlorodiphenyltrichloroethane (DDT) or rotenone persuade PD-like symptoms, and irreparable decaying of dopaminergic neurons (Siokas et al., 2021). The inflammation-raised concentration of iron in substantial pars compacta (SNC), deduction in glutathione (antioxidant enzyme), and oxidative stress (OS) are some chief factors in the pathogenesis of PD. Further prompting factors include aggregation of alpha-synuclein (α-Syn), deduction in the activity of mitochondrial complex I, the liberation of cytochrome C, and lowering of reduced glutathione (GSH)(Siokas et al., 2021). The patients with PD have also displayed instability in the electron transport chain that directs the accretion of ROS, the unstable molecules responsible for lipid peroxidation and cellular damage (Jurado-Coronel et al., 2016).
Potential mechanisms of green tea to minimize PD:
-
Inhibitor of monoamine oxidase B (MAO-B): MAO is a mitochondrial enzyme linked to the oxidative deamination of dopamine; therefore, inhibitors of MAO most probably could be a valuable agent to cure diseases related to the malfunction of dopamine such as PD. It has been reported that catechin possesses MOA-B inhibitory activity that is found to be capable one to treat PD (Bortolato et al., 2008, Jurado-Coronel et al., 2016).
-
Activation of potential signaling pathways: green tea polyphenols can exhibit constructive action with the help of several mechanisms such as:
-depletion in the delivery of cytokines such as tumour necrosis factor TNF-α or interleukin IL-1β, the renewing of signaling pathways that minimize the mass production of ROS and inducible nitric oxide synthase (iNOS) such as nicotinamide adenine dinucleotide (NADH) oxidase activation, and the restriction in the translocation of NF-κB to the nucleus, a major event triggered the inflammation process in the brain (Yahfoufi et al., 2018).
-protection of dopaminergic neuronal, provided by EGCG may depend upon the mitogen-activated protein kinases (MAPKs), PI3K/Akt, and activation of protein kinase C (Zhao et al., 2018). EGCG protects neurons by inhibiting the pro-apoptotic gene expression, such as TNF-α related apoptotic ligand TRAIL, Fas ligand, cell cycle inhibitors such as Gadd45, Bax, and Bad or by restricting the aggregation of α-Syn and iron in MPTP induced model of mice, probably due to its iron-chelating and antioxidant properties.
- the iron-chelating activities are attributed to the chelating groups such as the presence of the gallate group and 3,4-dihydroxyl group in the B ring of EGCG, which counteract the ferric iron to result in redox-inactive iron, and may safeguard the neuronal cells from the damages caused by OS.
- EGCG restricts the iNOS expression, avoid peroxidation of lipid, inhibit catechol-O-methyltransferase (COMT), thus reducing the metabolism of dopamine residue, and is demonstrated to be a determent of microglial activation and an anti-apoptotic agent (Jurado-Coronel et al., 2016).
4.3 Antiobesity and anti-dyslipidemic effects
Obesity is a metabolic and nutritional problem, which has a clinical presentation of fat cell hypertrophy and hyperplasia (Salehi et al., 2020). Fat cell hyperplasia results due to the differentiation and proliferation of preadipocytes which could direct the uncontrolled deposition of lipids in adipose tissue, and mainly impact the composition of the body and the mortality of adiposity and associated disorders. Whereas fat cell hypertrophy often occurs when the intake of energy exceeds the expenditure of energy (Huang et al., 2014, Muir et al., 2016).
After consumption, GTCs intrude with the mechanism of energy metabolism and absorption in organisms:
-
hindrance in the multiplication of preadipocytes,
-
affects the programmed cell death of the pre and mature adipocytes,
-
restrict the preadipocyte differentiation and the mature adipocyte adipogenesis,
-
restrain the function of the digestive enzymes of the gastrointestinal tract, the luminal emulsification, the solubilization of micelles and the hydrolysis of lipids
-
obstruct the processing and intracellular uptake of lipids, secretion and assembly of chylomicrons in enterocytes,
-
raises the faecal energy excretion,
-
stimulate the oxidation of fatty acids
-
increase the uptake of glucose by skeletal muscles
-
activates the expression of the gene responsible for the oxidation of fatty acid and lipolysis-associated genes in adipose tissue
-
repress the intake of glucose and the expression of genes related to fat synthesis (Huang et al., 2014).
Supplementation of GTC remarkably reported increased liver and body weights and the raised level of liver triglyceride and serum. Meantime, GTCs raised the level of peroxisome proliferator-activated receptor-γ (PPAR-γ) in subcutaneous white adipose tissue (SWAT) and reduced the level of PPAR-γ in visceral white adipose tissue (VWAT). Additionally, the treatment with GTC up-regulated the PPAR-δ level in brown adipose tissue, VWAT, and SWAT and encouraged the gene expression for the oxidation of fatty acid in brown adipose tissue. These results determine that GTC provides the anti-obesity effect by altering the signaling pathway of PPAR (Yan et al., 2013). Babu and Liu (2008) explained that cardiovascular disease (CVD) is a foremost cause of disability, fatality, and morbidity across the globe. GTC or green tea can suppress coronary heart disease and atherosclerosis through their low-density lipoprotein-cholesterol-decreasing activity. Zheng et al., (2011) revealed that catechin with gallate moiety was determined to interfere with the system of biliary micelle in the intestine by making insoluble precipitates of cholesterol and rising the cholesterol fecal excretion. This noticeable lowering in the absorption of cholesterol and depletion in the concentration of cholesterol in the liver leads to a promotion of low-density lipoprotein (LDL)-receptor activity and expression. This receptor is available on the uppermost side in most of the cells, particularly in hepatic cells, it can eliminate cholesterol-containing low-density lipoproteins from the circulation.
Recently, in vitro study has reported that GTC is a selective and strong inhibitor of a rate-limiting enzyme i.e. squalene epoxidase in cholesterol biosynthesis. Green tea remarkably restricts the concentration of LDL and serum total cholesterol, and these changes are not affected by the study quality, status of individual health, dose of treatment, duration of the study, and intervention type (Singh et al., 2009).
4.4 Antidiabetes effect
Diabetes mellitus is a chronic non-communicable disease that occurs when the pancreas can no longer produce insulin (or no insulin at all), or when the body can no longer use insulin properly. Additionally, it also resulted due to various impairments like fat, carbohydrate and protein metabolism by either inadequate quantity of insulin production or decreased sensitivity of target tissue to insulin (Painuli et al., 2022, Quispe et al., 2022). Type 1 Diabetes Mellitus (T1DM) include impaired β-cells of the pancreas and decreasing or no production of insulin whereas Type 2 Diabetes mellitus (T2DM) is determined by the resistance of target tissue to insulin and the relative scarcity of insulin produced by the islet of β-cells (Meng et al., 2019). Meng et al., (2019) reported that in T1DM, EGCG could preserve pancreatic β-cells function by restricting ROS and inflammatory factors in vitro or by down-regulating the generation of iNOS. Additionally, green tea was also able to lower the sugar level of blood with the help of β-cells to secrete more amount of insulin in the case of diabetic mice.
In T2DM, EGCG could trigger the 5′-adenylic acid-activated protein kinase (AMPK) pathway to surpass the insulin stress signaling pathway closure, resulting in the insulin receptor substrate-1 (IRS-1) phosphorylation. It could surpass the sensitivity of insulin by upregulating the level of insulin signaling proteins in insulin-resistant rats. EGCG was able to improve insulin resistance by counteracting ROS, which was capable to stop insulin signal transduction and inhibit the binding of IRS-1 to the receptor of insulin by reducing the TNF-α-directed c-jun NH2-terminal kinase (JNK) phosphorylation.
EGCG can play an insulin-like role and down-regulate the expression of protein and gene of hepatocyte nuclear factor (HNF4), a major transcription factor controlling the enzymes of gluconeogenesis, such as glucose-6-phosphatase and phosphoenolpyruvate carboxykinase, respectively. Moreover, catechin could assist the differentiation of adipocytes and raises insulin sensitivity by majorly triggering the PPAR-γ (Boucher et al., 2014).
4.5 Effect on thyroid disorders
The thyroid gland is present in the neck and directs the production of thyroid hormones. An enlarged thyroid gland (goitre) leads to the production of just enough, too little, & too much thyroid hormone (Calina et al., 2019). This improper production affects the rate of metabolism of the body (Haugen et al., 2013). The extract of green tea resulted from hypothyroidism at a higher dosage in the model of rats by changing the functional and morphological aspects of the thyroid. Furthermore, commercial catechins have showcased a promising effect in inducing hypothyroidism which proved that the efficacy of GTE may be due to the goitrogenic or antithyroid properties of catechins that exist in tea (Chandra and De 2010). Chandra and De (2010) revealed that oral consumption of green tea at the concentration of 2.5 % and 5.0 % for about 30 days altered the histology and morphology. There are significant restricted activities of 5′-monodeiodinase type 1 (5′-DI1) and thyroid peroxidase (TPO) with upraised K+, Na + ATPase and resultant dropping in serum triiodothyronine (T3), thyroxine (T4), and rise in serum thyrotropin (TSH) level which initiates a state of total biochemical hypothyroidism. All these results suggested that GTC has beneficial effects as adjuvant treatment in hypothyroidism.
4.6 Anti-inflammatory effect
Neutrophils play a crucial part in inflammation and host defence but later may activate and assist the pathogenesis of a wide range of chronic and acute diseases (Calina et al., 2020, Islam et al., 2020, Kostoff et al., 2020). Green tea has been proven to employ anti-inflammatory activity via an unspecified molecular process. GTC, EGCG, actively restrained neutrophil elastase. Furthermore, EGCG in micromolar concentration represses the activity of ROS and restricted the process of apoptosis of activated neutrophils, and hinders chemokine-directed neutrophil chemotaxis in vitro. Both green tea extract and EGCG obstruct neutrophil-directed angiogenesis in an angiogenesis model, and oral application of green tea extract improves the resolution in a pulmonary inflammation model, thus remarkably suppressing the consequent fibrosis. These outcomes furnished molecular and cellular insights into the affirmed therapeutic properties of green tea and specified that EGCG is a powerful anti-inflammatory molecule with a curative ability (Donà et al., 2003).
Rheumatoid arthritis (RA) is a persistent inflammatory disease determined by the triggering of synovial tissue that lines the joint capsule, which implicates in the invasion of the bone and cartilage distinguished the advanced joint dysfunction (Ahmed 2010, Mititelu et al., 2020). Haqqi et al., (1999) suggested that green tea polyphenols (GTPs) prosperous in antioxidants can minimize the reoccurrence of pathogenic Th1-type cells and related pathogenic CII-specific IgG2a antibodies in the diseased joints. These affected joints also had lesser concentrations of inflammatory mediators (cyclooxygenase 2 (COX-2) and TNF-α) and cytokines (Rogoveanu et al., 2018). This data revealed that the GTPs decrease the severity and incidence of collagen-directed arthritis in the case of DBAy1 mice.
Pulmonary fibrosis is represented by the composite process of inflammation that results in the uncontrolled proliferation of fibroblast and escalating sedimentation of connective tissue in the parenchymatous cells of the lungs (El-Safti et al., 2014). There are different types of pulmonary fibrosis, but the major one is the “idiopathic” form. You et al., (2014) reported that the therapy with EGCG, not dexamethasone (DEX) diminished the rate of mortality and index scores of the lung, surpassed histological alterations in the lung, decreased precipitation of collagen, decreased content of malondialdehyde (MDA), increased activity of superoxide dismutase (SOD), the restricted proliferation of protected alveolgenisteinar epithelial type II (AE2) cells, (myo) fibroblast, and controlled serum levels of TNF-α, IL-10, IL-6, and transforming growth factor (TGF)-β1. EGCG treatment triggered Nrf-2 and other antioxidant enzymes (NQO-1 and HO-1) at the downstream level. Taken together, these outcomes determined that EGCG therapy remarkably improves irradiation-induced pulmonary fibrosis.
Inflammatory bowel disease (IBD) is a group of inflammatory conditions of the small intestine and colon which influenced a large populace of individuals across the globe. GTPs are certainly efficacious for application in ameliorating IBD and other related unusual complications, with their acknowledged anti-bacterial, antioxidant, and anti-inflammatory activities.GTPs are appeared to have minimum inflammatory reactions in signaling pathways, by downregulating NF-κB, IKK, and cytokines such as Bcl-2, Cox-2, inflammatory markers, and TNF-α, to protect against liver and many other inflammatory diseases (Liu et al., 2017). Many studies reported that GTPs have prebiotic effects which created a healthy and strong microbiota that may be responsible for the anti-inflammatory effects through the production of short-chain fatty acids via direct impact on claudin and occluding proteins (Sharifi-Rad et al., 2020). Ultimately, GTPs may stabilize the flora of the intestine which helps in the retrieval of IBD (Oz 2017).
Polyphenols work as anti-inflammatory compounds that restrict the symptoms of UTIs, Catechins persuade the production of cytokines such as IL-10 and IL-12, GTP reduces the expression of the TNF-α gene which is principal in Escherichia coli pathogenesis.
4.7 Antibacterial, antifungal and antiviral activities
4.7.1 Antibacterial
Urinary tract infections (UTIs) are the usual type of infection in males and females. E. coli is the main reason for this infection (Flores-Mireles et al., 2015). The expansion of antibiotic resistance in E. coli is a major complication (Taheri et al., 2021). Tea polyphenols at a concentration ≥ 5000 μg, showed bactericidal activity and at a concentration of 500 μg can restrict E. coli growth (Noormandi and Dabaghzadeh 2015). According to Reygaert and Jusufi (2013), the bactericidal effect is based on the fact that tea polyphenols down-regulate the generation of proteins intricated in the biosynthesis of amino acid, proteins tangled in energy, carbon, and phospholipid metabolism and EF-2 (elongation factor for protein translation).
Catechins, by stopping the link of conjugated R plasmid inside the E.coli, have antitoxin and bactericidal effects. EGC can inhibit the gyrase enzyme activity by attaching to the site of ATP of the DNA gyrase β subunit of bacteria. The bactericidal function of catechin is because of the generation of hydrogen peroxide (Rahman et al., 2018). Hence, tea exhibits antimicrobial activity due to the presence of catechin and polyphenols as they damage the bacterial cell membrane. Catechins interfere with the β-lactamases expression in Staphylococcus sp. and restrict the extracellular release of verotoxin from enterohemorrhagic E. coli (EHEC) 0157 (Noormandi and Dabaghzadeh 2015).
Acne results in the inflammation, activity of Propionibacterium acnes bacterium, production of sebum by the sebaceous gland, and keratinization of the follicular keratinocytes. Staphylococcus aureus, Propionibacterium granulosum, Staphylococcus epidermidis, and P. acnes are the different bacteria present on acne-prone skin (Lee et al., 2019, Sifaki et al., 2020). Investigations suggested that tea polyphenols control the problem of acne via several mechanisms, by acting as anti-inflammatory, anti-lipogenic, and anti-microbial molecules (Saric et al., 2016). EGCG decreased sebum production by altering the AMPK-SREBP-1 signaling pathway in SEB-1 sebocytes. It also decreases inflammation by restricting the AP-1 and NF-κB pathways. It also directs the cytotoxicity of SEB-1 sebocytes via apoptosis and reduces the viability of almost all pathogenic microorganisms responsible for causing acne (Yoon et al., 2013).
EGCG not only do catechins restrict the extension of both Gram-positive and Gram-negative bacteria, but they raise the number of beneficial bacteria, such as bifidobacteria and lactobacilli (Taylor et al., 2005).
Streptococcus mutans are gram-positive bacteria and grow in rods and chains. These bacteria mainly account for oral health problems, such as plaque and tooth decay. They deteriorate carbohydrates, such as sucrose and lactose, with the help of lactic acid, which erodes the enamels of the tooth. S. mutans spread on teeth by metabolizing sucrose into a sticky sugar that let the attachment and aggregation of plaque. The plaque gathers in the mouth at indentations, dips, and fissures. Moreover, S. mutans are capable to cause deep-rooted infections in the mouth of humans due to their toleration of acidity (Lemos et al., 2019). Streptococcus sobrinus is another species of oral bacteria with finer sticking abilities than S. mutans. However, S. sobrinus are not as usually available in fissures as S. mutans. Furthermore, S. sobrinus has been determined to be cariogenic. Because S. sobrinus and S. mutans have resembling shapes and results in dental caries, so, it becomes inconvenient to differentiate between these two (Conrads et al., 2014).
Staphylococcus epidermidis, bacteria that majorly grow on the membrane of mucous and skin of a human, are also gram-positive type. These white facultative anaerobic bacteria are the main concern in some hospitals because of their potential to cause an infection. Contained in a polysaccharide intercellular adhesion capsule, these bacteria can stick to the extracellular matrix and blood and assemble with other bacteria, resulting in biofilms that are related to infections. Moreover, they show a broad range of tolerance to several antibiotics comprising vancomycin, ciprofloxacin, and other glycopeptide drugs (Otto 2009).
Porphyromonas gingivalis is a very powerful and fatal bacterium that also presents in the mouth of a human. P. gingivalis captures cells and detriment their immune responses with the deterioration of the cellular matrix. Furthermore, P. gingivalis debilitate leukocytes, reorganize the infected cells’ cytoskeleton, and degenerate the IgG antibodies. P. gingivalis utilize well-modified fimbriae to hold and assemble oral cells and saliva-coated apatites in the human mouth. Additionally, this bacterium can produce different enzymes with the capability to degrade host proteins both in vitro and in vivo. Green tea is important for dental health as it prevents the adherence of S. sobrinus and P. gingivitis(Palm et al., 2013, How et al., 2016).
The process of plaque emergence starts when bacteria cling to the tooth surface and produce a glycocalyx film. This film contains a glucan devised from glucosyltransferases (GTF) and arbitrates its attachment to the tooth. Numerous studies have concentrated on GTFs for the prevention of oral diseases. Many experiments reported the inhibitory property of GTC against the growth of S. sobrinus and S. mutans with a minimum concentration of restriction between 50 and 1000 μg/ml, and 250–500 μg/mL in the case of P. gingivalis(Bowen et al., 2018). Lakshmi and Parameswari (2017) reported the anti-cariogenic activity of EGCG against S. mutans by suppressing the specified virulence factors related to its cariogenicity. Moghbel et al., (2011) revealed a herbal mouthwash preparation including Iranian green tea extract with 1 % tannin could minimize the load of aerobic bacteria from the human mouth up to 26–32 % and also, due to this moderation it may inhibit plaque formation and consequently, halitosis.
The activity of EGCG was evaluated against the S. aureus and the in vitro investigation determined that the interaction of EGCG (negatively charged) to the lipids of the membrane (positively charged) destruct the structure of the membrane resulting in intramembranous seepage. EGCG carried out the inhibition of biofilm generation by S. aureus and further results in bacterial cell death by restricting the DNA gyrase of bacteria. Thus, EGCG manifests antifolate activity against DHFR and assists in the inhibition of DNA synthesis (Taylor et al., 2005).
Helicobacter pylori are well recognized as the causative agent of gastric cancer, peptic ulcer, and chronic gastric diseases. EGCG is safe and natural with physiochemical stability in the presence of acid in the stomach, which makes it a curative agent for the cure of gastric disorders at a concentration (inhibition) of 100 µg/ml in the case of H. pylori(Taylor et al., 2005, Kusters et al., 2006). EGCG blocks out the glycosylation of TLR-4 (toll-like receptor), resulting in H. pylori infection, which destroys H. pylori-directed host cell signaling and provides protection from gastric cytotoxicity (Taylor et al., 2005).
Mycobacterium tuberculosis (MTB) is the causative agent of tuberculosis, which activates and induces the pro-inflammatory cytokines (TNF-α) and ROS for its proliferation inside the host cells (Fatima et al., 2012, Fatima et al., 2013). EGCG acts as an inhibitor of reactive nitrogen intermediates and ROS pathways. It also results in stronger inhibition of MTB 85B and TNF-α gene expression in comparison to the first line of antibiotics (Fatima et al., 2012, Fatima et al., 2013).
4.7.2 Antifungal
More than 600 varied species of fungi are discovered to cause usually life-threatening diseases in mankind. The results of EGCG are mainly studied against dermatophytes such as Trichophyton rubrum and Trichophyton mentagrophytes; and yeast strains such as Candida glabrate and Candida albicans(Das et al., 2014). In the case of catechins, pyrogallol catechin showcases greater antifungal activity against C. albicans as compared to catechol catechin. The combined use of EGCG with fluconazole and amphotericin B in well-defined concentrations restricted the growth of amphotericin B and fluconazole-resistant C. albicans(Lakshmi and Parameswari 2017). According to Farhad Mollashahi et al., (2015), the antifungal activity of green tea was based on time and its inhibitory action which did not reduce over time. Das et al., (2014) documented that the formation of biofilm by C. albicans was damaged by the EGCG. It also produces spores in the membrane by attaching to ergosterol and interrupting the osmotic integrity. This results in the seepage of intracellular ions, therefore damaging the cell. Some researchers have reported the antifolic activity of EGCG against dihydrofolate reductase (DHFR), restriction to this important enzyme required for the biosynthesis of different amino acids, pyrimidines, and purines, results in inhibition of the growth of the fungal cells. They also determined that EGCG has a pH-dependent effect such that the inhibitory concentration of microorganisms was diminished by 10 times at more pH in comparison to low pH.
4.7.3 Antiviral
Recent studies revealed that EGCG can boost the immunity of an organism, therefore serving to treat HIV (Fig. 5). For HIV infection to occur, the HIV requires to get enter into CD4 (+) lymphocytes via a step based on the attachment to the CD4 molecule and further proliferation of the virus intracellularly (Boda et al., 2018, Popović-Djordjević et al., 2022). EGCG manifested a vigorous affinity for CD4 and, by interacting with them, could successfully restrict the attachment of the HIV envelope (gp120) (Sinija and Mishra 2008). HIV-1 infection eventually results in diminished immunity because of the binding of envelope glycoprotein 120 (gp120) of HIV-1 virion to the receptor of CD4 in complex with the receptor of chemokine on the surface of the T cell. The infection resulting from the viral entry and the successive deterioration of T cells leads to acquired immunodeficiency syndrome (AIDS). Isolates of HIV-1 are recognizable by the prime co-receptor utilized for cell entry, chemokine receptors CCR5 (R5) or CXCR4 (X4). Based on the genomic variation, HIV-1 has been expressed into three groups: O, M and N. Majorly the infection of HIV-1 has resulted from the M group viruses, and these are further classified into 9 subtypes (K, J, F-H, and A-D) with C and B as the usual one (Wilen et al., 2012).
Nance et al., (2009) explained that a diversity of natural products, like polyphenols, flavonoids, alkaloids, and ribosome-inactivating proteins possess great anti-HIV activity. EGCG has been stated to limit the replication of HIV-1 by focusing on the numerous steps in the HIV-1 life cycle, such as obstructing the protease and reverse transcriptase (RT) activity, sealing interaction of gp120-CD4 by attaching to CD4, and deactivating virions, but all of this was possible at a greater non-physiologic concentration (i.e. greater than 10 µM) of EGCG. EGCG restricted the infectivity of HIV-1 on macrophages and human CD4 + T cells in a dose-dependent manner. At 6 µM concentration, ECGC inhibited the production of HIV-1 p24 antigen across a wide spectrum of both laboratory-adapted subtypes and HIV-1 clinical isolates.
Influenza infection is the main source of acute respiratory disease and turns into an epidemic across the world. To reduce the rate of fatality, a wide variety of prevention mediation have been applied which includes coughing etiquette, hand hygiene, gargling, and the use of facemasks (Islam et al., 2020, Neagu et al., 2021, Islam et al., 2022). Influenza viruses (A and B) belong to the Orthomyxoviridae family and are the main reason for respiratory disease. Several antiviral drugs such as rimantadine and amantadine are used to treat this disease. However, numerous strains of viruses have generated resistance to these drugs and therefore, EGCG has been employed as an important source for influenza infection (Das et al., 2014). Matsumoto et al., (2011), registered about 197 eligible healthcare workers and arbitrarily allocated them to an intervention; 98 were allotted to get theanine/catechin capsules and the other 99 to placebo. The occurrence of influenza infection was abundantly lesser in the group of the theanine/catechin (4.1 %) in comparison to the placebo group (13.1 %). The frequency of laboratory-confirmed cases was also low in the catechin/theanine group (1.0 %) than in the placebo group (5.1 %), but this difference was not denotative. Lakshmi and Parameswari (2017) suggested the antiviral property of catechins against the influenza virus is arbitrated not only because of certain interactions but by altering the physical properties of the viral membrane. EGCG carried out the alteration of physical integrity and agglutination of the virus, thus avoiding their absorbance on MDCK cells (Madin-Dardy Canine Kidney). It also stopped the growth of the influenza virus by restricting the acidification of intracellular components like lysosomes, endosomes, etc and restrained the entry by resulting in haemagglutination (Das et al., 2014).
Adenovirus is related to the family of Adenoviridae, a non-enveloped virus comprised of linear, double-stranded DNA and nucleocapsid. About 5–10 % of infections related to the upper respiratory tract in children are resulted due to this virus (Weber et al., 2003). EGCG deactivated the adenovirus by inhibiting the protease’s activity. It also restrained the late stages of infection and its inside growth (Weber et al., 2003).
Enterovirus 71 is an RNA virus of single-stranded nature, related to the family of Picornaviridae and causes cutaneous, neurological, and life-threatening mouth, foot, and hand disease (Ho et al., 2009). EGCG exhibit its antiviral activity by restricting the replication of the virus and further the generation of viral progeny (Ho et al., 2009).
Steinmann et al., (2013) described the Hepatitis C virus as a positive-strand RNA virus that belongs to the Flaviviridae family with hepatic diseases including hepatocellular carcinoma, liver failure, and cirrhosis. Entry into the host cell comprises a relation between the extracellular virion that is coupled with lipoproteins and various receptors on the cell membrane of the host. These include possibly low-density lipoprotein receptor (LDL-R), Niemann-pickC1-like 1 (NPC1L1), occludin (OCLN), claudin 1 (CLDN1), CD81, EGFR, and scavenger receptor type B class 1 (SR-BI). It has been determined that the lipoprotein receptors LDL-R and SR-BI act first then the component of tight junction OCLN and CLDN1 and CD81.
Such relations contribute to the migration of the virus-receptor complex along the cell surface from the blood-side surface of the hepatic epithelium where CD81, DR-BI, and LDL-R are presented to the region of tight junction, where OCLN and CLDN1 are confronted. These events imitated by virion, directed the turn-on of receptor tyrosine kinase signaling like EGFR which results in clathrin-dependent endocytosis of the virion. Endosome acidification assigned a fusion peptide activity inside the glycoproteins E1 or E2, the viral envelope fuses with the membrane of the endosome and released the nucleocapsid into the cytoplasm. EGCG is reported to target the virus particle and restrict its entry by impairing the binding of the virus to the surface of the cell (Steinmann et al., 2013).
Hepatitis B virus is a small enveloped virus that belongs to the Hepadnaviridae family. It can be transmitted perinatally, sexually, and parentally (Das et al., 2014). EGCG was capable of decreasing the extracellular HBV DNA level and HBV-specific antigens expression, and restricting the intracellular intermediates replication, thus resulting in a declined production of covalently closed circular DNA (Das et al., 2014).
Epstein-Barr virus infection (EBV) is a herpes virus that belongs to the family of Herpesviridae. EGCG limited the immediate-early genes transcription and lytic protein expression, thus blocking the lytic cycle of EBV (Das et al., 2014).
Ebola virus infection (EBOV) results in Ebola haemorrhagic fever. A host chaperon protein, HSPS5, was identified as a valuable target for the treatment of EBOV infection and it was determined that EGCG carried out the restrainment of HSPS5, decreased the generation of new viruses via its inhibitory action on HSPS5 (Xu et al., 2017).
4.8 Other pharmacological properties
4.8.1 Anti-glaucoma
Glaucoma is a multifarious optic neuropathy distinguished by the continuing depletion of retinal ganglion cells (RGCs) and their axons. High intraocular pressure (IOP) looks to be associated with the death of RGC, both in the instance of acute closed-angle glaucoma, when there is an unexpected rise of IOP, and in the case of primary open-angle glaucoma (POAG), which evolves steadily over time (Weinreb et al., 2014). Many risk factors linked with the pathogenesis of glaucoma are the use of topical or systemic corticosteroids, diabetes mellitus, myopia, family history of glaucoma, hispanic origin, black race, and older age (Pimentel et al., 2015). Numerous pathogenic systems seem to be tangled in this disease such as glutamate neurotoxicity, autoimmunity activation, deprivation in neurotrophic growth factor, OS, ischemia, and elevation in IOP (Tezel 2006).
GTCs may protect against glaucoma and other diseases of the eye. Pooman et al., (2018) reported that the tissue of the eye of rats that consume green tea (such as the retina and lens) absorbed catechins present in green tea which upgraded their vision by 20 %.
Two prime mechanisms appeared as protective:
-
cyclic adenosine monophosphate (cAMP)
-
management of mitochondrial dysfunction and OS.
The cAMP is a secondary messenger intricated in discrete cellular pathways. It is important in managing aqueous humour (AH) reabsorption/secretion and in controlling the expression of the neurotrophin gene for RGC durability.
OS is the foremost target of protective agents including melatonin. Especially, the three composites (melatonin, forskolin, and brimonidine) all impart their contribution at a similar time to neuroprotection and IOP control, so that the alliance of these compounds together with the latest therapies of glaucoma could result in auxiliary assistance for patients (Rusciano et al., 2017).
4.8.2 Effects on infertility
Sharpe (2017) determined that the problem of infertility impacts around 15 to 30 % of couples that want to have a baby, therefore, infertility becomes a sensitive issue across the world. ROS are existing in minor concentrations in the genital parts of females and males. Uncontrolled ROS leads to the generation of OS, which causes the deterioration of proteins, lipids, and DNA. Such changes result in reduced sperm motility, raised morphological defects, and compromised vitality in the males whereas, in females, OS interferes with the maturation of the oocyte, and may also restrain the in-vitro maturation of the oocyte (Roychoudhury et al., 2017). The major mechanisms of catechins in various pathways (adenosine monophosphate-activated protein kinase, AMPK; low-density lipoprotein, LDL; adenosine triphosphate, ATP; ferric iron, Fe2+; calcium ion, Ca2+; cyclin adenosine monophosphate, cAMP and reactive oxygen species, ROS) suggest that GTPs can improve the fertility problem by upgrading the quality of embryo (Sharpe 2017). Supplementation of GT can minimize the level of OS and enhance the quality of the gametes in both females and males. At a lesser concentration, it exerts promising effects on oocyte and sperm parameters while they greatly obtain a negative effect at analogously raising concentrations.
Green tea supplementation in male candidates can notably upgrade the viability and motility of sperm parameters. In female candidates, appreciable refinement has been determined in the quality of embryo and oocyte along with clinical pregnancy and the fertilization rate as described by various animal model studies. The EGCG has the environment and cell-type-specific responses because of the signaling molecules and expression of a gene that are distinguishingly managed. Hence, a further detailed investigation is needed to evaluate how EGCG works in the case of reproductive gametes in vivo(Roychoudhury et al., 2017).
4.8.3 Antithrombotic
Thrombosis is the emergence of a blood clot in the venous and arterial systems(Wojciechowski et al., 2017, Sharifi-Rad et al., 2023). It results in different diseases such as limb ischemia, pulmonary embolism, stroke and myocardial infarction. Jin et al., (2008) evaluated that the GTCs showcase their potential antithrombotic effect by the restriction of platelet accumulation. EGCG provides an antiplatelet activity by regulating the different cellular targets, such as maintenance of Ca2+-ATPase activity, inhibitions of phosphoinositide-specific phospholipase Cγ2 (PLCγ2), phosphorylation of tyrosine protein, the liberation of amino acid, and elevation of cellular prostaglandin D2 (PGD2) levels, which may underlie its positive result on the atherothrombotic diseases.
4.8.4 Anti-photoaging
The polyphenols in green tea were reported to have great ROS-neutralizing activity, which makes it a prospective agent in anti-photoaging treatment. Recently, tea polyphenols were given to UV-mediated photoaging mice. A remarkable rise in the content of hydroxyproline was noticed in vitro, and the activity of catalases is hiked along with reduced content of protein carbonyl. The aqueous extract of green tea was found to upgrade the mice's skin affected by photoaging. It was found to raise the level of elastin and collagen fibres and decreased the collagen-degrading MMP-3 enzyme expansion, hence showcasing the antiwrinkle effect (Prasanth et al., 2019).
5 Therapeutic perspectives
Despite green tea is an eminent fat burner, it is well known to diminish or limit various other factors such as vitamin B12, and haemoglobin. It is indicated that the intake of green tea, beyond regulation, does suppress the level of haemoglobin. This is because of the antioxidants like polyphenols that exist in the tea. These polyphenols hold the iron, inhibiting its absorption in the intestine. Although green tea is one of the best powerful drinks, constant intake of green tea can reduce the level of haemoglobin and hence it is preferable to take an iron-rich diet or couple green tea consumption with iron-rich supplements. Consuming multivitamins and iron supplements will not only overcome the negative effects of green tea but will also help green tea to successfully burn fat and remove the free radicals from circulation (Sachdev and Jothipriya 2017). An interesting and promising therapeutic aspect is represented by the potential effect of prolonging life through chronic consumption of green tea. Drinkers of green tea are at a bottom risk of cancer and other diseases, which gives a clue that it could work as a life prolonger. In an investigation of 40,530 Japanese individuals, those who consume five cups or more than that green tea per day were less probably to die for 11 years: Death with other causes: 23 % lesser in females, and 12 % lesser in males. Death because of heart disease: 31 % lesser in females, 22 % lesser in males. Death due to stroke: 42 % lesser in females, and 35 % lesser in males, respectively. In another investigation of 14,001 elderly Japanese people, it was found that individuals who consume green tea were 76 % less expected to die in 6 years (Suzuki et al., 2009). EGCG prolongs the lifespan through the mechanism that is based on the 5′adenosine monophosphate-activated protein kinase/nicotinamide adenine dinucleotide-dependent deacetylase sirtuin-1/forkhead pox protein (AMPK/SIRT1/FOXO) pathway and through mitohormesis. AAK-2/AMPK might be triggered by the EGCG-directed momentary rise in ROS, which regulated EGCG-directed longevity by decreasing the internal level of ROS. AAK-2/AMPK has been manifested to change the metabolism of NAD+, which turns on the SIRT1/SIR-2.1. As a result, SIRT1/SIR-2.1 starts up the FOXO/DAF-16 transcription factor, which carried out the up-regulation of many anti-oxidant genes (Xiong et al., 2018).
5.1 Strategies for enhancing bioavailability and therapeutic efficacy of tea bioactive compounds
EGCG is considered to be a potent antitumor and antioxidant agent, but this has poor absorption and poor stability in the blood and intestinal environment. Research has shown that catechins associated with ascorbic acid can improve their absorption in the digestive system. To increase the bioavailability and therapeutic effect, various methods have been tried, such as chemical structure changes (Dai et al., 2020) and/or incorporation into pharmaceutical nanoparticles to obtain a targeted therapeutic effect (Fig. 6):
-
Methylation- involves modification of either all or part of the eight phenolic hydroxyl groups to methyl ester in the benzene ring. Saijo (1982) isolated a compound named EGCG3′’Me whose stability and solubility get modified after methylation. Its stability was 9-fold greater than that of the original EGCG in the case of oral absorption.
-
Acyclization methods- basically involve the reaction of the phenolic hydroxyl group of catechin molecules with different acylation reagents like acyl chloride to a phenolic ester to decrease the solubility of water and raise the solubility of fat (Wang et al., 2016).
-
Glycoside modification- involves the addition of monosaccharides of hydrophilic nature to eight phenolic hydroxyl groups of catechin. Moon et al., (2006) utilized sucrose-6-glucosyltransferase to carry the reaction of EGCG with sucrose and produced EGCG-7,4′-O-alpha-d-glucopyranoside, EGCG-4′-O-alpha-d-glucopyranoside, and EGCG-7-O-alpha-d-glucopyranoside with 114, 55, and 49 fold increase in water solubility than of sole catechin, respectively.
-
Preparation of nanoparticles of tea bioactive compounds.
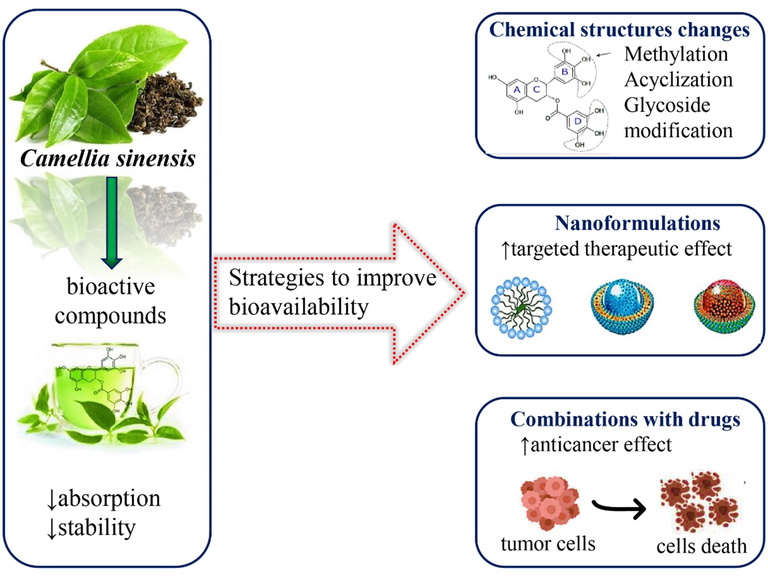
- Summarized scheme with the most important strategies to improve bioavailability of bioactive compounds of Camellia sinensis (L.) Kuntze.
Nanoparticles are molecules that have dimensions in the nanoscale (Docea et al., 2020; Javad Sharifi-Rad et al., 2022). Due to their versatility, they are considered an excellent carriers for drugs at their specific site and location (Pinzaru et al., 2018, Matei et al., 2021).
Catechins are highly unstable molecules as they are susceptible to different conditions such as metal ions, pH change, stress and oxygen. The nanoparticle-encapsulated delivery method is believed to be the better option to protect the catechin against unfavourable conditions (Salehi et al., 2020).
EGCG loaded in lipids showed improved stability. Most of the nanoformulations are water-based, and the loading of EGCG in liposomes promotes the formation of the hydrogen bond between the polar head group and hydrophilic catechins. Based on an in-vitro study, chitosan-tripolyphosphate nanoparticle was found to be a suitable one to improve the stability of EGCG under alkaline conditions (Cai et al., 2018). Chitosan-encapsulated EGCG exhibited greater stability as compared to free EGCG inside the gastrointestinal tract (Sharifi-Rad et al., 2021d) Additionally, they improve the stability and pharmacokinetics of certain drugs (Chen and Lin 2015) as shown in Table 4. Abbreviations and symbols: ↑ (increased), ↓ (decreased), ZnO (Zinc oxide); DOX (Doxorubicin), EGCG (Epigallocatechin gallate), 5-FU (5-fluorouracil).
Nanoparticles of tea components
Biological properties
Therapeutic effects
References
ZnO nanoparticles from green tea leaves
antibacterial
strong inhibition of Aspergillus niger, Escherichia coli, Staphylococcus aureus
(Irshad et al., 2018)
Silver nanoparticles from tea leaves
antibacterial
strong inhibition of Klebsiella pneumonie,Escherichia coli,Corynebacterium diptheriae, Vigna radiata
(Rajput et al., 2020)
antimicrobial cytotoxic
↓gram-positive bacterias at less concentration without causing any harm to HaCaT mammalian cells
(Rolim et al., 2019)
DOX-assisted green tea nanoparticle
anticancer
↑ cytotoxicity in HT-29 cells
(Chen et al., 2019)
Polysaccharide nanoparticles of EGCG
anticancer
↓ tumorigenesis in prostate cancer cells
(Rocha et al., 2011)
Gold and silver nanoparticles from green tea
antimicrobial
↑ antibacterial activity
(Onitsuka et al., 2019)
Gold nanoparticles of green tea
anticancer
↑toxicity against MCF-7 and Ehrlich’s ascites carcinoma
(Mukherjee et al., 2015)
Green tea nano complex with sunitinib
anticancer
↑anticancer activity of sunitinib
(Yongvongsoontorn et al., 2019)
EGCG/cisplatin-loaded gelatin nanoparticles
anticancer
synergistically overcome the resistance to cisplatin
(Chen et al., 2020)
↑anticancer activity in lung cancer cells
EGCG-loaded gold nanoparticle
anticancer
↑EGCG delivery at the targeted site
(Safwat et al., 2020)
↑cytotoxicity in Ehrlich tumour
EGCG and 5-FU co-loaded nanoparticles
Colon cancer
↑ anti-cancer activity of 5-FU
(Wang et al., 2019)
5.2 Synergistic effect of tea catechins combined with antitumor drugs in cancer treatment
The usage of EGCG along with tamoxifen improved the pharmacokinetics of orally consumed tamoxifen in the liver and intestine by restricting CYP3A and P-Glycoprotein by EGCG. EGCG in combination with sulindac remarkably up-regulated the expression of genes related to growth arrest, with a 3-fold rise in p21WAFI and a 12-fold increase in DNA damage-inducible 153 (GADD153). Meanwhile, a nil effect was reported when these two molecules were tested solely (Cai et al., 2018). Other treatment strategies are listed in Table 5 and Fig. 6. Abbreviations and symbols: ↑ (increased), ↓ (decreased), IL-1 (interleukin-1), Am80(synthetic unnatural retinoid; EGFR (epidermal growth factor).
Combinations
Cancer type
Therapeutic effect
References
EGCG + Resveratrol
head and neck cancer
↑ apoptosis
↓cancer cells’ growth(Amin et al., 2021)
Green tea extract + Paclitaxel
ovarian cancer
↑ apoptosis
(Panji et al., 2021)
EGCG + Cisplatin
biliary tract cancer
↑ cell cycle arrest
(Mayr et al., 2015)
Catechin + Doxorubicin
liver cancer
↑anticancer activity of doxorubicin
(Li 2010)
EGCG + Tamoxifen
breast cancer
↑synergistic cytotoxicity
(Chisholm et al., 2004)
EGCG + Celecoxin
pancreatic cancer
↓IL-1
↓tumorigenesis(Härdtner et al., 2012)
Catechin + Am80
lung cancer
down-regulation of histone deacetylase 6,5,4 which induced apoptosis cancer cells
(Oya et al., 2017)
EGCG + Tapentadol
breast cancer
↓proliferation
↑cell-cycle arrest(Bimonte et al., 2019)
EGCG derivative + Paclitaxel/Cisplatin
non-small cell lung cancer
↑anticancer activity
↓ EGFR phosphorylation(Zi et al., 2020)
6 Conclusion
This paper reveals the numerous advantages of different teas for their anti-oxidant, anti-inflammatory, anti-thyroidal, anti-thrombotic, anti-fungal, anti-bacterial and anti-viral properties. They are also being utilized to treat Alzheimer’s, Parkinson’s, oral cavity, and cardiovascular diseases. There is also a broad range of applications of teas in diabetes mellitus, OS associated with infertility, cancer, angiogenesis, pulmonary fibrosis, skin disorders, and inflammatory bowel diseases. Even though the clinical data is still restricted, this article determines the place of teas in both the alternative and conventional medical applications to prevent or help in the therapeutic conventional approach. There is a need for advancement in the area of bioavailability research of tea catechin and nanoformulations to improve accessibility and possible areas of application[183–186]. Multiple molecular targets and disease targets are expected to be explored and research improved to find a more stable, specific, and potent formulation of catechin.
Acknowledgments
We acknowledge the Bioinformatics Center, Banasthali Vidyapith supported by DBT and DST for providing computation and networking support through the FIST and CURIE programs at the Department of Bioscience and Biotechnology, Banasthali Vidyapith, Rajasthan. The author D. Mitra is grateful to the Government of West Bengal, India for Swami Vivekananda Merit Cum Means PhD. Scholarship (WBP191584588825).
Declaration of Competing Interest
The authors declare that they have no known competing financial interests or personal relationships that could have appeared to influence the work reported in this paper.
References
- Green tea polyphenol epigallocatechin 3-gallate in arthritis: progress and promise. Arthritis Res. Ther.. 2010;12:208.
- [CrossRef] [Google Scholar]
- Biology of Parkinson's disease: pathogenesis and pathophysiology of a multisystem neurodegenerative disorder. Dialogues Clin. Neurosci.. 2004;6:259-280.
- [CrossRef] [Google Scholar]
- Catechin hydrate inhibits proliferation and mediates apoptosis of SiHa human cervical cancer cells. Food Chem. Toxicol.. 2011;49:3281-3286.
- [CrossRef] [Google Scholar]
- Neuroprotective effects of white tea against oxidative stress-induced toxicity in striatal cells. Neurotox. Res.. 2011;20:372-378.
- [CrossRef] [Google Scholar]
- White tea consumption improves cardiac glycolytic and oxidative profile of prediabetic rats. J. Funct. Foods. 2015;14:102-110.
- [CrossRef] [Google Scholar]
- Combination of resveratrol and green tea epigallocatechin gallate induces synergistic apoptosis and inhibits tumor growth in vivo in head and neck cancer models. Oncol. Rep.. 2021;45
- [CrossRef] [Google Scholar]
- Effect of yellowing time on bioactive compounds in yellow tea and their antiproliferative capacity in HepG2 cells. Food Sci. Nutr.. 2019;7:1838-1847.
- [CrossRef] [Google Scholar]
- Green tea catechins and cardiovascular health: an update. Curr. Med. Chem.. 2008;15:1840-1850.
- [CrossRef] [Google Scholar]
- The role of catechins in cellular responses to oxidative stress. Molecules. 2018;23
- [CrossRef] [Google Scholar]
- Shining a light on the effects of the combination of (-)-epigallocatechin-3-gallate and tapentadol on the growth of human triple-negative breast cancer cells. In Vivo. 2019;33:1463-1468.
- [CrossRef] [Google Scholar]
- Human papilloma virus: Apprehending the link with carcinogenesis and unveiling new research avenues (Review) Int. J. Oncol.. 2018;52:637-655.
- [CrossRef] [Google Scholar]
- Monoamine oxidase inactivation: from pathophysiology to therapeutics. Adv. Drug Deliv. Rev.. 2008;60:1527-1533.
- [CrossRef] [Google Scholar]
- Insulin receptor signaling in normal and insulin-resistant states. Cold Spring Harb. Perspect. Biol.. 2014;6
- [CrossRef] [Google Scholar]
- Oral biofilms: pathogens, matrix, and polymicrobial interactions in microenvironments. Trends Microbiol.. 2018;26:229-242.
- [CrossRef] [Google Scholar]
- Honey and green/black tea consumption may reduce the risk of Helicobacter pylori infection. Diagn. Microbiol. Infect. Dis.. 2015;82:85-86.
- [CrossRef] [Google Scholar]
- Molecular and cellular stratagem of brain metastases associated with melanoma. Oncol. Lett.. 2019;17:4170-4175.
- [CrossRef] [Google Scholar]
- Beneficial effects of green tea–a review. J. Am. Coll. Nutr.. 2006;25:79-99.
- [CrossRef] [Google Scholar]
- Bioavailability of tea catechins and its improvement. Molecules. 2018;23
- [CrossRef] [Google Scholar]
- Management of endocrinopathies in pregnancy: A review of current evidence. Int. J. Environ. Res. Public Health. 2019;16
- [CrossRef] [Google Scholar]
- The treatment of cognitive, behavioural and motor impairments from brain injury and neurodegenerative diseases through cannabinoid system modulation-evidence from in vivo studies. J. Clin. Med.. 2020;9:28.
- [CrossRef] [Google Scholar]
- Recent advances, approaches and challenges in targeting pathways for potential COVID-19 vaccines development. Immunol. Res.. 2020;68:315-324.
- [CrossRef] [Google Scholar]
- Beneficial effects of green tea: a literature review. Chin. Med.. 2010;5:13.
- [CrossRef] [Google Scholar]
- Anti-fibrotic effect of black tea (Camellia sinensis) extract in experimental pulmonary fibrosis. Tissue Cell. 2019;56:14-22.
- [CrossRef] [Google Scholar]
- Goitrogenic/antithyroidal potential of green tea extract in relation to catechin in rats. Food Chem. Toxicol.. 2010;48:2304-2311.
- [CrossRef] [Google Scholar]
- Neuroprotective and neurorescue effect of black tea extract in 6-hydroxydopamine-lesioned rat model of Parkinson's disease. Neurobiol. Dis.. 2006;22:421-434.
- [CrossRef] [Google Scholar]
- Production, quality, and biological effects of Oolong Tea (Camellia sinensis) Food Rev. Int.. 2010;27:1-15.
- [CrossRef] [Google Scholar]
- Tea and human health: biomedical functions of tea active components and current issues. J. Zhejiang Univ. Sci. B. 2015;16:87-102.
- [CrossRef] [Google Scholar]
- The synergistic anticancer effect of dual drug- (Cisplatin/Epigallocatechin Gallate) loaded gelatin nanoparticles for lung cancer treatment. J. Nanomater.. 2020;2020:9181549.
- [CrossRef] [Google Scholar]
- DOX-assisted functionalization of green tea polyphenol nanoparticles for effective chemo-photothermal cancer therapy. J. Mater. Chem. B. 2019;7:4066-4078.
- [CrossRef] [Google Scholar]
- A review on anti-cancer effect of green tea catechins. J. Funct. Foods. 2020;74:104172
- [CrossRef] [Google Scholar]
- Tamoxifen and epigallocatechin gallate are synergistically cytotoxic to MDA-MB-231 human breast cancer cells. Anticancer Drugs. 2004;15:889-897.
- [CrossRef] [Google Scholar]
- Coêlho, M. L., M. T. Islam, G. Laylson da Silva Oliveira, et al., 2022. Cytotoxic and Antioxidant Properties of Natural Bioactive Monoterpenes Nerol, Estragole, and 3,7-Dimethyl-1-Octanol. Advances in Pharmacological and Pharmaceutical Sciences. 2022, 8002766. https://doi.org/10.1155/2022/8002766
- Comparing the cariogenic species Streptococcus sobrinus and S. mutanson whole genome level. J. Oral Microbiol.. 2014;6:26189.
- [CrossRef] [Google Scholar]
- Bioavailability enhancement of EGCG by structural modification and nano-delivery: a review. J. Funct. Foods. 2020;65:103732
- [CrossRef] [Google Scholar]
- Antimicrobial potential of epigallocatechin-3-gallate (EGCG): a green tea polyphenol. JBPR. 2014;2:167-174.
- [Google Scholar]
- Anticancer potential of alkaloids: a key emphasis to colchicine, vinblastine, vincristine, vindesine, vinorelbine and vincamine. Cancer Cell Int.. 2022;22:206.
- [CrossRef] [Google Scholar]
- White tea (Camellia sinensis L.): antioxidant properties and beneficial health effects. IJFS. 2013;2:19-26.
- [CrossRef] [Google Scholar]
- The effect of silver nanoparticles on antioxidant/pro-oxidant balance in a murine model. Int. J. Mol. Sci.. 2020;21:17.
- [CrossRef] [Google Scholar]
- Neutrophil restraint by green tea: inhibition of inflammation, associated angiogenesis, and pulmonary fibrosis. J. Immunol.. 2003;170:4335-4341.
- [CrossRef] [Google Scholar]
- Effect of green tea extract on experimentally induced lung fibrosis in adult male albino rat. J. Am. Sci.. 2014;10:234-247.
- [Google Scholar]
- Evaluation of antimicrobial activity of aqueous extract of white tea Camellia sinensis L. Kuntze (1887) Pharmacologia. 2011;2:131-136.
- [CrossRef] [Google Scholar]
- Antifungal efficacy of green tea extract against Candida albicans biofilm on tooth substrate. J. Dent. (Tehran). 2015;12:592-598.
- [Google Scholar]
- Chemopreventive efficacy of green tea, ocimum gratissimum and their combination against Benzo[a]pyrene induced preneoplastic lung lesions in murine model. J. Biol. Active Prod. Nat.. 2021;11:22-31.
- [CrossRef] [Google Scholar]
- Fatima, Z., S. Hameed and N. Islam, 2012. Epigallocatechin-2-gallate (EGCG), a green tea polyphenol suppresses bacilli-induced augmented expression of Mycobacterium tuberculosis 85B and proinflammatory TNF-α in human monocytes IJSRP. 2, 1-6
- Green tea polyphenol (EGCG) is a better inhibitor of TNF-α and MTB 85B antigen in human monocytes than known antioxidants and antibiotics. J. Infect. Dis.. 2013;112:131-137.
- [Google Scholar]
- Urinary tract infections: epidemiology, mechanisms of infection and treatment options. Nat. Rev. Microbiol.. 2015;13:269-284.
- [CrossRef] [Google Scholar]
- Current concepts on the reno-protective effects of phosphodiesterase 5 inhibitors in acute kidney injury: systematic search and review. J. Clin. Med.. 2020;9
- [CrossRef] [Google Scholar]
- Bactericidal activity of green tea extracts: the importance of catechin containing nano particles. Sci. Rep.. 2016;6:19710.
- [CrossRef] [Google Scholar]
- Green tea composition, consumption, and polyphenol chemistry. Prev. Med.. 1992;21:334-350.
- [CrossRef] [Google Scholar]
- Aroma compositions of large-leaf yellow tea and potential effect of theanine on volatile formation in tea. Food Chem.. 2019;280:73-82.
- [CrossRef] [Google Scholar]
- White tea (Camellia sinensis) inhibits proliferation of the colon cancer cell line, HT-29, activates caspases and protects DNA of normal cells against oxidative damage. Food Chem.. 2015;169:401-410.
- [CrossRef] [Google Scholar]
- Anti-obesity action of oolong tea. Int. J. Obes. Relat. Metab. Disord.. 1999;23:98-105.
- [CrossRef] [Google Scholar]
- Prevention of collagen-induced arthritis in mice by a polyphenolic fraction from green tea. Proc. Natl. Acad. Sci. U. S. A.. 1999;96:4524-4529.
- [CrossRef] [Google Scholar]
- (-)-Epigallocatechin-3-gallate, a green tea-derived catechin, synergizes with celecoxib to inhibit IL-1-induced tumorigenic mediators by human pancreatic adenocarcinoma cells Colo357. Eur. J. Pharmacol.. 2012;684:36-43.
- [CrossRef] [Google Scholar]
- Haugen, B., J. Hennessey and L. Wartofsky, 2013. Patient information page. Goiter. J Clin Endocrinol Metab. 98, 27A-28A. https://doi.org/10.1210/jcem.98.1.zeg27a.
- Tea and its consumption: benefits and risks. Crit. Rev. Food Sci. Nutr.. 2015;55:939-954.
- [CrossRef] [Google Scholar]
- Characterisation of white tea – comparison to green and black tea. J. Verbr. Lebensm.. 2007;2:414-421.
- [CrossRef] [Google Scholar]
- Antiviral effect of epigallocatechin gallate on enterovirus 71. J. Agric. Food Chem.. 2009;57:6140-6147.
- [CrossRef] [Google Scholar]
- Antihyperglycemic effect of oolong tea in type 2 diabetes. Diabetes Care. 2003;26:1714-1718.
- [CrossRef] [Google Scholar]
- Porphyromonas gingivalis: an overview of periodontopathic pathogen below the gum line. Front. Microbiol.. 2016;7:53.
- [CrossRef] [Google Scholar]
- The anti-obesity effects of green tea in human intervention and basic molecular studies. Eur. J. Clin. Nutr.. 2014;68:1075-1087.
- [CrossRef] [Google Scholar]
- Non-invasive imaging techniques for the in vivo diagnosis of Bowen’s disease: three case reports. Oncol. Lett.. 2019;17:4094-4101.
- [CrossRef] [Google Scholar]
- Inhibitory effect of black tea (Camellia sinensis) theaflavins and thearubigins against HCT 116 colon cancer cells and HT 460 lung cancer cells. J. Food Biochem.. 2019;43:e12822.
- [CrossRef] [Google Scholar]
- Phosphodiesterase-5 inhibitors ameliorate structural kidney damage in a rat model of contrast-induced nephropathy. Food Chem. Toxicol.. 2020;143:111535
- [CrossRef] [Google Scholar]
- Biosensing chips for cancer diagnosis and treatment: a new wave towards clinical innovation. Cancer Cell Int.. 2022;22:354.
- [CrossRef] [Google Scholar]
- Green tea leaves mediated ZnO nanoparticles and its antimicrobial activity. Cogent Chem.. 2018;4:1469207.
- [CrossRef] [Google Scholar]
- Effects of the aqueous extract of white tea (Camellia sinensis) in a streptozotocin-induced diabetes model of rats. Phytomedicine. 2011;19:25-31.
- [CrossRef] [Google Scholar]
- A perspective on emerging therapeutic interventions for COVID-19. Front. Public Health. 2020;8
- [CrossRef] [Google Scholar]
- Neuropharmacological effects of Quercetin: a literature-based review. Front. Pharmacol.. 2021;12
- [CrossRef] [Google Scholar]
- High skin melanin content, vitamin D deficiency and immunity: potential interference for severity of COVID-19. Farmacia. 2020;68:970-983.
- [CrossRef] [Google Scholar]
- Dietary supplements, vitamins and minerals as potential interventions against viruses: perspectives for COVID-19. Int. J. Vitam. Nutr. Res.. 2022;92:49-66.
- [CrossRef] [Google Scholar]
- Tobacco smoking and liver cancer risk: potential avenues for carcinogenesis. J. Oncol.. 2021;2021
- [CrossRef] [Google Scholar]
- Exosome derived from epigallocatechin gallate treated breast cancer cells suppresses tumor growth by inhibiting tumor-associated macrophage infiltration and M2 polarization. BMC Cancer. 2013;13:421.
- [CrossRef] [Google Scholar]
- Chapter 5: Antineoplastic activity of Green tea. In: Rai N., Anand J., eds. Antioxidant Properties and Health Benefits of Green Tea. Nova publishers; 2021. p. :111-132.
- [Google Scholar]
- Myricetin: targeting signaling networks in cancer and its implication in chemotherapy. Cancer Cell Int.. 2022;22:239.
- [CrossRef] [Google Scholar]
- Antiplatelet activity of epigallocatechin gallate is mediated by the inhibition of PLCgamma2 phosphorylation, elevation of PGD2 production, and maintaining calcium-ATPase activity. J. Cardiovasc. Pharmacol.. 2008;51:45-54.
- [CrossRef] [Google Scholar]
- Inhibition of tumour invasion and angiogenesis by epigallocatechin gallate (EGCG), a major component of green tea. Int. J. Exp. Pathol.. 2001;82:309-316.
- [CrossRef] [Google Scholar]
- Implication of green tea as a possible therapeutic approach for parkinson disease. CNS Neurol. Disord. Drug Targets. 2016;15:292-300.
- [CrossRef] [Google Scholar]
- Neuroprotective and therapeutic effect of caffeine on the rat model of parkinson's disease induced by Rotenone. J. Diet. Suppl.. 2017;14:553-572.
- [CrossRef] [Google Scholar]
- Total phenol, catechin, and caffeine contents of teas commonly consumed in the United kingdom. J. Agric. Food Chem.. 2002;50:565-570.
- [CrossRef] [Google Scholar]
- Anticancer effect of black tea extract in human cancer cell lines. Springerplus. 2015;4:127.
- [CrossRef] [Google Scholar]
- Vaccine- and natural infection-induced mechanisms that could modulate vaccine safety. Toxicol. Rep.. 2020;7:1448-1458.
- [CrossRef] [Google Scholar]
- The anti-cancer activity of green tea, coffee and cocoa extracts on human cervical adenocarcinoma HeLa cells depends on both pro-oxidant and anti-proliferative activities of polyphenols. RSC Adv.. 2015;5:3260-3268.
- [CrossRef] [Google Scholar]
- Javad Sharifi-Rad , Z. M. A., Charles O. Adetunji , Olugbenga Samuel Michael, Deepak Chandran , Radha Radha , Niharika Sharma , Manoj Kumar , Daniela Calina, 2022. Neuroprotective effect of curcumin and curcumin-integrated nanocarriers in stroke: from mechanisms to therapeutic opportunities. Minerva Biotechnology and Biomolecular Research. 34, 153-169. https://doi.org/DOI: 10.23736/S2724-542X.22.02946-7.
- Kuban-Jankowska, A., T. Kostrzewa, C. Musial, et al., 2020. Green Tea Catechins Induce Inhibition of PTP1B Phosphatase in Breast Cancer Cells with Potent Anti-Cancer Properties: In Vitro Assay, Molecular Docking, and Dynamics Studies. Antioxidants (Basel). 9, https://doi.org/10.3390/antiox9121208.
- Protective effect of yellow tea extract on N-nitrosodiethylamine-induced liver carcinogenesis. Pharm. Biol.. 2016;54:1891-1900.
- [CrossRef] [Google Scholar]
- Pathogenesis of Helicobacter pylori infection. Clin. Microbiol. Rev.. 2006;19:449-490.
- [CrossRef] [Google Scholar]
- Anticancer activities of epigallocatechin-3-gallate against cholangiocarcinoma cells. Onco. Targets Ther.. 2017;10:137-144.
- [CrossRef] [Google Scholar]
- A review on green tea catechins in oral health management. J. Adva. Pharm. Edu. Res.. 2017;7:178-181.
- [Google Scholar]
- Potential role of the microbiome in acne: a comprehensive review. J. Clin. Med.. 2019;8
- [CrossRef] [Google Scholar]
- Green tea catechins inhibit angiogenesis through suppression of STAT3 activation. Breast Cancer Res. Treat.. 2009;117:505-515.
- [CrossRef] [Google Scholar]
- Green tea polyphenol (-)-epigallocatechin-3-gallate prevents N-methyl-4-phenyl-1,2,3,6-tetrahydropyridine-induced dopaminergic neurodegeneration. J. Neurochem.. 2001;78:1073-1082.
- [CrossRef] [Google Scholar]
- Green tea catechins augment the antitumor activity of doxorubicin in an in vivo mouse model for chemoresistant liver cancer. International Journal of Oncology. 2010;37
- [Google Scholar]
- Chemical constituents and anticancer activity of yellow camellias against MDA-MB-231 human breast cancer cells. J. Agric. Food Chem.. 2013;61:9638-9644.
- [CrossRef] [Google Scholar]
- Flavor characteristics and chemical compositions of oolong tea processed using different semi-fermentation times. J. Food Sci. Technol.. 2018;55:1185-1195.
- [CrossRef] [Google Scholar]
- NF-κB signaling in inflammation. Signal Transduct. Target Ther.. 2017;2:17023-.
- [CrossRef] [Google Scholar]
- Inhibition of adenoma progression to adenocarcinoma in a 4-(methylnitrosamino)-1-(3-pyridyl)-1-butanone-induced lung tumorigenesis model in A/J mice by tea polyphenols and caffeine. Cancer Res.. 2006;66:11494-11501.
- [CrossRef] [Google Scholar]
- EGCG inhibited bladder cancer SW780 cell proliferation and migration bothin vitro and in vivovia down-regulation of NF-κB and MMP-9. J. Nutr. Biochem.. 2017;41:56-64.
- [CrossRef] [Google Scholar]
- White tea extract induces apoptosis in non-small cell lung cancer cells: the role of peroxisome proliferator-activated receptor-{gamma} and 15-lipoxygenases. Cancer Prev. Res. (Phila). 2010;3:1132-1140.
- [CrossRef] [Google Scholar]
- Polysaccharides from Chinese Liupao dark tea and their protective effect against hyperlipidemia. Int. J. Food Sci. Technol.. 2018;53:599-607.
- [CrossRef] [Google Scholar]
- Applications of nanosized-lipid-based drug delivery systems in wound care. Appl. Sci.. 2021;11:4915.
- [Google Scholar]
- Effects of green tea catechins and theanine on preventing influenza infection among healthcare workers: a randomized controlled trial. BMC Complement. Altern. Med.. 2011;11:15.
- [CrossRef] [Google Scholar]
- The green tea catechin epigallocatechin gallate induces cell cycle arrest and shows potential synergism with cisplatin in biliary tract cancer cells. BMC Complement. Altern. Med.. 2015;15:194.
- [CrossRef] [Google Scholar]
- Effects and mechanisms of tea for the prevention and management of diabetes mellitus and diabetic complications: an updated review. Antioxidants (Basel). 2019;8
- [CrossRef] [Google Scholar]
- Inflammatory and oxidative stress markers-mirror tools in rheumatoid arthritis. Biomedicines. 2020;8:14.
- [CrossRef] [Google Scholar]
- The effect of green tea on prevention of mouth bacterial infection, halitosis, and plaque formation on teeth. Iran. J. Toxicol.. 2011;5:502-515.
- [Google Scholar]
- Synthesis, structure analyses, and characterization of novel epigallocatechin gallate (EGCG) glycosides using the glucansucrase from Leuconostoc mesenteroides B-1299CB. J. Agric. Food Chem.. 2006;54:1230-1237.
- [CrossRef] [Google Scholar]
- Podophyllotoxin and its derivatives: Potential anticancer agents of natural origin in cancer chemotherapy. Biomed. Pharmacother.. 2023;158:114145
- [CrossRef] [Google Scholar]
- Adipose tissue fibrosis, hypertrophy, and hyperplasia: correlations with diabetes in human obesity. Obesity (Silver Spring). 2016;24:597-605.
- [CrossRef] [Google Scholar]
- Gold-conjugated green tea nanoparticles for enhanced anti-tumor activities and hepatoprotection–synthesis, characterization and in vitro evaluation. J. Nutr. Biochem.. 2015;26:1283-1297.
- [CrossRef] [Google Scholar]
- (-)-Epigallocatechin gallate inhibits stemness and tumourigenicity stimulated by AXL receptor tyrosine kinase in human lung cancer cells. Sci. Rep.. 2020;10:2444.
- [CrossRef] [Google Scholar]
- Preclinical development of the green tea catechin, epigallocatechin gallate, as an HIV-1 therapy. J. Aller. Clin. Immunol.. 2009;123:459-465.
- [CrossRef] [Google Scholar]
- Back to basics in COVID-19: antigens and antibodies-completing the puzzle. J. Cell Mol. Med.. 2021;25:4523-4533.
- [CrossRef] [Google Scholar]
- Oolong tea: a critical review of processing methods, chemical composition, health effects, and risk. Crit. Rev. Food Sci. Nutr.. 2018;58:2957-2980.
- [CrossRef] [Google Scholar]
- Chemical constituents analysis of white tea of different qualities and different storage times. Eur. Food Res. Technol.. 2016;242:2093-2104.
- [CrossRef] [Google Scholar]
- Effects of green tea on Escherichia colias a uropathogen. J. Tradit. Complement. Med.. 2015;5:15-20.
- [CrossRef] [Google Scholar]
- Green tea extract improves high fat diet-induced hypothalamic inflammation, without affecting the serotoninergic system. J. Nutr. Biochem.. 2014;25:1084-1089.
- [CrossRef] [Google Scholar]
- Preparation of antimicrobial gold and silver nanoparticles from tea leaf extracts. Colloids Surf. B Biointerfaces. 2019;173:242-248.
- [CrossRef] [Google Scholar]
- Staphylococcusepidermidis–the ‘accidental’ pathogen. Nat. Rev. Microbiol.. 2009;7:555-567.
- [CrossRef] [Google Scholar]
- Down-regulation of histone deacetylase 4, -5 and -6 as a mechanism of synergistic enhancement of apoptosis in human lung cancer cells treated with the combination of a synthetic retinoid, Am 80 and green tea catechin. J. Nutr. Biochem.. 2017;42:7-16.
- [CrossRef] [Google Scholar]
- Chronic inflammatory diseases and green tea polyphenols. Nutrients. 2017;9
- [CrossRef] [Google Scholar]
- Nutraceutical profiling, bioactive composition, and biological applications of Lepidium sativum L. Oxid. Med. Cell. Longev.. 2022;2022:2910411.
- [CrossRef] [Google Scholar]
- Porphyromonas gingivalis downregulates the immune response of fibroblasts. BMC Microbiol.. 2013;13:155.
- [CrossRef] [Google Scholar]
- Synergistic effects of green tea extract and paclitaxel in the induction of mitochondrial apoptosis in ovarian cancer cell lines. Gene. 2021;787:145638
- [CrossRef] [Google Scholar]
- Anticancer properties of bromelain: State-of-the-art and recent trends. Front. Oncol.. 2023;12
- [CrossRef] [Google Scholar]
- Association between glucose levels and intraocular pressure: pre- and postprandial analysis in diabetic and nondiabetic patients. J. Ophthalmol.. 2015;2015:832058
- [CrossRef] [Google Scholar]
- Stable PEG-coated silver nanoparticles – a comprehensive toxicological profile. Food Chem. Toxicol.. 2018;111:546-556.
- [CrossRef] [Google Scholar]
- A review on: green tea: a miraculous drink. Int. J. Pharm. Sci. Rev. Res.. 2018;51:26-34.
- [Google Scholar]
- Natural products and synthetic analogues against HIV: a perspective to develop new potential anti-HIV drugs. Eur. J. Med. Chem.. 2022;233:114217
- [CrossRef] [Google Scholar]
- A review of the role of green tea (Camellia sinensis) in antiphotoaging, stress resistance, neuroprotection, and autophagy. Nutrients. 2019;11
- [CrossRef] [Google Scholar]
- Key oncologic pathways inhibited by Erinacine A: a perspective for its development as an anticancer molecule. Biomed. Pharmacother.. 2023;160:114332
- [CrossRef] [Google Scholar]
- PubChem. 2022. PubChem. from https://pubchem.ncbi.nlm.nih.gov/.
- Pharmacological properties of Bergapten: mechanistic and therapeutic aspects. Oxid. Med. Cell Longev.. 2022;2022:8615242.
- [CrossRef] [Google Scholar]
- Therapeutic applications of curcumin in diabetes: a review and perspective. Biomed. Res. Int.. 2022;2022:1375892.
- [CrossRef] [Google Scholar]
- Treatment of inflammatory bowel disease via green tea polyphenols: possible application and protective approaches. Inflammopharmacology. 2018;26:319-330.
- [CrossRef] [Google Scholar]
- Green synthesis of silver nanoparticles using waste tea leaves. Adv. Nano Res.. 2020;3:1-14.
- [CrossRef] [Google Scholar]
- Anti-inflammatory activity of green versus black tea aqueous extract in a rat model of human rheumatoid arthritis. Int. J. Rheum. Dis.. 2017;20:203-213.
- [CrossRef] [Google Scholar]
- Green tea catechin is an alternative immune checkpoint inhibitor that inhibits PD-L1 expression and lung tumor growth. Molecules. 2018;23
- [CrossRef] [Google Scholar]
- Green tea as an effective antimicrobial for urinary tract infections caused by Escherichia coli. Front. Microbiol.. 2013;4:162.
- [CrossRef] [Google Scholar]
- Epigallocatechin gallate-loaded polysaccharide nanoparticles for prostate cancer chemoprevention. Nanomedicine (Lond).. 2011;6:79-87.
- [CrossRef] [Google Scholar]
- Association of cytokine gene polymorphisms with osteoarthritis susceptibility. Exp. Ther. Med.. 2018;16:2659-2664.
- [CrossRef] [Google Scholar]
- Green tea extract mediated biogenic synthesis of silver nanoparticles: characterization, cytotoxicity evaluation and antibacterial activity. Appl. Surf. Sci.. 2019;463:66-74.
- [CrossRef] [Google Scholar]
- Potential role of green tea catechins in the management of oxidative stress-associated infertility. Reprod. Biomed. Online. 2017;34:487-498.
- [CrossRef] [Google Scholar]
- Neuroprotection in glaucoma: old and new promising treatments. Adv. Pharmacol. Sci.. 2017;2017:4320408.
- [CrossRef] [Google Scholar]
- Effect of green tea on haemoglobin. IOSR J. Dent. Med. Sci.. 2017;16:116-118.
- [CrossRef] [Google Scholar]
- Epigallocatechin-3-gallate-loaded gold nanoparticles: preparation and evaluation of anticancer efficacy in ehrlich tumor-bearing mice. Pharmaceuticals (Basel). 2020;13
- [CrossRef] [Google Scholar]
- Isolation and chemical structures of two new catechins from fresh tea leaf. Agric. Biol. Chem.. 1982;46:1969-1970.
- [CrossRef] [Google Scholar]
- Curcumin's nanomedicine formulations for therapeutic application in neurological diseases. J. Clin. Med.. 2020;9:35.
- [CrossRef] [Google Scholar]
- The therapeutic potential of anthocyanins: current approaches based on their molecular mechanism of action. Front. Pharmacol.. 2020;11:20.
- [CrossRef] [Google Scholar]
- Ficusplants: State of the art from a phytochemical, pharmacological, and toxicological perspective. Phytother. Res.. 2021;35:1187-1217.
- [CrossRef] [Google Scholar]
- Simultaneous determination of twelve tea catechins by high-performance liquid chromatography with electrochemical detection. Analyst. 2001;126:816-820.
- [CrossRef] [Google Scholar]
- Green tea and other tea polyphenols: effects on sebum production and acne vulgaris. Antioxidants (Basel). 2016;6
- [CrossRef] [Google Scholar]
- Cannabinoids and inflammations of the gut-lung-skin barrier. J. Pers. Med.. 2021;11
- [CrossRef] [Google Scholar]
- Diosgenin: an updated pharmacological review and therapeutic perspectives. Oxid. Med. Cell. Longev.. 2022;2022:1035441.
- [CrossRef] [Google Scholar]
- Pharmacological activities of psoralidin: a comprehensive review of the molecular mechanisms of action. Front. Pharmacol.. 2020;11:11.
- [CrossRef] [Google Scholar]
- Probiotics: versatile bioactive components in promoting human health. Med. Lith.. 2020;56:30.
- [CrossRef] [Google Scholar]
- Therapeutic potential of neoechinulins and their derivatives: an overview of the molecular mechanisms behind pharmacological activities. Front. Nutr.. 2021;8:664197
- [CrossRef] [Google Scholar]
- Cinnamomum Species: Bridging Phytochemistry Knowledge, Pharmacological Properties and Toxicological Safety for Health Benefits. Frontiers in pharmacology. 2021;12:600139.
- Sharifi-Rad, J., C. Quispe, M. Butnariu, et al., 2021. Chitosan nanoparticles as a promising tool in nanomedicine with particular emphasis on oncological treatment. Cancer Cell Int. 21, 318-318. https://doi.org/10.1186/s12935-021-02025-4.
- Genistein: an integrative overview of its mode of action, pharmacological properties, and health benefits. Oxid. Med. Cell. Longev.. 2021;2021:3268136.
- [CrossRef] [Google Scholar]
- Lifestyle, oxidative stress, and antioxidants: back and forth in the pathophysiology of chronic diseases. Front. Physiol.. 2020;11:21.
- [CrossRef] [Google Scholar]
- Multi-target mechanisms of phytochemicals in alzheimer's disease: effects on oxidative stress, neuroinflammation and protein aggregation. J. Pers. Med.. 2022;12
- [CrossRef] [Google Scholar]
- An updated review on glycoprotein IIb/IIIa inhibitors as antiplatelet agents: basic and clinical perspectives. High Blood Press Cardiovasc. Prev. 2023
- [CrossRef] [Google Scholar]
- A thought on the biological activities of black tea. Crit. Rev. Food Sci. Nutr.. 2009;49:379-404.
- [CrossRef] [Google Scholar]
- Environmental causes of testicular dysfunction. In: Winters S., Huhtaniemi I., eds. Male Hypogonadism. Cham: Humana Press; 2017. p. :281-304.
- [Google Scholar]
- Oolong tea extract induces DNA damage and cleavage and inhibits breast cancer cell growth and tumorigenesis. Anticancer Res.. 2018;38:6217-6223.
- [CrossRef] [Google Scholar]
- MACC1 antibody target therapy suppresses growth and migration of nonsmall cell lung cancer. Mol. Med. Rep.. 2017;16:7329-7336.
- [CrossRef] [Google Scholar]
- Possible mechanisms of green tea and its constituents against cancer. Molecules. 2018;23
- [CrossRef] [Google Scholar]
- A novel approach regarding the anti-aging of facial skin through collagen reorganization. Exp. Ther. Med.. 2020;19:717-721.
- [CrossRef] [Google Scholar]
- Green and black tea extracts inhibit HMG-CoA reductase and activate AMP kinase to decrease cholesterol synthesis in hepatoma cells. J. Nutr. Biochem.. 2009;20:816-822.
- [CrossRef] [Google Scholar]
- ADORA2A rs5760423 and CYP1A2 rs762551 polymorphisms as risk factors for Parkinson's disease. J. Clin. Med.. 2021;10
- [CrossRef] [Google Scholar]
- Antifungal activity of black tea polyphenols (catechins and theaflavins) against Candida species. Chemotherapy. 2009;55:189-196.
- [CrossRef] [Google Scholar]
- Tea: production, composition, consumption and its potential as an antioxidant and antimicrobial agent. Int. J. Food Ferment. Technol.. 2015;5:95.
- [CrossRef] [Google Scholar]
- Anti-infective properties of epigallocatechin-3-gallate (EGCG), a component of green tea. Br. J. Pharmacol.. 2013;168:1059-1073.
- [CrossRef] [Google Scholar]
- Anti-cancer activity of catechin against A549 lung carcinoma cells by induction of cyclin kinase inhibitor p21 and suppression of cyclin E1 and P-AKT. Appl. Sci.. 2020;10
- [CrossRef] [Google Scholar]
- Green tea consumption and mortality among japanese elderly people: the prospective shizuoka elderly cohort. Ann. Epidemiol.. 2009;19:732-739.
- [CrossRef] [Google Scholar]
- The burden of the serious and difficult-to-treat infections and a new antibiotic available: cefiderocol. Front. Pharmacol.. 2021;11:18.
- [CrossRef] [Google Scholar]
- Urtica dioica-derived phytochemicals for pharmacological and therapeutic applications. Evid. Based Complement. Alternat. Med.. 2022;2022:4024331.
- [CrossRef] [Google Scholar]
- Anti-diabetic activity of chemically profiled green tea and black tea extracts in a type 2 diabetes mice model via different mechanisms. J. Funct. Foods. 2013;5:1784-1793.
- [CrossRef] [Google Scholar]
- Health functions and related molecular mechanisms of tea components: an update review. Int. J. Mol. Sci.. 2019;20
- [CrossRef] [Google Scholar]
- Antimicrobial properties of green tea catechins. Food Sci. Technol. Bull.. 2005;2:71-81.
- [CrossRef] [Google Scholar]
- White tea (Camellia sinensis) extract reduces oxidative stress and triacylglycerols in obese mice. Food Sci. Technol.. 2012;32:733-741.
- [CrossRef] [Google Scholar]
- Oxidative stress in glaucomatous neurodegeneration: mechanisms and consequences. Prog. Retin. Eye Res.. 2006;25:490-513.
- [CrossRef] [Google Scholar]
- Targeted metabolomic analysis of serum fatty acids for the prediction of autoimmune diseases. Front. Mol. Biosci.. 2019;6
- [CrossRef] [Google Scholar]
- Reversal of brain aging by targeting telomerase: a nutraceutical approach. Int. J. Mol. Med.. 2021;48
- [CrossRef] [Google Scholar]
- Cucurbitacins as potential anticancer agents: new insights on molecular mechanisms. J. Transl. Med.. 2022;20:630.
- [CrossRef] [Google Scholar]
- Black and green tea and heart disease: a review. Biofactors. 2000;13:127-132.
- [CrossRef] [Google Scholar]
- Enhanced anti-colon cancer efficacy of 5-fluorouracil by epigallocatechin-3- gallate co-loaded in wheat germ agglutinin-conjugated nanoparticles. Nanomedicine. 2019;21:102068
- [CrossRef] [Google Scholar]
- Epigallocatechin gallate inhibits dimethylhydrazine-induced colorectal cancer in rats. World J. Gastroenterol.. 2020;26:2064-2081.
- [CrossRef] [Google Scholar]
- Prodrug of green tea epigallocatechin-3-gallate (Pro-EGCG) as a potent anti-angiogenesis agent for endometriosis in mice. Angiogenesis. 2013;16:59-69.
- [CrossRef] [Google Scholar]
- Antiglycation activity of lipophilized epigallocatechin gallate (EGCG) derivatives. Food Chem.. 2016;190:1022-1026.
- [CrossRef] [Google Scholar]
- Inhibition of adenovirus infection and adenain by green tea catechins. Antiviral Res.. 2003;58:167-173.
- [CrossRef] [Google Scholar]
- The pathophysiology and treatment of glaucoma: a review. JAMA. 2014;311:1901-1911.
- [CrossRef] [Google Scholar]
- WFO. 2021. WFO The World Flora Online. from http://www.worldfloraonline.org/.
- A guide to acquired vitamin K coagulophathy diagnosis and treatment: the Russian perspective. Daru. 2017;25:10.
- [CrossRef] [Google Scholar]
- Green tea polyphenols inhibit malignant melanoma progression via regulating circ_MITF/miR-30e-3p/HDAC2 axis. Biotechnol. Appl. Biochem. 2021
- [CrossRef] [Google Scholar]
- Epigallocatechin-3-gallate promotes healthy lifespan through mitohormesis during early-to-mid adulthood in Caenorhabditis elegans. Redox. Biol.. 2018;14:305-315.
- [CrossRef] [Google Scholar]
- Large yellow tea attenuates macrophage-related chronic inflammation and metabolic syndrome in high-fat diet treated mice. J. Agric. Food Chem.. 2018;66:3823-3832.
- [CrossRef] [Google Scholar]
- Anti-angiogenic effects of green tea catechin on an experimental endometriosis mouse model. Hum. Reprod.. 2009;24:608-618.
- [CrossRef] [Google Scholar]
- A review of the antiviral role of green tea catechins. Molecules. 2017;22
- [CrossRef] [Google Scholar]
- Yellow tea (Camellia sinensisL.), a promising Chinese tea: Processing, chemical constituents and health benefits. Food Res. Int.. 2018;107:567-577.
- [CrossRef] [Google Scholar]
- The immunomodulatory and anti-inflammatory role of polyphenols. Nutrients. 2018;10
- [CrossRef] [Google Scholar]
- Green tea catechins prevent obesity through modulation of peroxisome proliferator-activated receptors. Sci. China Life Sci.. 2013;56:804-810.
- [CrossRef] [Google Scholar]
- Carrier-enhanced anticancer efficacy of sunitinib-loaded green tea-based micellar nanocomplex beyond tumor-targeted delivery. ACS Nano. 2019;13:7591-7602.
- [CrossRef] [Google Scholar]
- Epigallocatechin-3-gallate improves acne in humans by modulating intracellular molecular targets and inhibiting P. acnes. J. Invest. Dermatol.. 2013;133:429-440.
- [CrossRef] [Google Scholar]
- The green tea extract epigallocatechin-3-gallate inhibits irradiation-induced pulmonary fibrosis in adult rats. Int. J. Mol. Med.. 2014;34:92-102.
- [CrossRef] [Google Scholar]
- Neuroprotective effects of oolong tea extracts against glutamate-induced toxicity in cultured neuronal cells and beta-amyloid-induced toxicity in Caenorhabditis elegans. Food Funct.. 2020;11:8179-8192.
- [CrossRef] [Google Scholar]
- Chemistry and biological activities of processed Camellia sinensisteas: a comprehensive review. Compr. Rev. Food Sci. Food Saf.. 2019;18:1474-1495.
- [CrossRef] [Google Scholar]
- Effects of green, oolong and black teas and related components on the proliferation and invasion of hepatoma cells in culture. Cytotechnology. 1999;31:37-44.
- [CrossRef] [Google Scholar]
- Epigallocatechin-3-gallate confers protection against corticosterone-induced neuron injuries via restoring extracellular signal-regulated kinase 1/2 and phosphatidylinositol-3 kinase/protein kinase B signaling pathways. PLoS One. 2018;13:e0192083.
- [Google Scholar]
- Brick dark tea: a review of the manufacture, chemical constituents and bioconversion of the major chemical components during fermentation. Phytochem. Rev.. 2015;14:499-523.
- [CrossRef] [Google Scholar]
- Green tea intake lowers fasting serum total and LDL cholesterol in adults: a meta-analysis of 14 randomized controlled trials. Am. J. Clin. Nutr.. 2011;94:601-610.
- [CrossRef] [Google Scholar]
- Roasting improves the hypoglycemic effects of a large-leaf yellow tea infusion by enhancing the levels of epimerized catechins that inhibit α-glucosidase. Food Funct.. 2018;9:5162-5168.
- [CrossRef] [Google Scholar]
- Synthesis of (-)-epigallocatechin-3-gallate derivative containing a triazole ring and combined with cisplatin/paclitaxel inhibits NSCLC cancer cells by decreasing phosphorylation of the EGFR. J. Chem. Res.. 2020;44:586-591.
- [CrossRef] [Google Scholar]