Translate this page into:
Diagnosing the complex activation factor in rheumatoid arthritis on nanomaterial-modified ELISA
* Corresponding author: E-mail address: hhhtliuchanglu@sina.com (C.-L. Liu)
-
Received: ,
Accepted: ,
Abstract
Rheumatoid arthritis (RA) is a common inflammatory disease that causes functional disability and joint deformity. In RA, the immune system attacks the lining of the joints and tissues of the body. RA generally affects the ankles, hands, and knees. An early diagnosis of RA is imperative for a greater chance of remission and disease control. Various biomarkers have been discovered for diagnosing and treating RA. Among them, interleukin-6 (IL-6) has been established as a biomarker as an increment in its levels was found in RA patients. In this research, a nanomaterial-modified improved enzyme-linked immunosorbent assay (ELISA) was developed to quantify IL-6 levels in spiked human serum samples. Multiwalled carbon nanotube (MWCN) was used to modify the ELISA polystyrene (PS) surface by amine linkers, and then a probe of antibody-gold nanoparticle (GNP)-aptamer was attached onto the MWCN. The probe molecule was created by immobilizing the antibody and aptamer on the GNP. It enhanced the detection of IL-6 and performed better with only antibodies. The absorbance was increased with increasing IL-6 levels, and the detection limit was calculated to be 0.25 pg/mL [y = 0.0636x - 0.0361; R2 = 0.9907] in 10 mM PBS buffer (pH 7.4) and IL-6 spiked serum with the R2 value of 0.9907. Furthermore, experiments with relevant proteins as control namely cyclic citrullinated peptide and matrix metalloproteinases, did not show any absorbance increments signifying the specific detection of IL-6. This IL-6 biosensor quantifies the IL-6 and diagnoses RA and its conditions.
Keywords
Biomarker
Interleukin-6
Nanomaterial
Rheumatoid arthritis
1. Introduction
Rheumatoid arthritis (RA) is an autoimmune inflammatory disorder that mainly damages the joints but also affects other parts of the body, such as the heart, eyes, lungs, and circulatory system [1]. RA occurs when the body’s immune system malfunctions and unintentionally targets the lining of the joints and its tissues. RA generally affects the knees, hands, and ankles. It mostly affects the joint pairs, such as knees or hands [2]. Unlike other bone diseases, RA affects the lining of the joints, which leads to painful swelling, joint deformity, and bone erosion. Mostly, women are affected more often than men and it usually develops in the middle age-groups [3]. Having a family history increases the risk of acquiring RA. Receiving an accurate diagnosis as early as possible is the first step in effective RA treatment. Since RA leads to the deterioration of the ends of the joint bones, ultrasound, magnetic resonance imaging (MRI), and X-ray help in detecting the erosions. The RA cannot be identified with imaging tests until the bone becomes damaged. Imaging tests mainly help in monitoring the treatment. Consequently, researchers are now focusing on finding blood-based biomarkers for diagnosing RA [4,5]. Several biomarkers have been studied in hopes of improving timely and accurate RA diagnosis [6–8]. Among these Interleukin-6 (IL-6) was established as the biomarker for diagnosis and monitoring.
IL-6 is the most abundantly expressed pro-inflammatory cytokine and plays a vital role in propagating RA and its comorbidities [9]. IL-6 is responsible for acute inflammation and immune regulation [10]. As IL-6 functions as a T-cell factor for B-cell recognition and differentiation, it is being extensively targeted to enhance the treatment of a number of inflammatory rheumatic illnesses [11]. Since IL-6 involving inflammatory pathways are responsible for RA progression, the therapeutic options with IL-6 for RA are being widely explored. Anti-IL-6 drugs have been tested with variable degrees of success in RA patients. Increased serum IL-6 levels were reported in RA affected patients. Quantifying levels of IL-6 in human sera helps in identifying RA and its conditions. Researchers have developed various sensing systems for quantifying IL-6 from biological samples [12–14]. At the same time, selectivity for IL-6 is crucial, as it is a big molecule that can be confused with other cytokines if the diagnosis system is only based on the peak position. To rectify this issue, various biosensing systems, such as electrochemical, surface plasmon resonance (SPR), and enzyme-linked immunosorbent assay (ELISA) are the focal point for researchers to quantify the level of IL-6. Among these, ELISA is a simple and established assay, effectively used for diagnosing and monitoring various diseases [15].
ELISA is a gold standard immunoassay to diagnose various bacterial, viral, and molecular species. Diagnosis by ELISA is appealing due to its low cost, lower detection limit, and ease of use. In addition, simultaneous performances with ELISA assay for various diseases are also possible [16]. In conventional ELISA, polystyrene (PS) wells were used to perform the experiment. Antibodies can be attached to the PS plate through electrostatic interaction and identified by their target. Probe immobilization is a key factor in ELISA to determine the selectivity and sensitivity of the target [17]. Smaller molecules such as peptides, nucleic acids, and aptamers are difficult to attach to the PS well. Researchers are working towards improving the immobilization process in ELISA with various chemical and physical modifications [18]. Nanomaterials were found to be effective in immobilizing the probe molecule in the ELISA well [19]. Herein, authors introduced a nanomaterial-modified PS well ELISA for quantifying the biomarker ‘IL-6’ for RA diagnosis.
Nanomaterials are nanosized materials. They are effectively utilized in various biomedical fields, including drug-delivery, biosensors, imaging, and drug screening [20–23]. Nanomaterials are also used for surface modification of biomolecules on the sensor surface to improve the capture, detection, molecular immobilization, and interaction. Herein, two nanomaterials, namely, multi-walled carbon nanotube (MWCN) and gold nanoparticle, were utilized to attach the probe molecules, aptamers, and antibodies in the PS well. Gold is a versatile material, often used in various sensors to enhance detection [24]. For instance, gold nanoparticles (GNPs) conjugated with antibodies and aptamers were found to lower the detection limit to the least femtomolar level in the SPR based sensor system [25]. In another study, Guo et al. studied a GNP-conjugated antibody, which improved the attachment of the antibody in the ELISA well and enhanced the detection limit compared to the conventional detection system sixty-fold [26]. In conventional ELISA, the probe molecule, aptamer, or antibody was directly immobilized, which was not effective for target identification. Here, we used nanomaterials to improve probe immobilization. The ELISA well was modified into a multi-walled carbon nanotube (MWCN) using APTES as linker, and then antibody-GNP-aptamer conjugates were immobilized on MWCN. Finally, IL-6 was detected on this modified ELISA well. The antibody and aptamer were attached to the GNP to create a single probe for IL-6 detection. This system attracts more IL-6 molecules and helps lower the detection limit of the biomarker to diagnose RA-related issues.
2. Materials and Methods
2.1. Chemicals and biomolecules
Multiwalled carbon nanotube (MWCN), APTES (3-aminopropyl)trimethoxysilane, IL-6, anti-IL-6 antibody, gold nanoparticle (GNP, 30 nm), ethanol, Tween 20, cyclic citrullinated peptide (CCP), ELISA plate, human serum, phosphate buffered saline (PBS, pH 7.4) were bought from Sigma Aldrich, USA. Secondary antibody-horseradish peroxidase (HRP) was ordered from Thermo Scientific, USA. BioTEK, Japan, ELISA reader was used to measure the absorbance. The following aptamer sequence was adapted from the previous report [27]: 5′-NH2-GGTGGCAGGAGGACTATTTATTTGCTTTTCT-3′. These sequences were synthesized by the supplier, Apical Scientific, Malaysia.
2.2. Amine modification on MWCN
After dispersing 0.5 g of MWCN in 250 mL of ethanol, the beaker was subjected to an ultrasonication for one hour. Next, 2.5 mL of APTES (100%) was added, and agitated for 4 hrs at 70°C. The modified MWCN was rinsed using acetone and water to eliminate the unbound APTES. Filtration was used to isolate the amine modified MWCN product (MWCN-amine), which was then vacuum-dried for 12 hrs at 100°C.
2.3. Anti-IL-6 antibody-GNP conjugation
Using 1-ethyl-3-(3-dimethylaminopropyl) carbodiimide (EDC) - N-hydroxysuccinimide (NHS), the anti-IL-6 antibodies were conjugated with GNPs and utilized as a probe to detect IL-6. Briefly, 100 mL of GNP was combined with 5 mg of 16-mercaptoundecanoic acid (16-MDA), allowed to rest for an hour, and then centrifuged to collect the 16-MDA-modified GNP. Following that, the 16-MDA-GNP modification was added to EDC-NHS, and the mixture was stirred for 15 mins. Centrifugation was then used to recover EDC-NHS coupled GNP. Lastly, IL-antibody (0.5 mg/mL) was added, and the mixture was constantly agitated for two hrs. The conjugated antibody-GNP was then separated by centrifugation (10000 rpm for ten mins). Distilled water was used to rinse the conjugates several times in order to get rid of any free antibodies in the mixture. The other antibody concentrations (1, 2, 4, and 8 mg/mL) were also conjugated with GNPs with the same procedure.
2.4. Preparation of GNP-aptamer conjugates
Amine linkers were used to create GNP-aptamer conjugates. Briefly, 100 nM of aminated IL-6 aptamer was combined with the received GNP and allowed to stand for an hour. Following that, distilled water was used to rinse the GNP-aptamer conjugate before it was centrifuged and recovered. The same process was used to prepare the aptamer concentration of 200-1000 nM.
2.5. Antibody-GNP-aptamer preparation
To improve IL-6 detection, the antibody-GNP-aptamer conjugates were made and employed as the capture probes. Briefly, the optimized antibody-GNP was combined with 100 pM of aminated IL-6 aptamer and incubated for one hour. Subsequently, the conjugated antibody-GNP-aptamer was recovered by centrifugation and washed with distilled water. To prepare the aptamer concentration of 200 to 1000 pM, the same process was used.
2.6. MWCN modification on ELISA well
Amine-modified MWCN was modified in the ELISA well through amine and KOH interaction. For this process, the ELISA PS well was filled with KOH (1%) for 10 mins, and then 50 µL MWCN dilutions of 0, 0.12, 0.25, 0.5, 1, and 2 mg/mL were added to the wells (totally 6 wells) and rested for 30 mins. The wells were rinsed with 10 mM PBS; anti-IL-6 antibody (1000 nM) was added and kept for 30 mins. The well was further coved using 1 mg/mL of PEG-COOH for 30 mins and rinsed with washing buffer. The secondary antibody HRP (1:1000 dilution) was added and the assembly was rested for 30 mins followed by rinsing five times with washing buffer. Finally, the substrate for HRP was added to see the color changes, and the absorbance was recorded by the ELISA reader. For control, the well was treated without the IL-6 antibody.
2.7. Optimization of anti-IL-6 antibody-GNP on MWCN-modified ELISA well
After optimizing the level of MWCN, IL-6 was optimized on MWCN-modified ELISA well. The steps involved in this experiment were as follows: (a) PS well was filled with 1% KOH; (b) Optimized MWCN-APTES was added followed by resting for 30 mins; (c) Anti-IL-6-antibody concentrations (0.5, 1, 2, 4, and 8 mg/mL) were added in 5 ELISA wells and kept for 30 mins; (d) PEG-COOH was filled in the PS well for 30 mins; (e) IL-6 with 1 ng/mL was added and held for 30 mins; (f) Primary antibody (1:1000 dilution) was added; (g) Secondary antibody-HRP (1:1000 dilution) was added for 30 mins. Finally, the substrate for HRP was added to see the color changes, and the absorbance was recorded by the ELISA reader. A control well was processed without the IL-6 antibody.
2.8. Antibody-GNP-aptamer attachment on MWCN modified ELISA well
Upon optimizing the level of MWCN, the optimal antibody-GNP-aptamer concentration was determined on the MWCN-modified ELISA well. The steps involved in this experiment were as follows: (a) ELISA well was filled with 1% KOH; (b) Optimized MWCN-APTES was added and rested for 30 mins; (c) Anti-IL-6 antibody-GNP-aptamer (Aptamer at 100, 200, 400, 800, and 1600 nM) was added in the ELISA wells and rested for 30 mins; (d) PEG-COOH (1 mg/mL) was filled in the ELISA well for 30 mins; (e) IL-6 with 1 ng/mL was added and kept for 30 mins; (f) Primary antibody was added; (g) Secondary antibody-HRP was added for 30 mins. Finally, the substrate for HRP was added to see the color changes, and the absorbance was recorded by the ELISA reader. The control well was processed without the anti-IL-6 antibody-GNP-aptamer.
2.9. IL-6 quantification with modified ELISA
After all the optimization, IL-6 was quantified with optimized molecules. The steps involved in this experiment were as follows: (a) ELISA well was filled with 1% KOH for 15 mins; (b) Optimized MWCN-APTES was added and rested it for 30 mins; (c) Optimized anti-IL-6 antibody-GNP-aptamer was added in ELISA wells and kept for 30 mins; (d) PEG-COOH was filled in the ELISA well for 30 mins; (e) IL-6 with different concentrations (0.012 to 2000 pg/mL) was added and kept for 30 mins; (f) Primary antibody was added; (g) Secondary antibody-HRP was added for 30 mins. Finally, the substrate for HRP was added to see the color changes, and the absorbance was recorded by the ELISA reader. A control well was processed without IL-6.
2.10. Selective and specific IL-6 detection on modified ELISA
For selective IL-6 detection, concentrations from 0.025 to 2000 pg/mL were spiked in human serum and added to the antibody-GNP-aptamer immobilized ELISA well. All other methods described earlier were followed. The absorbance was recorded to detect IL-6 from the target-spiked human serum samples. For specific ELISA experiments, four different control experiments were conducted as follows: (i) Control with protein CCP instead of IL-6; (ii) Control with protein MMP-9 instead of IL-6; (iii) Without probe molecule; (iv) Without IL-6; On the aptamer modified surfaces, directly antibody was added without adding IL-6. The absorbance of control was compared with the specific IL-6 detection.
3. Results and Discussion
Immobilization of the capture molecule on the ELISA well is crucial and plays a major role in lowering the detection limit of the target molecule. Different immobilization techniques were continuously identified by researchers to improve the target identification. In this research, the new modified sandwich ELISA well was prepared to capture the aptamer through the nanomaterials (MWCN and GNP) for the detection of the RA biomarker IL-6. Since IL-6 has different binding sites for the aptamer and antibody, a sandwich pattern was performed with both. As shown in Figure 1, the KOH-treated well was modified with APTES-MWCN. APTES attachment on the PS well directly lowers its immobilization because of the missing hydrogen bond acceptor, which can be increased by treating the PS well with KOH [19]. Two different surface functionalizations were conducted on these APTES-MWCN surfaces to increase the IL-6 detection. First one is with antibody-GNP, and the second one is with the antibody-GNP-aptamer. In this experiment, the antibody was conjugated with GNP and then attached onto the MWCN-modified PS well, which was compared with the aptamer-conjugated GNP on the MWCN-modified PS well. The main role of the GNP is to increase the aptamer or antibody concentration on the sensing surfaces. When the aptamer is attached on the GNP area, it can cover the entire GNP and improve the aptamer binding on the electrodes. In addition, GNP provides better stabilit, which helps to lower the detection limit of IL-6. Research proved that GNP-biomolecule conjugates enhanced the molecular immobilization and lowered the target molecule identification [28,29]. Guo et al., attached FIX proteins on the ELISA wells through the APTES and GNP. It was identified that the APTES mixed with GNP enhances the attachment of FIX on the ELISA well [26]. In another research, primary antibodies were attached with GNP and to the PS well directly, which was used to detect the Mycobacterium tuberculosis biomarker ESAT-6 with a 1 nM limit of detection [30]. In this research, to improve the probe immobilization, we utilized two nanomaterials (MWCN and GNP) for surface functionalization. The aptamer was conjugated with GNP, and attached to the amine-modified MWCN. Several aptamers were attached to the surface of the single GNP, which increased the aptamer concentration rather than the directly attached aptamer on the sensing surfaces. Further, amine-modified MWCN attract more aptamer-GNPs, which enhances the aptamer attachment and also provides better orientation, which increases the chances of IL-6 interaction with aptamer. We combined aptamer and antibody-immobilized GNP and used it as a single probe for IL detection. MWCN helps to enhance the probe molecular immobilization on the PS well and enhance the biomolecular interaction.
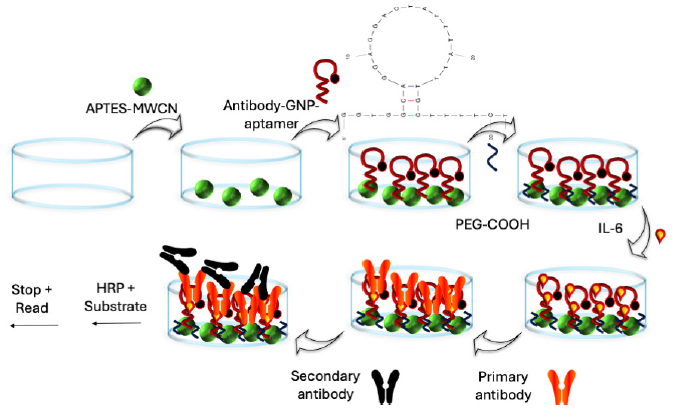
- Schematic illustration for detection of interleukin-6 (IL-6) with modified enzyme-linked immunosorbent assay (ELISA). KOH-treated well was modified with 3-aminopropyl-Multiwalled carbon nanotube (APTES-MWCN). Antibody-gold nanoparticle (GNP)-aptamer was attached to the MWCN, and the IL-6 was added followed by the primary antibody for IL-6. Further secondary antibody-horseradish peroxidase (HRP) and substrate for HRP were added. KOH: Potassium hydroxide.
3.1. MWCN modification on PS well
MWCN modification was used to improve the probe immobilization with proper orientation. For that, different amounts of MWCN (0 to 2 mg/mL) were tested on the PS well. APTES-modified MWCN was attached to the ELISA well, and the IL-6 antibody attachment was monitored by adding primary antibody, secondary antibody-HRP, and substrate. Control functionalization was carried out without IL-6. As shown in the figure, no color change was noticed in the control well. Without MWCN (well 2), the absorbance was 0.15 O. D. MWCN-APTES concentration with 0.125 mg/mL increased the absorbance to 0.22 O.D. Slight difference in absorbance was noticed with 0.125 mg/mL, which confirms that there were some effects on adding MWCN for antibody immobilization in the ELISA PS well. With increment of the MWCN level to 0.25, 0.5, 1, and 2 mg/mL, the absorbances increased to 0.32, 0.38, 0.45, and 0.46 O.D (Figure 2a). Clear changes of color response were noted from the 0.125 mg/mL concentration of MWCN, and it increased with increasing concentrations. Several APTES were linked to the surface of the single MWCN, which attracted a higher number of antibodies on the ELISA well comparatively without MWCN. Apart from that, through the MWCN-APTES linker, the antibody attachment on the PS well is more stable with a proper arrangement and also the reason to improve probe immobilization. The absorbance was saturated from 1 mg/mL; this concentration was optimized to carry on a further experiment.
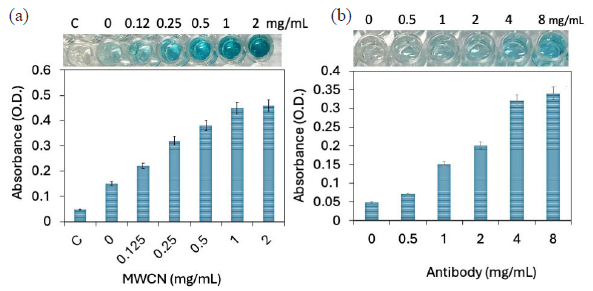
- (a) Multiwalled carbon nanotube (MWCN) modification on polystyrene (PS) well. Different dilutions of MWCN (0 to 2 mg/mL) were optimized on the PS well. Clear changes in color response and absorbance increment were noted from 0.125 mg/mL. The absorbance was saturated from 1 mg/mL concentration. (b) Antibody-gold nanoparticle (GNP) immobilization on PS well. Different concentrations of antibody-GNP were added to the enzyme-linked immunosorbent assay (ELISA) well and detected by interleukin-6 (IL-6), and its antibody. The absorbance wavelength was drastically increased from 800 nM; after that there is not much of absorbance was increased. Experiments have been repeated 5 times and averaged.
3.2. Antibody-GNP immobilization on PS well
After MWCN attachment, the antibody-GNP immobilization was optimized. Since antibody concentration and its attachment play a major role in lowering IL-detection, different concentrations of antibody-GNP were added to the ELISA well and detected by IL-6, and its primary antibody. As shown in Figure 2(b), without the IL-6 antibody, there is no color change. Upon adding 100 nM of antibody-GNP, the well shows a slight color change and increased absorbance level of 0.07 O.D, which confirms the binding of IL-6 antibody on the ELISA plate through the GNP and APTES. Further, increasing IL-6 antibody to 200, 400, 800, and 1600 nM, absorbances were increased to 0.15, 0.2, 0.32, and 0.34 O.D, respectively. The absorbance wavelength was drastically increased to 800 nM, after which there wasn’t much increment, indicating the saturation of antibody-GNP on the MWCN-modified PS well. Various research found that GNP-conjugated biomolecules improve the biomolecule attachment on the sensor surface, and also improve the biomolecular interaction due to its higher stability with biomolecules and sensor surfaces [29,31]. Through the GNP and MWCN, a higher number of antibodies were attached onto the well for the efficient detection of IL-6.
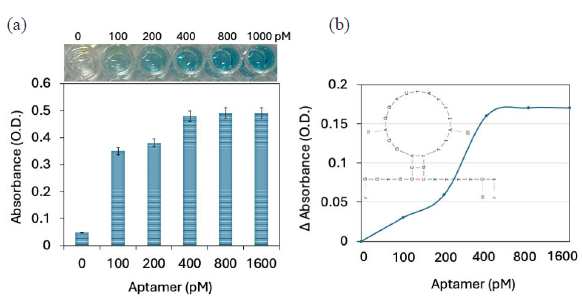
- (a) Antibody-GNP-aptamer attachment on enzyme-linked immunosorbent assay (ELISA) polystyrene (PS) well. Different concentrations of aptamer were added to the antibody-gold nanoparticle (GNP) immobilized ELISA. The gradual increment of absorbance was noted, and it was saturated at 400 pM of aptamer. (b) Comparison of probe immobilization on ELISA PS well. After adding aptamer to the antibody-GNP shows the increment in changes for the same concentration of interleukin-6 (IL-6). The predicted secondary structure of aptamer sequences by UNAfold is shown as a figure inset. Experiments have been repeated 5 times and averaged.
3.3. Antibody-GNP-aptamer attachment on ELISA PS well
After antibody-GNP and aptamer-GNP optimization, antibody-GNP-aptamer immobilization on MWCN was optimized for the comparison. Since aptamers and antibodies have different binding sites with a target molecule, the authors created a single probe with an aptamer and antibody on a GNP to improve IL-6 detection. At first an antibody was immobilized on the GNP surface, and then the gap between the antibodies were covered by the aptamer. Since the antibody is bigger in size, it automatically created the space on the surface of the GNP. In general, these gaps are covered by blocking molecules such as BSA to reduce the biofouling because GNPs easily attract the biomolecules, which leads to a false-positive result. Herein, the excess GNP surface was covered with the aptamer to create a detection molecule with aptamer and antibody for IL-6. To optimize the needed aptamers, different concentrations of aptamers were added to the antibody-GNP immobilized ELISA. Since there is a possibility of less space on the GNP, aptamers were added from the lowest picomolar to nanomolar concentrations. Constant IL-6 was added and detected by its secondary antibody. As shown in Figure 3(a), without IL-6, there was no color change. Upon adding 100 pM of aptamer, the well shows a clear color change and an increased absorbance level of 0.35 O.D, which confirms the binding of aptamer on the antibody-GNP and increases the IL-6 binding on the PS well. Further, increasing aptamer to 200, 400, 800, and 1600 pM, the absorbances were increased to 0.38, 0.48, 0.49, and 0.49 O.D. The increment of absorbance was noted with increasing aptamer concentrations and saturated at 400 pM of the aptamer. The antibody with 4 mg/mL and aptamer at 400 nM, the probe was prepared for IL-6 modified ELISA assay.
3.4. Comparison of probe immobilization on ELISA PS well
Two different types of probe immobilization, namely, antibody-GNP, antibody-GNP-aptamer, were compared to improve the IL-6 detection. Comparing these two experiments, after adding the aptamer to the antibody-GNP shows the increment of current responses for the same concentration of IL-6 (Figure 3b). This experiment confirms that adding aptamers on the excess GNP surface helps to improve the interaction of IL-6 and increase the absorbance. The optimized concentration of antibody and aptamer was utilized for further IL-6 quantification experiments.
3.5. Quantification of IL-6 by improved ELISA
IL-6 was quantified on optimized PS wells. For this, diluted IL-6 from 0.125 to 2000 pg/mL were added on the antibody-GNP-aptamer modified ELISA well probe. Further secondary antibody-HRP followed by the HRP substrate was added to the well to see the color and absorbance changes. As shown in Figure 4(a), 0.125 pg/mL of IL-6 did not show any color change or absorbance increment. When IL-6 levels were increased to 0.25 pg/mL, the absorbance increased from 0.048 O.D to 0.14 O.D, which is approximately a three times absorbance enhancement, indicating the interaction of IL-6 with its aptamer and antibody. Further, after increasing IL-6 to 0.5, 1, 10, 100, 1000, and 2000 pg/mL, absorbances were increased to 0.28, 0.34, 0.41, 0.45, and 0.497 O.D, respectively. Visible color changes were noted from a concentration of 0.25 pg/mL. This increment in absorbance was due to higher probe immobilization on the PS well through MWCN and GNP. Apart from that, due to the higher absorbance, the result can be seen visibly by color changes without the ELISA reader. The absorbance difference was calculated and plotted in an Excel spreadsheet for all IL-6 concentrations. The detection limit was detected as 0.25 pg/mL with an R2 value of 0.9907 (Figure 4b). Until now, ELISA was the well approved sensing system for diagnosing and screening of different diseases. The improved ELISA is suitable to detect any kind of target with its suitable aptamer and antibody and helps to identify a wide range of diseases.
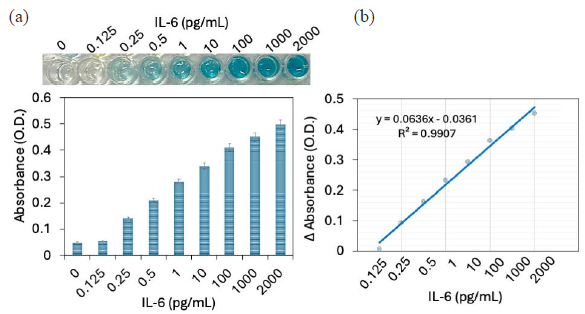
- (a) Quantification of interleukin-6 (IL-6) by improved enzyme-linked immunosorbent assay (ELISA). Diluted IL-6 concentrations from 0.125 to 2000 pg/mL were added to the probe of antibody-gold nanoparticle (GNP)-aptamer modified ELISA well. Increment in absorbance was noted with increasing IL-6. (b) Detection limit of IL-6. The absorbance difference was calculated and plotted in an excel spreadsheet for all IL-6 concentrations, and calculated the detection limit as 0.25 pg/mL with an R2 value of 0.9907. Experiments have been repeated 5 times and averaged.
3.6. Detection of IL-6 in human serum spiked solution
IL-6 was spiked in human serum and detected with improved ELISA modified by MWCN and GNP. As shown in Figure 5(a), the color intensity increased with increasing IL-6 concentrations. The absorbance first drastically increased from 0.5 to 1 pg/mL, after which a slower increment was noted. In healthy human serum, IL-6 levels were very low, (∼ 1-5 pg/mL), and physiological level of IL-6 rapidly increase following trauma, infection, and injury [32]. Serum level of IL-6 was higher in patients with RA (55 IU/mL) compared with the normal control (10 Iu/mL). Quantifying the level of IL-6 in human serum identifies the condition of RA. Identifying the target from the whole serum is still challenging due to the interference of other high molecular weight proteins, such as albumin, in the blood sample. They can easily bind to the surface and lead to false-positive results. Herein, we used the aptamer combined antibody as a probe, which can selectively detect the IL-6 from the human serum. The control experiment was further done with the closely related proteins, namely CCP and MMP. As shown in Figure 5(b), there is no color change and absorbance increment in control wells, indicating a specific IL-6 detection on modified ELISA.
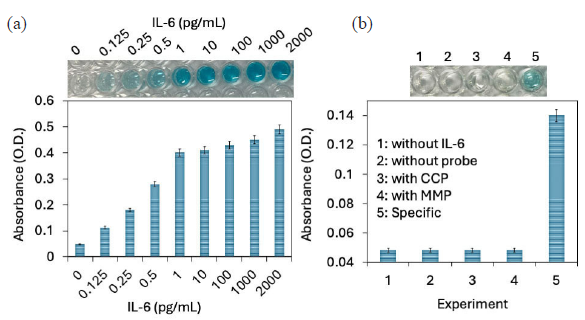
- (a) Detection with interleukin-6 (IL-6) spiked human serum. IL-6 was spiked in human serum and detected with improved enzyme-linked immunosorbent assay (ELISA) modified with Multiwalled carbon nanotube (MWCN) and gold nanoparticle (GNP). The color intensity and absorbance were increased with increasing IL-6 concentration, which confirms the selective IL-6 detection from the human serum. (b) A control experiment was done with the closely related proteins namely CCP and MMP. There is no color change and absorbance increments were noted with control experiments, indicating the specific IL-6 detection on modified ELISA. Experiments have been repeated 5 times and averaged.
4. Conclusions
Rheumatoid arthritis (RA) is a degenerative joint disease, mainly affecting the joints of the knee, ankles, and hands. Nanomaterial-modified ELISA assay was performed for diagnosis and early identification of RA. MWCN and GNP were utilized to attach the probe molecules (aptamer and antibody) to the ELISA well. Antibody and aptamer were attached onto the GNP and created as a single probe, and attached to the MWCN-modified ELISA through the amine modification. The new probe with antibody-GNP-aptamer shows a higher absorbance than with only antibodies. The new surface modification with a single probe system increases the absorbance by increasing the IL-6 and lowers the detection limit to 0.25 pg/mL with an R2 value of 0.9907. Further, target-spiked serum IL-6 shows a gradual increment of absorbance, indicating the selective detection of IL-6, and the control experiments with MMP and CCP do not show any increment in absorbance. This modified ELISA lowers the detection limit of IL-6 and helps to monitor RA and its conditions. The current method has been developed primarily with the interaction of aptamer. If the desired target has no developed aptamer, it needs to be generated before going for analysis. The current method uses MWCN and GNP to enhance the detecting performance. A similar strategy can be implemented with other nanomaterial combinations for high-performance and high-throughput analysis.
Acknowledgment
General Project of Inner Mongolia Autonomous Region Natural Science Foundation (2020MS08143). “Support Project for Young Scientific and Technological Talents” of Higher Education Institutions in Inner Mongolia Autonomous Region (NJYT23071). Joint Project of Inner Mongolia Medical University (YKD2022LH003).
CRediT authorship contribution statement
Dong Su and Zi-Zheng Zhang: Data curation, Formal analysis, Investigation, Writing original draft preparation, Wang Luo and Hong-jian Zhang: Data curation, Methodology, Writing-Reviewing and Editing, Jia-Xing Guo: Data curation, Validation, Visualization, Writing-Reviewing and Editing, Subash C.B. Gopinath: Validation, Visualization, Writing-Reviewing and Editing, Chang-Lu Liu: Conceptualization, Methodology, Project administration, Funding acquisition, Resources, Writing-Reviewing and Editing, All authors have read and agreed with the content of manuscript.
Declaration of competing interest
The authors declare no competing interest.
Declaration of Generative AI and AI-assisted technologies in the writing process
The authors confirm that there was no use of artificial intelligence (AI)-assisted technology for assisting in the writing or editing of the manuscript and no images were manipulated using AI.
References
- The role of TNF-α in rheumatoid arthritis: A focus on regulatory T cells. Journal of Clinical and Translational Research. 2016;2:84-90. https://doi.org/10.18053/jctres.02.201603.005
- [PubMed] [PubMed Central] [Google Scholar]
- Rheumatoid arthritis: A brief overview of the treatment. Medical Principles and Practice. 2018;27:501-7. https://doi.org/10.1159/000493390
- [CrossRef] [PubMed] [PubMed Central] [Google Scholar]
- The natural history of rheumatoid arthritis. Clinical Therapeutics. 2019;41:1256-1269. https://doi.org/10.1016/j.clinthera.2019.04.028
- [CrossRef] [PubMed] [Google Scholar]
- Early biomarkers of joint damage in rheumatoid and psoriatic arthritis. Arthritis Research & Therapy. 2015;17:141. https://doi.org/10.1186/s13075-015-0652-z
- [CrossRef] [PubMed] [Google Scholar]
- Biomarkers in rheumatoid arthritis, what is new? Journal of Medicine and Life. 2016;9:144-8. https://pubmed.ncbi.nlm.nih.gov/27453744/
- [PubMed] [PubMed Central] [Google Scholar]
- Cytokines as biomarkers in rheumatoid arthritis. Mediators of Inflammation. 2014;2014:545493. https://doi.org/10.1155/2014/545493
- [CrossRef] [PubMed] [PubMed Central] [Google Scholar]
- Antibodies against cyclic citrullinated peptide and igA rheumatoid factor predict the development of rheumatoid arthritis. Arthritis and Rheumatism. 2003;48:2741-2749. https://doi.org/10.1002/art.11223
- [CrossRef] [PubMed] [Google Scholar]
- Synovial tissue macrophages: A sensitive biomarker for response to treatment in patients with rheumatoid arthritis. Annals of the Rheumatic Diseases. 2005;64:834-8. https://doi.org/10.1136/ard.2004.029751
- [CrossRef] [PubMed] [Google Scholar]
- The role of interleukin 6 in the pathophysiology of rheumatoid arthritis. Therapeutic Advances in Musculoskeletal Disease. 2010;2:247-256. https://doi.org/10.1177/1759720X10378372
- [CrossRef] [PubMed] [PubMed Central] [Google Scholar]
- Interleukin-6 and chronic inflammation. Arthritis Research & Therapy. 2006;8:S3. https://doi.org/10.1186/ar1917
- [PubMed] [Google Scholar]
- IL-6 biology: Implications for clinical targeting in rheumatic disease. Nature reviews. Rheumatology. 2014;10:720-7. https://doi.org/10.1038/nrrheum.2014.127
- [CrossRef] [PubMed] [Google Scholar]
- A facile graphene conductive polymer paper based biosensor for dopamine, TNF-α, and IL-6 detection. Sensors (Basel). 2023;23:8115. https://doi.org/10.3390/s23198115
- [CrossRef] [PubMed] [PubMed Central] [Google Scholar]
- Paper biosensors for detecting elevated IL-6 levels in blood and respiratory samples from COVID-19 patients. Sensors and Actuators. B, Chemical. 2021;330:129333. https://doi.org/10.1016/j.snb.2020.129333
- [Google Scholar]
- Ultrasensitive label-free sensing of IL-6 based on PASE functionalized carbon nanotube micro-arrays with RNA-aptamers as molecular recognition elements. Biosensors (Basel). 2017;7:17. https://doi.org/10.3390/bios7020017
- [CrossRef] [PubMed] [PubMed Central] [Google Scholar]
- Adaption of a conventional ELISA to a 96-well ELISA-array for measuring the antibody responses to influenza virus proteins and vaccines. Journal of Immunological Methods. 2020;481-482:112789. https://doi.org/10.1016/j.jim.2020.112789
- [CrossRef] [PubMed] [Google Scholar]
- Highly sensitive Hepatitis B virus identification by antibody-aptamer sandwich enzyme-linked immunosorbent assay. INNOSC Theranostics and Pharmacological Sciences. 2022;5:7-14. https://doi.org/10.36922/itps.298
- [Google Scholar]
- A novel approach to evaluate ELISA antibody coverage of host cell proteins—combining ELISA-based immunocapture and mass spectrometry. Biotechnology Progress. 2020;36:e2983. https://doi.org/10.1002/btpr.2983.
- [CrossRef] [PubMed] [PubMed Central] [Google Scholar]
- Microfluidic ELISA: On-chip fluorescence imaging. Biomedical Microdevices. 2004;6:7-9. https://doi.org/10.1023/b:bmmd.0000013360.65653.c2
- [CrossRef] [PubMed] [Google Scholar]
- Graphene oxide-gold nanoparticle-aptamer complexed probe for detecting amyloid beta oligomer by ELISA-based immunoassay. Journal of immunological methods. 2021;489:112942. https://doi.org/10.1016/j.jim.2020.112942
- [CrossRef] [PubMed] [Google Scholar]
- Nanomaterial-enhanced paper-based biosensors. TrAC Trends in Analytical Chemistry. 2014;58:31-9. https://doi.org/10.1016/j.trac.2014.03.008
- [CrossRef] [Google Scholar]
- Artificial intelligence and neuroscience: An update on fascinating relationships. Process Biochemistry. 2023;125:113-120. https://doi.org/10.1016/j.procbio.2022.12.011
- [CrossRef] [Google Scholar]
- Tiny medicine: Nanomaterial-based biosensors. Sensors (Basel). 2009;9:9275-9299. https://doi.org/10.3390/s91109275
- [CrossRef] [PubMed] [PubMed Central] [Google Scholar]
- Artificial intelligence: Potential tool to subside SARS-coV-2 pandemic. Process Biochemistry (Barking, London, England). 2021;110:94-9. https://doi.org/10.1016/j.procbio.2021.08.001
- [CrossRef] [PubMed] [PubMed Central] [Google Scholar]
- Gold-coated carbon nanotube electrode arrays: Immunosensors for impedimetric detection of bone biomarkers. Biosensors & Bioelectronics. 2016;77:580-588. https://doi.org/10.1016/j.bios.2015.10.014
- [CrossRef] [PubMed] [Google Scholar]
- A high-performance waveguide-mode biosensor for detection of factor IX using PEG-based blocking agents to suppress non-specific binding and improve sensitivity. The Analyst. 2013;138:2863-2870. https://doi.org/10.1039/c3an00298e
- [CrossRef] [PubMed] [Google Scholar]
- Complementation of ELISA and an interdigitated electrode surface in gold nanoparticle functionalization for effective detection of human blood clotting defects. Nanoscale Research Letters. 2019;14:222. https://doi.org/10.1186/s11671-019-3058-z
- [CrossRef] [PubMed] [PubMed Central] [Google Scholar]
- Obtaining DNA aptamers to human interleukin-6 for biomagnetic immunoassay nanosensors. Moscow University Physics. 2016;71:135-8. https://doi.org/10.3103/s002713491601015x
- [CrossRef] [Google Scholar]
- Co-immobilized poly(ethylene glycol)-block-polyamines promote sensitivity and restrict biofouling on gold sensor surface for detecting factor IX in human plasma. The Analyst. 2014;139:3977-3985. https://doi.org/10.1039/c4an00168k
- [CrossRef] [PubMed] [Google Scholar]
- A multi-functional gold/iron-oxide nanoparticle-CNT hybrid nanomaterial as virus DNA sensing platform. Biosensors & Bioelectronics. 2018;102:425-431. https://doi.org/10.1016/j.bios.2017.11.052
- [CrossRef] [PubMed] [Google Scholar]
- Signal enhancement in ELISA: Biotin-streptavidin technology against gold nanoparticles. Journal of Taibah University Medical Sciences. 2016;11:432-8. https://doi.org/10.1016/j.jtumed.2016.05.010
- [CrossRef] [Google Scholar]
- Application of gold nanoparticles for electrochemical DNA biosensor. Journal of Nanomaterials. 2014;2014:683460, 7. https://doi.org/10.1155/2014/683460
- [CrossRef] [Google Scholar]
- Unravelling the broader complexity of IL-6 involvement in health and disease. Cytokine. 2021;148:155684. https://doi.org/10.1016/j.cyto.2021.155684
- [CrossRef] [PubMed] [Google Scholar]