Translate this page into:
Investigating the active components and molecular mechanism of alpinia officinarum hance therapy against depression by network pharmacology and molecular docking combined with neuroprotective effects in PC12 cells
* Corresponding author: E-mail address: zhanglitao@mail.jnmc.edu.cn (L. Zhang)
-
Received: ,
Accepted: ,
Abstract
Depression is a common chronic mood-related disorder characterized by depressed mood, loss of interest, and fatigue. Extract from alpinia officinarum hance (AOH) has shown anti-depressant properties in Bagg Albino/C (BALB/c) mice with depression induced by chronic unanticipated stress. However, the molecular mechanisms underlying the anti-depressant effects of AOH remained unclear. The active ingredients of AOH were acquired via the Traditional Chinese Medicine Systems Pharmacology database and analysis platform (TCMSP), and their corresponding targets were collected from the high-throughput experiment- and reference-guided database of traditional Chinese medicine (HERB). Moreover, the targets associated with depression were identified using the GeneCards database. Common targets between the databases were identified as the anti-depressant targets of AOH. The Search Tool for the Retrieval of Interacting Genes/Proteins (STRING) was used to build the Protein-Protein Interaction (PPI) network. Kyoto Encyclopedia of Genes and Genomes (KEGG) pathway and Gene Ontology (GO) enrichment analyses were performed using Database for Annotation, Visualization, and Integrated Discovery (DAVID). Using AutoDock Vina and PyMOL software, molecular docking technology was employed to verify whether the main active components in AOH could bind to central targets. Finally, the CCK8 assay was used to analyze the activity of AOH extract on PC12 cells and depression model cells induced by corticosterone (CORT). The expression was checked via the Quantitative Real - Time Polymerase Chain Reaction (qRT-PCR) of key target molecules. After a successful screening of 10 active components in AOH, it was demonstrated that these compounds had strong relationships with 115 targets associated with depression, among which Interleukin - 6 (IL6), RAC - alpha serine/threonine - protein kinase (AKT1), Interleukin - 1 beta (IL1B), tumor necrosis factor (TNF), Epidermal Growth Factor Receptor (EGFR), and Tumor protein p53 (TP53) could be the potential targets for treating depression. GO and KEGG enrichment pathway analysis yielded 416 terms and 153 pathways, respectively. Quercetin, kaempferol, sitosterol, medicarpin, and galangin were the key active components of AOH involved in treating depression. Molecular docking indicated the screened target proteins had good binding activity with active components with affinity values < 5 kcal/mol. AOH extract was non-cytotoxic to PC12 cells and had a good protective effect on the depression cell model. The qRT-PCR analysis showed that the AOH extract can restore EGFR, TP53, and IL1B levels in CORT-induced PC12 cells to normal. Totally Quercetin, kaempferol, sitosterol, medicarpin, and galangin were identified as possible active ingredients in AOH that could potentially treat depression. The antidepressant benefits of AOH may stem from its capacity to modulate neuroinflammation.
Keywords
Alpinia officinarum
Depression
Molecular mechanism
Network pharmacology
1. Introduction
Depression is a common chronic mood-related disorder characterized by depressed mood, loss of interest, and fatigue, which affects people all around the world and significantly lowers their quality of life [1]. The high incidence of severe depression will pose a significant risk to human well-being [2]. World Health Organization (WHO) has classified severe depression as the third most significant global burden of disease, and it has been predicted to rank first by 2030 [3]. There is no consensus regarding the best drugs to treat depression since the molecular causes of the condition are complicated and not fully understood [4]. Drug resistance, significant adverse reactions, and a high risk of relapse upon withdrawal are the current issues associated with anti-depressant drugs. Drugs exhibiting a good safety profile, highly targeted action, and minimal side effects against depression are urgently required. Depression has been effectively treated using traditional Chinese medicine (TCM), which has been shown to provide long-lasting, moderate, and steady benefits with a low incidence of adverse effects. This makes it a suitable option for long-term medication [5]. Therefore, TCM has gained significant attention from researchers in their pursuit to develop anti-depressant drugs in recent years.
The Chinese native pungent plant with an aromatic rhizome, Alpinia officinarum Hance (AOH, Gao Liang Jiang; Zingiberaceae), has been used as a European spice for over a millennium [6]. Traditionally used as a medicinal plant in China, it is also widely grown in Southeast Asia, West Bengal, Assam, and the Eastern Himalayas. It has long been used as a culinary spice in Ayurvedic and Chinese medicine [7]. Oriental medicine has historically utilized the rhizome of this plant for its anti-arthritic, anti-emetic, anti-phlogistic, carminative, analgesic, stomachic, and anti-spasmodic properties [8-10]. Current research in pharmacology demonstrated that AOH exhibited strong pharmacological effects both in vivo and in vitro, such as angiogenesis, anti-microbial, anti-cancer, anti-inflammatory, anti-diabetic, vasorelaxation, anti-diarrhea, anti-emetic, analgesic, anti-coagulation, and anti-obesity, and antioxidant activity [3,11]. AOH’s yang-warming, cold-dispersing, qi-regulating, liver-dispersing, and depression-resolving abilities are also well-known [12]. In Bagg Albino/C (BALB/c) mice with depression brought on by prolonged and unanticipated stress, the treatment with hydroalcoholic extract of AOH demonstrated anti-depressant properties [13]. However, it remained unclear the way AOH functioned on a molecular level to alleviate depression. Traditional experimental methods for analyzing the molecular mechanisms of TCM on depression require significant financial and human resources. Utilizing network pharmacology may efficiently give unbiased verification of TCM’s ability to treat depression and offer novel perspectives on the development of innovative anti-depressant drugs [14,15]. Furthermore, a computational technique called molecular docking can be employed to predict the interaction and binding affinity between ligands and proteins [16]. Molecular docking and network pharmacology offer rapid solutions for studying the cellular and molecular mechanisms followed by TCM.
In this study, the effective ingredients of AOH were initially screened by the Traditional Chinese Medicine Systems Pharmacology database and analysis platform (TCMSP). Then, molecular docking and network pharmacology analysis were used to estimate the probable target genes and signaling pathways linked to AOH’s anti-depressant action. The PC12 cell injury model generated by corticosterone (CORT) has been widely used as an in vitro model to evaluate the mechanisms and effects of anti-depressants [17]. Moreover, the acting molecules of the AOH extract and their positive effects on CORT-induced PC12 cells were identified. The study establishes a theoretical foundation for utilizing AOH as a therapeutic approach for depression.
2. Materials and Methods
2.1. Database and software
The Traditional Chinese Medicine Systems Pharmacology database and analysis platform (TCMSP; http://tcmspw.com/tcmsp.php), PubChem (https://pubchem.ncbi.nlm.nih.gov/), Chemical European Molecular Biology Laboratory (ChEMBL; https://www.ebi.ac.uk/chembl/), Swiss Absorption, Distribution, Metabolism and Excretion (SwissADME; http://www.swissadme.ch/), Comparative Toxicogenomics Database (CTD; http://ctdbase.org/), high-throughput experiment- and reference-guided database of traditional Chinese medicine (HERB; http://herb.ac.cn/), GeneCards (https://www.genecards.org/), the Search Tool for the Retrieval of Interacting Genes/Proteins (STRING; https://string-db.org/), Database for Annotation, Visualization, and Integrated Discovery (DAVID; https://david.ncifcrf.gov/), Bioinformatics online tools (http://www.bioinformatics.com.cn/), Prediction of Activity Spectra for Substances Online tool (PASS Online tool; http://www.way2drug.com/passonline/), Cytoscape, ChemDraw, Pymol and AutoDock Vina.
2.2. Chemicals and reagents
The rat pheochromocytoma cells (PC12 cells) and their specific culture medium were purchased from Wuhan Procell Co., Ltd. CORT was purchased from Aladdin Co., Ltd. The cell proliferation detection reagent (CCK-8) was purchased from Abbkine Technology Co., Ltd. The 0.25% trypsin was purchased from Biosharp Technology Co., Ltd. Moreover, the reverse transcription and Quantitative Real - Time Polymerase Chain Reaction (qRT-PCR) kits were purchased from Cwbio Biological Technology Co., Ltd., and the UNIQ-1 column RNA extraction kit and primers were procured from Sangon Biotech Co., Ltd.
2.3. Network pharmacology analysis
2.3.1. Screening of active components in AOH
The TCMSP database was searched for active ingredients in AOH, using the following criteria: drug-likeness (DL) ≥ 0.18, oral bioavailability (OB) ≥ 30%, and the number of bonds that permit free rotation around themselves (RBN) ≤ 10 [18]. PubChem and ChEMBL were employed to conduct structural validation and activity prediction for the active ingredients screened in AOH. Meanwhile, SwissADME was utilized to predict the key pharmacokinetic parameters as well as the drug - likeness properties of the active ingredients. The PASS Online tool was used to predict biological activities of AOH components.
2.3.2. Screening for anti-depressant targets of AOH
Using the GeneCards, targets related to depression were identified. Moreover, the HERB database was utilized to access the targets of the active components in AOH. The purpose of the MyVenn tool on the CTD platform was to display the overlap of genes linked to depression and the active components of AOH [19].
2.3.3. Network construction
Using STRING for multiple proteins in human (Homo sapiens), the Protein-Protein Interaction (PPI) Network was developed for the overlapping targets of AOH compounds and depression. The Cytoscape was used to show the network graphically. To establish the ‘herbal-active ingredient-anti-depressant target network’ of AOH, the overlapping targets and their active components were imported into Cytoscape.
2.3.4. Target gene functional enrichment analysis
The DAVID, GO (Gene Ontology), and KEGG (Kyoto Encyclopedia of Genes and Genomes) were utilized for performing the pathway enrichment analyses on the potential anti-depressant targets of AOH, with a p ≤ 0.01 threshold. Bioinformatics online resources were employed for generating bubble plots and bar charts.
2.3.5. Molecular docking
The PubChem database was utilized to search for key active ingredients, and the ChemDraw tool was used to draw their chemical structures. Moreover, ChemBio 3D was employed to produce three-dimensional chemical structures, and Calculation-MM2-Minimize Energy-Run was utilized for conformational optimization procedures. Finally, the structure data file (SDF) format of active ingredients was saved and later converted into protein data bank (PDB) file format using Pymol software. After exporting the protein’s PDB file from the Research Collaboratory for Structural Bioinformatics (RCSB) PDB database, AutoDock Vina eliminated water and incorporated hydrogen atoms and charges into the core target’s crystal structure before storing it in PDB format. Using AutoDock Vina, molecular docking between key active compounds and core targets was accomplished. Finally, the binding mode with the lowest binding energy was selected for further analysis. The interaction between bioactive compounds and key targets was shown as a 3D diagram using Pymol.
2.4. Experimental validation in vitro
2.4.1. Cell culture maintenance and treatment
PC12 cells were grown in a culture medium with 5% CO2 and 37°C. Log-phase cells were cultured at a seeding density of 5000 cells/well in a 96-well cell culture plate. After 24 hrs, different concentrations (0, 16.9, 33.8, 67.5, 125, 250, and 500 μg/mL) of AOH extract were added to the cells. The CCK8 test was used to evaluate the extract’s toxicity on PC12 cells over 24 hrs. The 96-well cell culture plate’s log-phase cells were exposed to 200 μM of CORT for 24 hrs to generate the in vitro depression model. Furthermore, gradient concentrations of AOH extract were added, and the CCK8 assay was used to measure the cell viability. Cells without CORT and AOH extract served as a blank control, while cells treated with CORT were the model group.
2.4.2. Validation of target gene expression
RNA extraction was performed using the UNIQ-1 column-based RNA extraction kit. Using a reverse transcription kit, cDNA was produced, and qRT-PCR was performed to determine the target genes influenced by AOH in terms of expression level. The 10 μL reaction system was made up of 1 μL cDNA template, 2 μL forward primer, 2 μL reverse primer, and 5 μL 2 × MagicSYBR Mixture. The target gene’s expression level was determined using the 2-ΔΔCq technique, with β-actin as the reference gene. Table 1 shows a list of primers used in this assay.
Primer | Sequence |
---|---|
ACTIN-F | 5’-ACATCCGTAAAGACCTCTATGCC-3’ |
ACTIN-R | 5’-TACTCCTGCTTGCTGATCCAC-3’ |
AKT1-F | 5’-GTCACCTCTGAGACCGACACC-3’ |
AKT1-R | 5’-GCCTCCGTTCACTGTCCAC-3’ |
TP53-F | 5’-GCTTCGAGATGTTCCGAGAG-3’ |
TP53-R | 5’-AGACTGGCCCTTCTTGGTCT-3’ |
EGFR-F | 5’-GGCATCATGGGGGAGAACAA-3’ |
EGFR-R | 5’-TCTTTGGCCCATAGGTACAGTT-3’ |
BAX-F | 5’-CGAGCTGATCAGAACCATCA-3’ |
BAX-R | 5’-CTCAGCCCATCTTCTTCCAG-3’ |
BCL2-F | 5’-CTCAGTCATCCACAGGGCGA-3’ |
BCL2-R | 5’-AGAGGGGCTACGAGTGGGAT-3’ |
Caspase3-F | 5’-GGACCTGTGGACCTGAAAAA-3’ |
Caspase3-R | 5’-GCATGCCATATCATCGTCAG-3’ |
3. Results and Discussion
3.1. Active components and possible AOH targets
From the TCMSP database, a total of 130 chemical constituents of AOH were obtained. Several screening criteria, such as OB ≥ 30%, DL ≥ 0.18, and RBN ≤ 10, were employed to determine the active ingredients of AOH. Moreover, 10 active components were found following chemical structure analysis, and then based on the data from PubChem and ChEMBL, the names of the active components were meticulously corrected. Simultaneously, the 2D structures were obtained by extracting the Simplified Molecular Input Line Entry Specification (SMILES) from these two databases (Table 2). The pharmacokinetic properties, including gastrointestinal (GI) absorption, blood-brain barrier (BBB) permeability, and P-glycoprotein (P-gp) substrate status, have been summarized in Table 2. Among the active components, all compounds except sitosterol exhibited high GI absorption. Notably, 50% of the molecules were predicted to be BBB permeant. Furthermore, only two compounds—2-(3,4-dimethoxyphenyl)-5,7-dimethoxy-3-chromanol and medicarpin—were identified as P-gp substrates. The drug-likeness and bioavailability scores of the compounds were assessed through the SwissADME database (Table 2) and the results indicated that all compounds meet the Lipinski’s rule of five and exhibited a bioavailability score of 0.55.
No. | Molecule name | MW | OB (%) | DL | RBN | Pharmacokinetics | Druglikeness | 2D Structure | |||
---|---|---|---|---|---|---|---|---|---|---|---|
GI absorption | BBB permeant | P-gp substrate | Lipinski rule of five | Bioavailability score | |||||||
1 | 5-methoxy-1,7-diphenyl-3-heptanone | 296.44 | 68.29 | 0.2 | 9 | High | Yes | No | Yes | 0.55 |
![]() |
2 | 1,7-diphenyl-5-hydroxy-3-heptanone | 282.41 | 61.9 | 0.18 | 8 | High | Yes | No | Yes | 0.55 |
![]() |
3 | 2-(3,4- Dimethoxyphenyl)- 5,7-dimethoxy-3-chromanol | 346.41 | 51.89 | 0.37 | 5 | High | Yes | Yes | Yes | 0.55 |
![]() |
4 | isorhamnetin | 316.28 | 49.6 | 0.31 | 2 | High | No | No | Yes | 0.55 |
![]() |
5 | medicarpin | 270.3 | 49.22 | 0.34 | 1 | High | Yes | Yes | Yes | 0.55 |
![]() |
6 | quercetin | 302.25 | 46.43 | 0.28 | 1 | High | No | No | Yes | 0.55 |
![]() |
7 | galangin | 270.25 | 45.55 | 0.21 | 1 | High | No | No | Yes | 0.55 |
![]() |
8 | kaempferol | 286.25 | 41.88 | 0.24 | 1 | High | No | No | Yes | 0.55 |
![]() |
9 | 8-[(2S)-2-ethoxy-3-hydroxy-3-methylbutyl]-7-methoxychromen-2-one | 306.39 | 40.36 | 0.21 | 6 | High | Yes | No | Yes | 0.55 |
![]() |
10 | Sitosterol | 414.79 | 36.91 | 0.75 | 6 | Low | No | No | Yes | 0.55 |
![]() |
MW: Molecular weight, OB: Oral bioavailability, DL: Druglikeness, RBN: Number of rotatable bonds, GI Absorption: Gastrointestinal absorption, BBB Permeant: Blood-brain barrier Permeability, P-gp Substrate: P-glycoprotein substrate, AOH: Alpinia officinarum hance.
Using the HERB databases, 205 targets were selected from the 10 active components in AOH. To generate the herbal-active ingredient-target network depicted in Figure 1, each active ingredient in AOH that was acquired, as well as their possible targets, were loaded into the Cytoscape program. The herb AOH was represented by the orange triangular ellipse nodes, the constituents of AOH were represented by the green ellipse nodes, and the targets were shown by the blue rectangle nodes. The multi-component and multi-target properties of AOH were confirmed by the edges, which demonstrated the association between the active components and the targets.
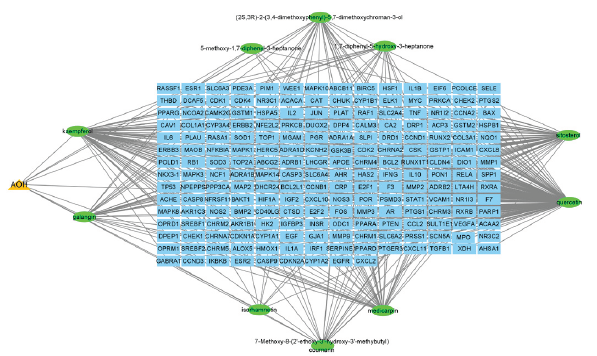
- Construction of the herbal-active ingredient-target network. The nodes with orange triangles symbolize the herb AOH, the green ellipses represent AOH’s components, and the blue rectangles indicate the targets.
3.2. Core target acquisition in AOH
To find potential targets linked to depression from the GeneCards, the term ‘depression’ was searched. Out of 10371 genes, a total of 1630 genes correlated with depression were found based on a relevance score > 3.0. The MyVenn tool on the CTD platform yielded 115 AOH treatment depression targets due to the intersection of AOH targets and depression targets (Figure 2).
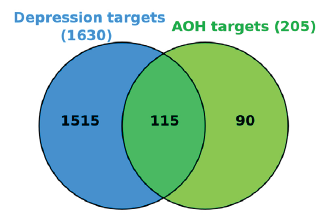
- Venn diagram of the targets of depression and AOH. AOH: Alpinia officinarum hance.
3.3. PPI Network construction and analysis
The STRING platform received 115 targets that were shared by both drugs and diseases. These targets were then used to establish PPI networks, and high-confidence interactions (>0.7) were selected. Furthermore, to create a function-related PPI network, the PPI network was uploaded into Cytoscape after isolated nodes were removed (Figure 3a). The network has 883 edges and 108 nodes, with a 15.4 mean connection degree. A PPI enrichment p-value < 1.0 × 10-16 indicates a tighter network than anticipated. After conducting a topology analysis based on BC (Betweenness centrality), CC (Closeness centrality), and DC (Degree centrality), 14 core targets were identified and sorted by degree values to determine the important targets for AOH in the treatment of depression (Figures 3b, c). Interleukin 6 (IL6), RAC - alpha serine/threonine - protein kinase (AKT1), Interleukin 1 Beta (IL1B), Tumor Necrosis Factor (TNF), Epidermal Growth Factor Receptor (EGFR), Tumor protein p53 (TP53), Jun proto - oncogene (JUN), Matrix Metallopeptidase 9 (MMP9), Caspase 3 (CASP3), and B - cell lymphoma 2 (BCL2) were the top 10 key targets.
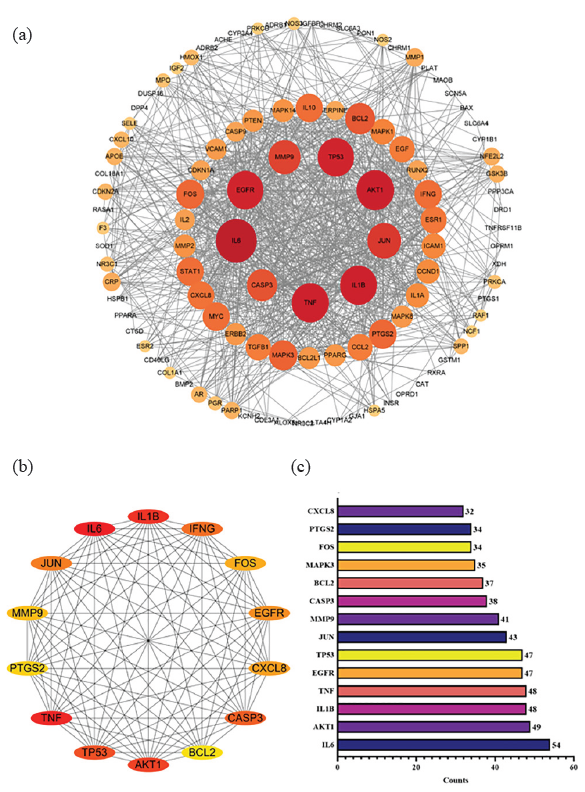
- Interaction network of 115 predicted targets (a) network of PPI with 115 predicted targets; (b) the top 14 key proteins’ network; (c) ranking by degree values of the top 14 targets. PPI: Protein-protein interaction.
3.4. GO and KEGG pathway enrichment analysis
With a p-value of less than 0.01 for each of the 115 identified targets for AOH in the depression treatment, GO and KEGG pathway enrichment analyses were performed using DAVID. Biological processes (BP), cellular components (CC), and molecular functions (MF) were the three components of the GO enrichment analysis. A total of 416 entries were enriched: 315 for BP, 46 for CC, and 55 for MF. Figure 4 displays the top 10 significant terms in the BP, CC, and MF categories. BP mainly involved processes such as response to xenobiotic stimulus, positive regulation of DNA-templated transcription, positive regulation of transcription by RNA polymerase II, positive regulation of Mitogen - Activated Protein Kinase (MAPK) cascade, cellular response to cadmium ion, positive regulation of gene expression, response to hypoxia, negative regulation of apoptotic process, positive regulation of miRNA transcription, apoptotic process, etc. CC included components such as extracellular space, extracellular region, mitochondrion, cell surface, caveola, etc. MF involved functions such as protease binding, protein homodimerization activity, identical protein binding, enzyme binding, nuclear receptor activity, protein binding, cytokine activity, estrogen response element binding, etc.
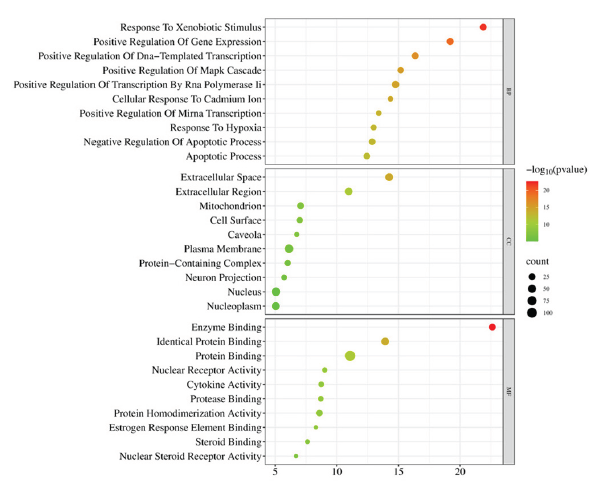
- Top 10 GO terms of AOH in the depression treatment. GO: Gene ontology, AOH: Alpinia officinarum hance.
A p-value of less than 0.01 was found for 153 critical pathways in the KEGG pathway enrichment analysis. Eleven pathways related to cellular processes, 45 to organismal systems, 22 to environmental information processing, and 75 to human disease were divided into four main groups (Figure 5). The top 10 pathways for the cellular processes, organismal systems, and environmental information processing were determined based on the p-value and have been displayed in Figure 6. The results primarily involved the pathways for HIF-1, MAPK, TNF, PI3K-Akt, and ErbB signals within environmental information processing; in organismal systems, the pathways for IL-17, relaxin, osteoclast differentiation, c-type lectin receptor, and T-cell receptor signals were involved; and in cellular processes, the pathways for apoptosis, focal adhesion, p53, and gap junction signals were present.
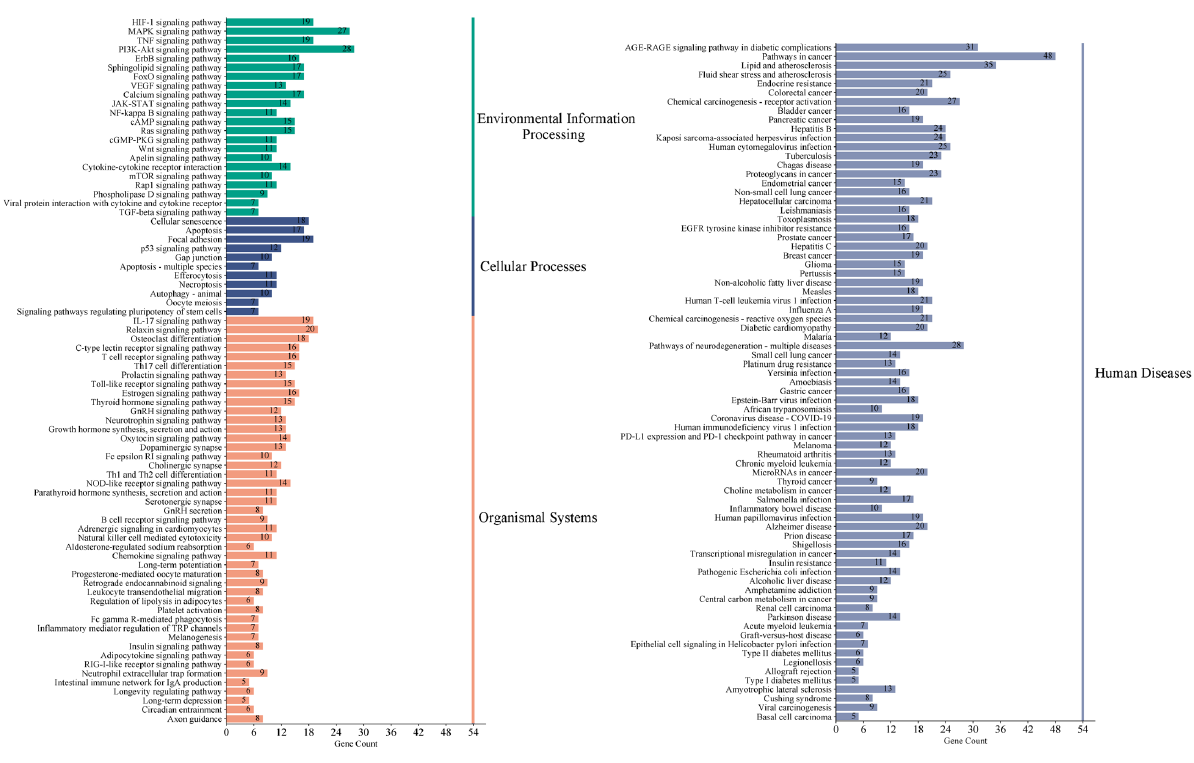
- Classified enriched KEGG pathways in the AOH treatment of depression. The pathways involved were divided into four main categories: cellular activities, organismal systems, processing of environmental information, and human diseases. KEGG: Kyoto encyclopedia of genes and genomes, AOH: Alpinia officinarum hance.
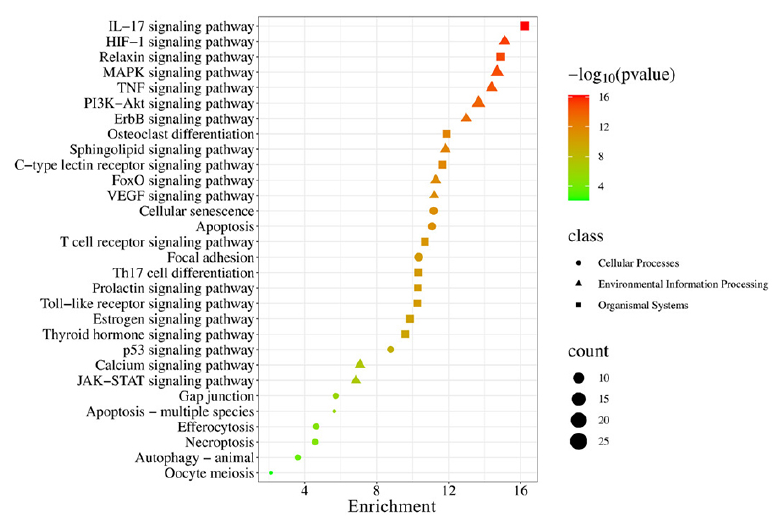
- Top 10 signaling pathways for the class of environmental information processing, organismal systems, and cellular processes in KEGG enrichment analysis. KEGG: Kyoto encyclopedia of genes and genomes.
3.5. Molecular docking
Molecular docking research was conducted between the top six major targets (IL6, AKT1, IL1B, TNF, EGFR, and TP53) and the top five components of AOH (quercetin, kaempferol, sitosterol, medicarpin, and galangin) based on PPI and component-target-pathway network. The docking scores for affinity values between target and compound have been shown in Figure 7 and Figure 8(a). Affinity values < –5 kcal/mol reflected a favorable binding interaction between the drug and the target when evaluating docking effects [20]. The binding energies of the aforementioned target proteins and compounds were less than –5 kcal/mol, suggesting excellent binding activity, according to molecular docking analysis. Figure 8(b) presents the ideal complex structure of the targets and components.
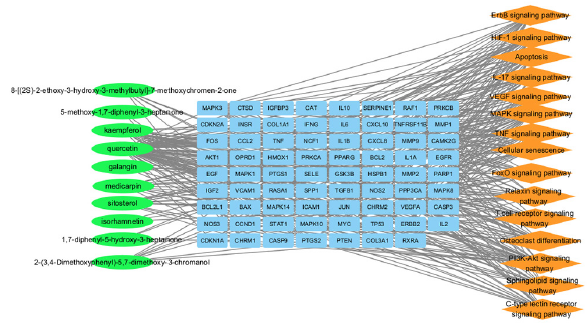
- Component-target-pathway network. Compounds, targets, and pathways are represented as green circles, blue boxes, and orange diamonds, respectively.
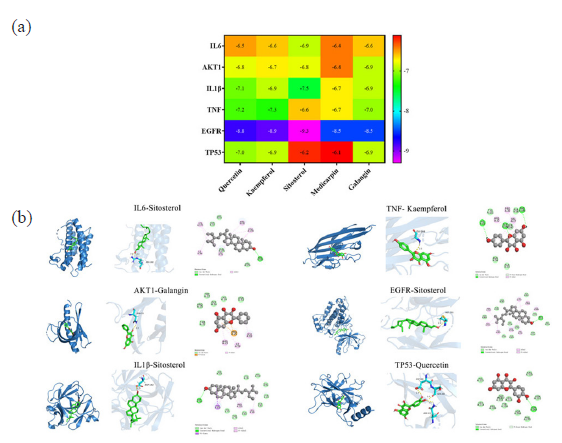
- Molecular docking analysis. (a) The heat map showed values of docking affinity between core targets and active compounds, (b) optimal complex structure of components and targets.
3.6. Protection of AOH extract against CORT-induced PC12 cells
Figure 9 displays the cell viability of PC12 cells. AOH extract had no impact on the viability of PC12 cells at low concentrations, like 16.9, 33.8, and 67.5 μg/mL. With the increase in concentration, the viability of PC12 cells significantly increased but decreased when the concentration reached 500 μg/mL (Figure 9a). The cell viability in the CORT group was significantly reduced to 52.16% (p < 0.0001). After being treated with AOH extract, the viability improved, as shown in Figure 9(b), in comparison to the control group, which had 100% viability. This experiment demonstrated that the AOH extract was non-cytotoxic to PC12 cells and displayed anti-depressant-like effects.
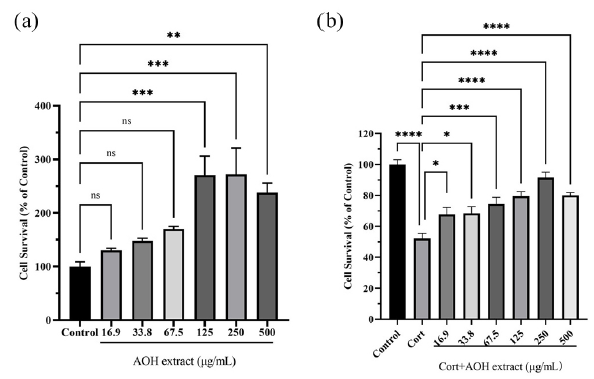
- The impact of (a) AOH extract on PC12 cells viability and (b) the CORT-induced C12 cells. (ns>0.05, *P < 0.05, **P < 0.01, ***P < 0.001 and ****P<0.0001). AOH: Alpinia officinarum hance, CORT: Corticosterone.
PC12 cells are unrelated to the immune system and are unable to generate inflammatory mediators in response to stimulation. As a result, qRT-PCR was used to analyze the expression of AKT1, EGFR, and TP53, three of the top six important targets (Figure 10). The expression of EGFR and TP53 was enhanced by CORT and similarly lowered after AOH extract treatment; however, the expression of AKT1 in the model group significantly decreased and recovered following treatment with AOH extract, as compared to the control group.
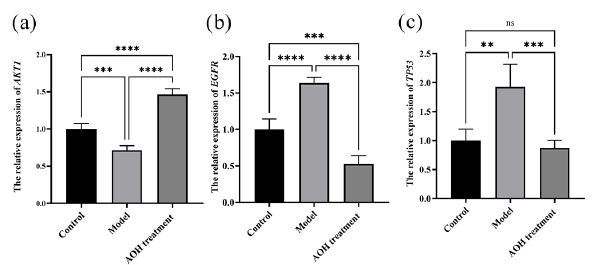
- The AOH extract’s impact on (a) AKT1, (b) EGFR and (c) TP53 mRNA levels (ns > 0.05, **P < 0.01, ***P < 0.001 and ****P < 0.0001).
Furthermore, the impact of AOH extract on apoptotic pathways was investigated using qRT-PCR (Figure 11). The results revealed a significant upregulation in the expression levels of BAX, BCL2, and Caspase3 following CORT treatment. However, AOH extract treatment effectively restored these levels to near-normal. Additionally, the BAX/BCL-2 ratio, which was elevated by CORT, was subsequently reduced by AOH intervention. These findings collectively demonstrate that AOH extract possesses protective properties against CORT-induced apoptotic pathways in cells.
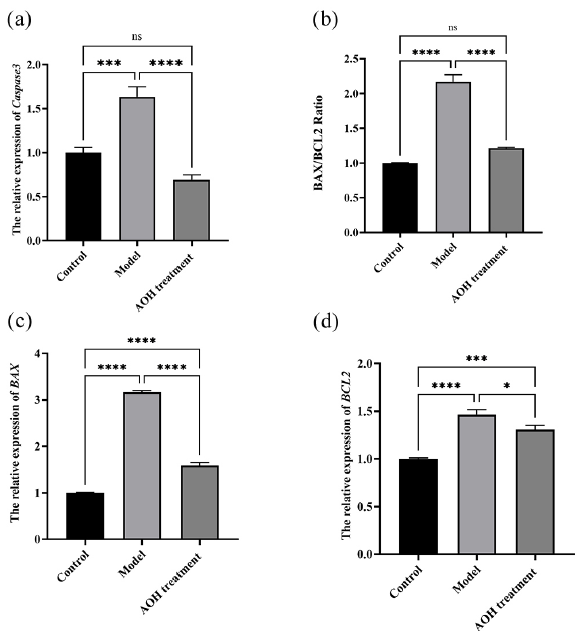
- The effect of AOH extract on apoptotic pathways. (a) Caspase 3 mRNA levels (b) BAX/BCL Radio, (c) BAX mRNA levels, (d) BCL2 mRNA levels (ns>0.05, *P < 0.05, ***P < 0.001 and ****P<0.0001). AOH: Alpinia officinarum hance.
3.7. Discussion
Depression is becoming more widespread worldwide and has a significant negative social and economic impact [21]. The pathogenesis of depression is complex and involves multiple molecular and cellular signaling pathways. However, since it is not a well-understood phenomenon, there are significant barriers to its therapy [22]. Thus, the development of multi-target, multi-pathway, safe, and efficacious anti-depressants is crucial. It has been demonstrated that TCM efficiently treats depression via a variety of targets, components, and pathways [23]. There were 875 herbal medicines related to depression in the HERE database (Table S1). Moreover, the p-value of AOH was 1.080 × 10-24, which was much lower than that of the reported anti-depressant Chinese herb Radix Bupleuri with p-value 3.10 × 10-22 [24], Radix Astragali with p-value 7.44 × 10-9 [25], and Uncaria rhynchophylla with p-value 7.44 × 10-9 [26]. Research on AOH’s anti-depressant effects is limited, and its precise mechanism is not sufficiently explored. In the present research work, AOH’s anti-depressant impact was elucidated using molecular docking and network pharmacology.
From AOH, a total of 10 active components were examined. Through analysis of the component-target-pathway network, it was determined that quercetin, sitosterol, kaempferol, medicarpin, and galangin were the primary active components of AOH since they had the highest number of targets. This indicates that flavonoids may possess a significant anti-depressant impact. By regulating neurotransmitter levels, reducing inflammatory responses, increasing the activity of antioxidant enzymes, lowering oxidative stress indicators, and promoting changes in the HPA axis, quercetin ameliorates depressive-like behaviors [27]. Moreover, the methanol extract of AOH, containing quercetin as a principal active flavonoid, demonstrated notable antidepressant activity. by modulating brain monoamines and normalizing the Hypothalamic - Pituitary - Adrenal (HPA) axis [28]. The anti-depressive benefits associated with kaempferol were demonstrated by its significant antioxidative and anti-inflammatory properties as well as its regulation of several signaling cascades, such as the Akt, p38, and NF-κB pathways [29]. When administered to adult male rats, sitosterol and its derivatives elicited comparable effects to those of anti-depressants via the action of the dopamine (DA), 5-hydroxytryptamine (5-HT), and gamma-aminobutyric acid (GABA)-ergic systems [30]. Quercetin, sitosterol, and kaempferol were also the most promising ingredients in the Chaihu Anxin Capsule, displaying anti-depressant effects [31], which is consistent with the results obtained in the present study. Medicarpin has been reported to improve depressive-like behaviors by upregulating liver x receptor β expression [32]. Galangin, a distinctive constituent of AOH, also has specific neuroprotective properties in the context of depression [33]. In addition to the flavonoids identified in our study, diarylheptanoid compounds, such as 5-methoxy-1,7-diphenyl-3-heptanone and 1,7-diphenyl-5-hydroxy-3-heptanone, have also been reported to exhibit significant neuroprotective effects [34,35]. Furthermore, the PASS Online tool predicted that these compounds possess 5-HT release-stimulating activity (Table S2), which aligns well with the findings discussed above. The PASS Online tool analysis further confirmed that all ten compounds exhibit anti-depressant-related activities (Table S2). These data indicate that AOH may have therapeutic potential in treating depression.
IL6, AKT1, IL1B, TNF, EGFR, and TP53 were identified by the PPI network as potential targets for AOH treatment against depression. The pathophysiology of depression is greatly influenced by inflammatory factors, and individuals with depression have significantly higher levels of pro-inflammatory cytokines [36]. IL6, IL1β, and TNF are crucial targets for AOH in treating depression, as they are all components associated with inflammation. Stress reactions and depressive disorders are significantly influenced by IL-6, a vital cytokine in both peripheral and central systems [37]. It has been suggested that IL1B has a central mediating role in a range of stress-related behavioral activities and may present novel therapy options for treating depression [38]. It has previously been demonstrated that anti-depressant effects and improved anti-depressant response are achieved by anti-TNF-α antagonists [39]. AKT1 is one of the most critical downstream molecules of PI3K. Its activation leads to the phosphorylation of downstream GSK3β, which in turn inactivates GSK3β and prevents the secretion of pro-inflammatory cytokines IL-6, IL-1β, and TNF-α, promoting neurogenesis and neuronal survival [40]. EGFR is a multifunctional glycoprotein widely distributed on the cell membrane of various human tissues. It belongs to the family of receptor tyrosine kinases called epidermal growth factor receptors and is intimately linked to depression-related neuroinflammation [41]. It has been demonstrated that the most distinctive pathways connected to severe depressive disorders brought on by inflammation are the TP53 pathways [42]. Through pathway enrichment analysis, it was discovered that the majority of the targets for AOH’s treatment of depression were associated with the inflammation-related signaling pathways PI3K-Akt, ErbB, TNF, HIF-1, MAPK, and IL-17. The results suggested that AOH’s ability to regulate neuroinflammation may be connected to its anti-depressant effects.
According to the results of molecular docking, depression-related molecules such as IL6, AKT1, IL1B, TNF, EGFR, and TP53 were well-affinized for quercetin, kaempferol, medicarpin, sitosterol, and galangin. The AOH extract was able to restore EGFR, TP53, and IL1B levels in CORT-induced PC12 cells to normal, according to the qRT-PCR data.
The present study has some limitations. The identification of AOH’s active substances has yet to be fully addressed. Thus, more work on thorough content analysis will be required in the future. Secondly, a notable constraint of this research is the absence of in vitro studies on the pure compounds, which could offer deeper insights into their individual roles in the anti-depressant properties of AOH. Furthermore, the examination of the fundamental constituents in the AOH and their ability to mimic drugs may need to be more adequately elucidated, as important targets are only determined through computer simulations and validation with previous studies on comparable pharmaceuticals. Moreover, the precise role of pathways in this study remains ambiguous. Therefore, additional studies are necessary to thoroughly investigate and confirm the diverse pharmacological effects and underlying mechanisms of AOH in the treatment of depression.
4. Conclusions
Using network pharmacology and molecular docking, the current study investigated the possible targets and processes of AOH in the treatment of depression. An in vitro neuroprotective experiment corroborated the findings. Medicarpin, galangin, sitosterol, kaempferol, and quercetin were found to be the possible active ingredients in AOH that can be used to treat depression. AOH has the potential to treat depression by targeting several essential targets, biological mechanisms, and neuroinflammatory pathways. The qRT-PCR results also indicated that the AOH extract could restoring the levels of TP53, EGFR, and IL1B to normal in the CORT-induced PC12 cells. Molecular docking validation further revealed that several core components of AOH could bind stably to the various key targets of depression. The findings suggested that AOH’s ability to regulate neuroinflammation may be connected to its anti-depressant effects. However, a limitation of this study is the lack of in vitro studies on the pure compounds, which would provide further insights into their individual contributions to the anti-depressant effects of AOH. Future studies should focus on isolating and testing the pure compounds identified in this study to elucidate their individual mechanisms of action and potential synergistic effects in treating depression, which can further confirm the antidepressant effect of AOH.
Acknowledgment
The authors would like to thank all the reviewers who participated in the review and MJEditor (www.mjeditor.com) for its linguistic assistance during the preparation of this manuscript. This work was supported by grants from Jining Medical University (the Research Fund for Academician Lin He New Medicine, No. JYHL2022MS16) and Shandong health and Health Committee (the Medical and Health Science and Technology Development Project of Shandong Province, No.202102060559).
CRediT authorship contribution statement
Xiuling Sun: Writing original draft, Methodology, Investigation, Data curation, Formal analysis. Zhiwei Jiang: Investigation, Data curation, Formal analysis. Qihua Wang: Investigation, Visualization. Wenjie Hu: Writing review & editing, Supervision, Funding acquisition, Conceptualization. Litao Zhang: Writing review & editing, Supervision, Project administration, Conceptualization.
Declaration of competing interest
The authors declare that they have no known competing financial interests or personal relationships that could have appeared to influence the work reported in this paper.
Declaration of Generative AI and AI-assisted technologies in the writing process
The authors confirm that there was no use of artificial intelligence (AI)-assisted technology for assisting in the writing or editing of the manuscript and no images were manipulated using AI.
Supplementary data
Supplementary material to this article can be found online at https://dx.doi.org/10.25259/AJC_27_2024.
References
- Virgin coconut oil attenuates lipopolysaccharide-induced depression-like behaviors: Integrating network pharmacology analysis and molecular mechanism evaluation. Neuroscience Letters. 2023;810:137350. https://doi.org/10.1016/j.neulet.2023.137350
- [CrossRef] [PubMed] [Google Scholar]
- Mental health: A world of depression. Nature. 2014;515:181. https://doi.org/10.1038/515180a
- [CrossRef] [PubMed] [Google Scholar]
- Depression. Lancet (London, England). 2018;392:2299-2312. https://doi.org/10.1016/S0140-6736(18)31948-2
- [CrossRef] [PubMed] [Google Scholar]
- Research progress on classical traditional chinese medicine formula baihe zhimu (Lilium lancifolium bulb and anemarrhena asphodeloides rhizome) decoction in the treatment of depression. Heliyon. 2024;10:e25171. https://doi.org/10.1016/j.heliyon.2024.e25171
- [CrossRef] [PubMed] [PubMed Central] [Google Scholar]
- Antidepressant active ingredients from herbs and nutraceuticals used in TCM: Pharmacological mechanisms and prospects for drug discovery. Pharmacological research. 2019;150:104520. https://doi.org/10.1016/j.phrs.2019.104520
- [CrossRef] [PubMed] [Google Scholar]
- Alpinia officinarum. In: Bulbs Roots, Lim T.K., eds. Edible Medicinal and Non-Medicinal Plants: Volume 12 Modified Stems. Cham: Springer International Publishing; 2016. p. :178-195.
- [Google Scholar]
- A review on the ethnomedicinal uses, phytochemistry and pharmacology of alpinia officinarum hance. Journal of Ethnopharmacology. 2018;224:45-62. https://doi.org/10.1016/j.jep.2018.05.027
- [CrossRef] [PubMed] [Google Scholar]
- Anti-inflammatory, anti-nociceptive, and anti-psychiatric effects by the rhizomes of alpinia officinarum on complete freund’s adjuvant-induced arthritis in rats. Journal of Ethnopharmacology. 2009;126:258-264. https://doi.org/10.1016/j.jep.2009.08.033
- [CrossRef] [PubMed] [Google Scholar]
- Research and application of alpinia officinarum in medicinal field. Chinese Herbal Medicines. 2019;11:132-140. https://doi.org/10.1016/j.chmed.2019.04.003
- [CrossRef] [Google Scholar]
- Galangal, the multipotent super spices: A comprehensive review. Trends in Food Science & Technology. 2020;101:50-62. https://doi.org/10.1016/j.tifs.2020.04.032
- [PubMed] [Google Scholar]
- Alpinia officinarum hance: A mini review. Materials Today: Proceedings. 2023;72:3869-3874. https://doi.org/10.1016/j.matpr.2022.10.080
- [CrossRef] [Google Scholar]
- Comparisons of pharmacokinetics and tissue distribution of six major bioactive components of the herbal pair alpinia officinarum-cyperus rotundus in normal and primary dysmenorrhea rats. Journal of Pharmaceutical and Biomedical Analysis. 2024;248:116316. https://doi.org/10.1016/j.jpba.2024.116316
- [CrossRef] [PubMed] [Google Scholar]
- Antidepressant effects of hydroalcoholic extract of alpinia officinarum rhizome on chronic unpredictable stress induced depression in BALB/c mice. Journal of Medicinal Plants. 2020;1:170-179. https://doi.org/10.29252/jmp.1.73.170
- [CrossRef] [Google Scholar]
- Integrated network pharmacology and GC-MS-based metabolomics to investigate the effect of xiang-su volatile oil against menopausal depression. Frontiers in Pharmacology. 2021;12:765638. https://doi.org/10.3389/fphar.2021.765638
- [CrossRef] [PubMed] [PubMed Central] [Google Scholar]
- Uncovering the active components, prospective targets, and molecular mechanism of baihe zhimu decoction for treating depression using network pharmacology-based analysis. Journal of Ethnopharmacology. 2021;281:114586. https://doi.org/10.1016/j.jep.2021.114586
- [CrossRef] [PubMed] [Google Scholar]
- Integrating molecular docking and molecular dynamics simulations. Methods in Molecular Biology (Clifton, N.J.). 2019;2053:13-34. https://doi.org/10.1007/978-1-4939-9752-7_2
- [CrossRef] [PubMed] [Google Scholar]
- Identification of antidepressant constituents from Xiangfu-chuanxiong herbal medicine pair via spectrum-effect relationship analyses, molecular docking and corticosterone-induced PC12 cells. Fitoterapia. 2024;177:106087. https://doi.org/10.1016/j.fitote.2024.106087
- [CrossRef] [PubMed] [Google Scholar]
- TCMSP: A database of systems pharmacology for drug discovery from herbal medicines. Journal of Cheminformatics. 2014;6:13. https://doi.org/10.1186/1758-2946-6-13
- [CrossRef] [PubMed] [PubMed Central] [Google Scholar]
- Comparative toxicogenomics database (CTD): Update 2023. Nucleic Acids Research. 2023;51:D1257-D1262. https://doi.org/10.1093/nar/gkac833
- [CrossRef] [PubMed] [PubMed Central] [Google Scholar]
- Evaluation of autoDock and autoDock vina on the CASF-2013 benchmark. Journal of Chemical Information and Modeling. 2018;58:1697-1706. https://doi.org/10.1021/acs.jcim.8b00312
- [CrossRef] [PubMed] [Google Scholar]
- Combining network pharmacology and experimental verification to reveal the mechanism of Chaigui granules in the treatment of depression through PI3K/Akt/mTOR signaling pathways. Metabolic Brain Disease. 2023;38:2849-2864. https://doi.org/10.1007/s11011-023-01312-5
- [CrossRef] [PubMed] [PubMed Central] [Google Scholar]
- Pathogenesis of depression: Insights from human and rodent studies. Neuroscience. 2016;321:138-162. https://doi.org/10.1016/j.neuroscience.2015.05.053
- [CrossRef] [PubMed] [PubMed Central] [Google Scholar]
- Chinese herbal medicine for the treatment of depression: applications, efficacies and mechanisms. Current Pharmaceutical Design. 2017;23:5180-5190. https://doi.org/10.2174/1381612823666170918120018
- [CrossRef] [PubMed] [Google Scholar]
- Radix bupleuri ameliorates depression by increasing nerve growth factor and brain-derived neurotrophic factor. International Journal of clinical and Experimental Medicine. 2015;8:9205-9217.
- [PubMed] [PubMed Central] [Google Scholar]
- Ethanol extract of mixture of astragali radix and salviae miltiorrhizae radix, alleviates in unpredictable chronic mild stress-induced depression mouse model. IBRO Reports. 2019;6:S198. https://doi.org/10.1016/j.ibror.2019.07.622
- [CrossRef] [Google Scholar]
- Uncaria rhynchophylla ameliorates unpredictable chronic mild stress-induced depression in mice via activating 5-HT1A receptor: Insights from transcriptomics. Phytomedicine: International Journal of Phytotherapy and Phytopharmacology. 2021;81:153436. https://doi.org/10.1016/j.phymed.2020.153436
- [CrossRef] [PubMed] [Google Scholar]
- Role of quercetin in depressive-like behaviors: Findings from animal models. Applied Sciences. 2021;11:7116. https://doi.org/10.3390/app11157116
- [CrossRef] [Google Scholar]
- Evaluation of the ameliorative effect of alpinia officinarum methanol extract in an experimental model of depression. International Journal of Pharmaceutical Sciences Research. 2019;10:1160-1171. https://doi.org/10.13040/IJPSR.0975-8232.10(3).1160-71
- [Google Scholar]
- Flavonols in action: Targeting oxidative stress and neuroinflammation in major depressive disorder. International Journal of Molecular Sciences. 2023;24:6888. https://doi.org/10.3390/ijms24086888
- [CrossRef] [PubMed] [PubMed Central] [Google Scholar]
- The effect of beta-sitosterol and its derivatives on depression by the modification of 5-HT, DA and GABA-ergic systems in mice. RSC Advances. 2018;8:671-680. https://doi.org/10.1039/c7ra11364a
- [CrossRef] [PubMed] [PubMed Central] [Google Scholar]
- A network pharmacology and molecular docking approach to reveal the mechanism of chaihu anxin capsule in depression. Frontiers in Endocrinology. 2023;14:1256045. https://doi.org/10.3389/fendo.2023.1256045
- [CrossRef] [PubMed] [PubMed Central] [Google Scholar]
- Medicarpin improves depressive-like behaviors in a chronic unpredictable mild stress-induced mouse model of depression by upregulating Liver X receptor β expression in the amygdala. Neurotoxicity Research. 2022;40:1937-1947. https://doi.org/10.1007/s12640-022-00610-7
- [CrossRef] [PubMed] [Google Scholar]
- Galangin mitigates DOX-induced cognitive impairment in rats: Implication of NOX-1/Nrf-2/HMGB1/TLR4 and TNF-α/MAPKs/RIPK/MLKL/BDNF. Neurotoxicology. 2022;92:77-90. https://doi.org/10.1016/j.neuro.2022.07.005
- [CrossRef] [PubMed] [Google Scholar]
- Potential neuroprotective effects of alpinia officinarum hance (Galangal): A review. Nutrients. 2024;16:3378. https://doi.org/10.3390/nu16193378
- [CrossRef] [PubMed] [PubMed Central] [Google Scholar]
- Diarylheptanoids with neuroprotective effects from alpinia officinarum rhizomes. Fitoterapia. 2024;175:105980. https://doi.org/10.1016/j.fitote.2024.105980
- [CrossRef] [PubMed] [Google Scholar]
- The role of inflammation in depression: From evolutionary imperative to modern treatment target. Nature reviews. Immunology. 2016;16:22-34. https://doi.org/10.1038/nri.2015.5
- [CrossRef] [PubMed] [PubMed Central] [Google Scholar]
- Role of interleukin-6 in depressive disorder. International Journal of Molecular Sciences. 2020;21:2194. https://doi.org/10.3390/ijms21062194
- [CrossRef] [PubMed] [PubMed Central] [Google Scholar]
- Evidence for IL-1 receptor blockade as a therapeutic strategy for the treatment of depression. Current Opinion in Investigational Drugs (London, England : 2000). 2009;10:664-671.
- [PubMed] [PubMed Central] [Google Scholar]
- Pathogenetic and therapeutic applications of tumor necrosis factor-α (TNF-α) in major depressive disorder: A systematic review. International Journal of Molecular Sciences. 2016;17:733. https://doi.org/10.3390/ijms17050733
- [CrossRef] [PubMed] [PubMed Central] [Google Scholar]
- Resveratrol exerts a protective effect in chronic unpredictable mild stress–induced depressive-like behavior: Involvement of the AKT/GSK3β signaling pathway in hippocampus. Psychopharmacology. 2019;236:591-602. https://doi.org/10.1007/s00213-018-5087-1
- [CrossRef] [PubMed] [Google Scholar]
- Irisin ameliorates the postoperative depressive-like behavior by reducing the surface expression of epidermal growth factor receptor in mice. Neurochemistry International. 2020;135:104705. https://doi.org/10.1016/j.neuint.2020.104705
- [CrossRef] [PubMed] [Google Scholar]
- Transcriptomic signaling pathways involved in a naturalistic model of inflammation-related depression and its remission. Translational Psychiatry. 2021;11:203. https://doi.org/10.1038/s41398-021-01323-9
- [CrossRef] [PubMed] [PubMed Central] [Google Scholar]