Larvicidal potency of four Egyptian herbs on Culex pipiens larvae: Phytochemical composition and molecular networking for most potent extracts
⁎Corresponding authors. asmaey.mostafa.2142@azhar.edu.eg (Mostafa A. Asmaey), seif.eg@gmail.com (Mohamed seif)
-
Received: ,
Accepted: ,
This article was originally published by Elsevier and was migrated to Scientific Scholar after the change of Publisher.
Abstract
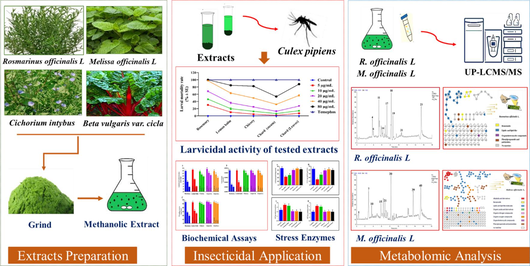
Abstract
Utilizing synthetic insecticides to control mosquito populations has many adverse side effects as they can cause environmental pollution and insecticide resistance. Therefore, the search for eco-friendly and effective mosquito control agents has led to the exploration of plant extracts with larvicidal efficacy. In this study, we aimed to explore the larvicidal activity of four Egyptian plant extracts: Rosmarinus officinalis, Melissa officinalis, Cichorium intybus, and Beta vulgaris var. cicla against Culex pipiens and to identify the chemical constituents present in the active extracts. The methanolic extracts of the four plants were prepared and tested against the third instar larvae of C. pipiens using a standard method. The lethal concentrations (LC50 and LC90) were calculated using probit analysis. The active extracts were subjected to ultra-performance liquid chromatography-mass spectroscopy (UPLC-MS/MS) analysis and the data were processed using the global natural products social molecular networking (GNPS) platform to generate molecular networks and identify the compounds based on spectral similarity and database matching. The methanolic extracts of R. officinalis L. and M. officinalis L. had the highest larvicidal activity, where LC50 values were 9.795 and 26.505 μg/mL, respectively. Their exposure caused a high mortality rate and disrupted the biochemical and physiological parameters (total carbohydrates, total lipids, total protein, acetylcholinesterase (AChE), and glutathione S-transferase (GST) in the body of C. pipiens when compared with Cichorium intybus (Chicory) and Beta vulgaris var. cicla (Chard). The UPLC-MS/MS analysis with the aid of the GNPS platform, revealed the presence of 23 and 41 metabolites from R. officinalis L. and M. officinalis L., respectively. The identified metabolites may act as larvicidal agents by interfering with the physiological or biochemical processes of the mosquito larvae. Overall, the findings suggest that the methanolic extracts of R. officinalis and M. officinalis are potential sources of natural larvicides for mosquito control.
Keywords
Culex pipiens
Egyptian plants
Larvicidal activity
Melissa officinalis
Rosmarinus officinalis
UPLC/MS
1 Introduction
Mosquitoes are vectors of many pathogens that affect millions of people and animals worldwide, There are more than 3500 mosquito species that have been identified worldwide (Robert, 2001). In 2019, several outbreaks of dengue fever were witnessed in Egypt, with over 12,000 cases reported (Gaber et al., 2022). Moreover, many arboviral diseases, such as Zika virus and chikungunya are prevalent and their spread is rising (Tiwari et al., 2020).
Furthermore, mosquitoes can carry and spread the Rift Valley fever virus, West Nile virus, and filariasis (Conley et al., 2014), as well as other diseases such as dengue and malaria that result in significant mortality and economic loss, especially in developing countries where the resources for prevention and treatment are limited (Onen et al., 2023). Culex spp. is one of the most widespread vectors in Egypt (Haba and McBride 2022). Moreover, C. pipiens may affect food safety particularly dairy and meat products, by transmitting and spreading microbial contamination. This can occur not only through mechanical methods but biologically as well (Adly et al., 2022; Abdel-Haleem et al., 2024).
Controlling mosquito populations has become one of the most effective strategies to prevent the transmission of many vector-borne diseases and food contamination. Particularly, larviciding is a fruitful way of reducing the mosquito population in their breeding places before they emerge from the larval stage (Karunaratne and Surendran 2022).
Commonly, the traditional procedures of mosquito control, which mainly involve the use of synthetic insecticides, have several limitations due to their adverse effects on the environment, food chain, human and animal health, and insecticide resistance (Rani et al., 2021; Abbasi et al., 2022; Tantawy et al., 2022; Onen et al., 2023). Therefore, the search for safe and effective mosquito control agents became obligatory and led to the exploration of plant-based natural products that may substitute conventional pesticides and may act as insecticides, antifeedants, and repellents (Abdel-Haleem et al., 2024).
Plants have been used for centuries for insect control, and their insecticidal properties are well known. Recently, several plant species have been reported to have larvicidal activity against mosquitoes, including Culex pipiens, Culex antennas, and Aedes aegypti (Shehata, 2019; Shahat et al., 2020; Assemie and Gemeda, 2023; Priya et al., 2023; Farag et al., 2024). Due to the variety of the Egyptian climate, the Egyptian flora is widely diverse and a rich source of natural molecules (Asmaey et al., 2024; Al-Tohamy et al., 2018; El-Seedi et al., 2021; Elsoghiar et al., 2024). For instance, previous studies have reported that the essential oils of Melissa officinalis and Rosmarinus officinalis have shown promising larvicidal activity against different mosquito species (Conti et al., 2010; Ebadollahi et al., 2020; Zeghib et al., 2020; Ramzi et al., 2022; Khater et al., 2023). However, the larvicidal activity of plant extracts grown in Egypt has not been extensively evaluated.
Acetylcholinesterase (AChE) and glutathione S-transferase (GST) are key enzymes in insect control strategies and are among the most common detoxification enzymes. Evaluating their role is important to understand the effect of tested materials. The AChE enzyme catalyzes the hydrolysis of the neurotransmitter in the nervous system which is impacted by synthetic and botanical insecticides (Zibaee et al., 2009; Oni et al., 2019). The GST enzyme plays a crucial role in detoxification and cellular antioxidant defenses against oxidative stress, including insecticides, through its conjugation of reduced glutathione to the electrophilic sites of both natural and synthesized exogenous xenobiotics (Enayati et al., 2005; BK et al., 2022).
Therefore, this study aimed to explore and compare the efficiency of four plant extracts from Egypt as biopesticides to potentially replace synthetic insecticides for controlling C. pipiens, a common mosquito vector that can transmit several diseases. The tested plants namely; Rosmarinus officinalis (Lamiaceae family), also known as “alecrim,” is a perennial herb native to the Mediterranean region. Melissa officinalis (lemon balm) is a crucial herb among the autochthonous medicinal plants of the genus Melissa. Cichorium intybus L., commonly known as chicory, is a medicinally important plant in Eurasia and parts of Africa. Beta vulgaris var. cicla (chard) was selected based on its wide application in traditional medicine and culinary purposes in many countries, including Egypt. Moreover, the study sheds light on the investigation of the metabolic profile of the most effective extracts against the larvae of C. pipiens using UPLC-MS/MS analysis with the aid of GNPS molecular networking to explain their effects as bio-insecticides.
2 Materials and methods
2.1 Plant material
The aerial parts of the tested plants (Table 1) were collected from Al-Azhar Agricultural Research Nursery for Trees and Plants, Al Walideyah, Asyut 2, (27°11′55.4″N 31°10′26.1″E), Assiut Governorate, Upper Egypt in 2022. The plants were identified (Belhumeur et al., 2008) and stored at the chemistry department of the Faculty of Science, Al-Azhar University (with serial numbers CHM103, CHM104, CHM105, and CHM106).
Scientific name | Common name | Time of collection | Herbarium Code |
---|---|---|---|
Rosmarinus officinalis L. | Rosemary | June 2022 | CHM103 |
Melissa officinalis L. | Lemon balm | July 2022 | CHM104 |
Cichorium intybus | Chicory | August 2022 | CHM105 |
Beta vulgaris var. cicla | Chard | June 2022 | CHM106 |
2.2 Plant extraction
The collected plants (1000 gm from each) were cleaned, dried, and ground to a fine powder (250 gm as the ground yield of each plant) and kept in a dark place at room temperature. A 200 g portion of the fine powder of rosemary and lemon balm was hydrodistilled over 120 min by using a Clevenger apparatus resulting in the isolation of 0.230 gm and 0.620 gm of essential oils, respectively. Then the dried residue remaining after hydrodistillation was extracted three times with 2 L of methanol for 60 min in an ultrasonic bath (Elsoghiar et al., 2024); the solvent was evaporated completely under reduced pressure until dryness to afford 37.8 and 40.6 gm of extract, respectively.
For chicory, and chard (stems and leaves), 200 g of the fine powder were directly extracted three times with 2 L of methanol for 60 min in an ultrasonic bath. The resulting solvent was evaporated under reduced pressure until dryness. The yield of the chicory extract was 25.4 gm, whereas the chard extracts from stems and leaves were 31.4 and 27.2 gm, respectively. The crude extracts of the four plants were subjected to larvicidal evaluation. To evaluate the phytochemical analysis, the extracts were further fractionated and defatted with n-hexane. Then, the polar and non-polar fractions were kept at 4 °C until use.
2.3 Larvicidal activity evaluation of the different extracts
2.3.1 Mosquito rearing
The mosquito, C. pipiens larvae was acquired from the Insectary of Medical Entomology, Animal House, Al-Azhar University, Cairo, Egypt, where it was established there since 2018. Then, we reared it for several generations to obtain enough progeny for current experimental studies. The colony was reared under controlled conditions of temperature of 27 ± 2 °C, and relative humidity of 75 ± 5 % with a (light: dark = 14 h:10 h). regimen. The emerged adults were transferred to wooden cages (30 × 30 × 30 cm) supplied with a 10 % sucrose solution for development and 500 mL plastic cups containing distilled water for hatching. The females' blood meal source was the pigeons' blood used to stimulate reproductive and developmental purposes.
2.3.2 Larvicidal activity of the tested herbal extracts
The larvicidal activity of the tested extracts was assessed against the mosquito vector, C. pipiens using the procedures of the World Health Organization bioassay (WHO, 2005). Briefly, larvae were reared under laboratory conditions of a temperature of 27 ± 2 °C, and relative humidity of 75 ± 5 % with a constant photoperiod (light:dark = 14 h:10 h). Then, 25 early 3rd instar larvae were transferred to polyethylene disposable cups (500 mL) containing 249 mL distilled water + 1 mL of test concentration solubilized in dimethyl sulphoxide (DMSO). The larvae were then exposed to different ranges of concentrations of the tested extracts, 5, 10, 20, 40, and 80 μg/mL for the rosemary and lemon balm, while at 25, 50, 100, 200, and 400 μg/mL for the chicory, stem chard, and leaves chard extracts.
The negative control was tested under the same conditions without toxicants with the same amount of DMSO, while temephos (1 μg/mL) was used as a positive control. The mortality was recorded 24 h after exposure, and each concentration was tested in five replicates.
2.4 Biochemical assays
For biochemical assays, a hundred first-instar C. pipiens larvae were collected 3 days post-treatment with the LC50 and LC90 concentrations of each tested extract alongside the untreated (control) group. Treatment was done in triplicates. The collected larvae were then homogenized in distilled water using a Teflon homogenizer that had been dipped in a jacket of crushed ice. The homogenization process was carried out to ensure a thorough mixture. Subsequently, the samples were centrifuged in a cooling centrifuge at 4427 RCF for 10 min, and the supernatant was collected for further biochemical assays.
2.4.1 Determination of total carbohydrates
Total carbohydrate content was determined using the phenol–sulfuric acid reaction method (DuBois et al., 1956). Total carbohydrates were then prepared for bioassay following the procedures outlined by (Crompton and Birt, 1967).
2.4.2 Determination of total lipids
Total lipid content was determined by the scheme of using phospho-vanillin reagent (Knight et al., 1972) (prepared by dissolving 0.6 g pure vanillin in 10 mL ethanol and filled to 100 mL with distilled water), then 400 mL concentrated phosphoric acid was added.
2.4.3 Determination of total proteins
Total protein content was determined according to the method of Bradford M. (Bradford 1976).
2.4.4 Activity of the acetylcholinesterase (AChE) and glutathione S-transferase (GST) enzymes
Acetylcholinesterase activity was evaluated using acetylcholine bromide as a substrate according to the method briefly described by Simpson D (Simpson et al., 1964). The activity of glutathione S-transferase was evaluated using 1-chloro-2,4-dinitrobenzene (CDNB) as a substrate according to the method briefly described (Kao et al., 1989).
2.5 Phytochemical analysis of only the most effective polar extracts
2.5.1 UPLC–MS/MS analysis
UPLC is accomplished through the use of a narrower column filled with particles typically less than 2 µm in diameter. To gain quick insight into the components, recording a mass spectrum of each component as it elutes from the LC column proves highly beneficial. For efficient online LC/MS, ESI-MS and LC are usually interfaced.
In this study, the defatted extract of rosemary and lemon balm was prepared and injected into the UPLC/ESI-MS apparatus after being dissolved in H2O/MeOH mixtures. The UPLC analysis was carried out using a Dionex Ultimate 3000 UPLC system (Sunnyvale, CA, USA: Thermo Scientific™Dionex™), and a reverse-phased Hypersil Gold UPLC C18 column (2.1 × 150 mm, 1.9 µm) was employed, following a method previously reported (Asmaey et al., 2023; Nazeh et al., 2024).
2.5.1.1 Molecular networking (MNW)
The online workflow (https://ccms-ucsd.github.io/GNPSDocumentation) on the GNPS website (https://gnps.ucsd.edu) was used to design a molecular network. All MS/MS fragment ions within ±17 Da of the precursor m/z were removed from the data. MS/MS spectra were window-filtered throughout the spectrum by selecting only the top 6 fragment ions in the ±50 Da window. The MS/MS fragment ion tolerance was set at 0.5 Da and the precursor ion mass tolerance was set to 2.0 Da. The edges of the network were then filtered to have a cosine score greater than 0.7 and more than 6 matching peaks. Edges between two nodes were also preserved in the network if and only if both nodes featured in each other's top 10 most similar nodes.
The MNW of our work is (ProteoSAFe/status.jsp?task=354d7a818fc849e5882b48b50c553788) for rosemary and (ProteoSAFe/status.jsp?task=3fc656c21ad54cd6811238ce033debb8) for lemon balm scanning. To improve chemical structural data within the molecular network, information from in silico structure annotations from GNPS Library Search was integrated into the network using the GNPS MolNetEnhancer workflow (https://ccms-ucsd.github.io/GNPSDocumentation/molnetenhancer) on the GNPS website (http://gnps.ucsd.edu).
Chemical class interpretations were performed using the ClassyFire chemical ontology (Djoumbou Feunang et al., 2016; Ernst et al., 2019). The GNPS spectral libraries and molecular network visualization software (Cytoscape, version 3.9.1) were also used.
2.6 Statistical analysis
Descriptive analysis including Mean ± Standard Error (SE) was calculated for each treatment. Larval mortality was subjected to probit analysis to determine, LC50, and LC90 with confidence limits of 95 %. Analysis of variance, lower and upper confidence limits, and chi-squared values were performed using SPSS (IBM SPSS ver. 25). The LSD post-hoc method was used for pairwise comparisons, while, the P value was considered significant at p < 0.05.
3 Results
3.1 The larvicidal activity of tested extracts
Data presented in Fig. 1 show the larvicidal activity of tested extracts; rosemary, lemon balm, chicory, and chard (leaves and stems) against the 3rd instar larvae of C. pipiens. The larval mortality results showed a concentration-dependent pattern as larval mortality increased when the concentration increased. Additionally, the obtained data showed varying levels of mortality with different tested extracts with LC50 values ranging from 9.795 μg/mL for rosemary extract to 363.093 μg/mL for chard stem extract. Based on the obtained LC50 values, tested extracts may be arranged in descending order as the following: rosemary extract and lemon balm extract then chard leaves extract and chicory extract, and last chard stem extract with LC50 values of 9.795, 26.505, 150.867, 179.128, and 363.093 μg/mL, respectively.
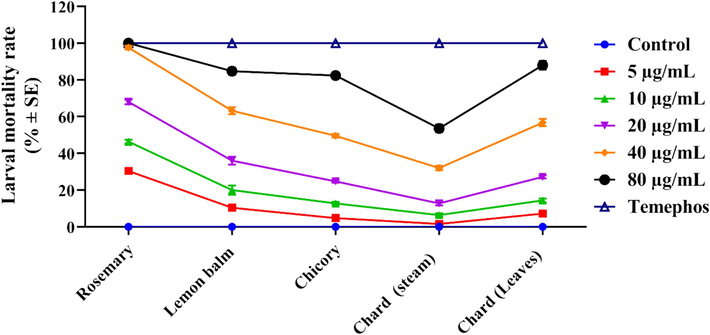
- Larvicidal activity of different concentrations of the rosemary, lemon balm, chicory, chard (stem), and chard (leaves) extracts against C. pipiens. Larval mortalities are presented as Mean ± SE, (n = 5). Temephos are used as appositive control.
Overall, the tested extracts exhibited moderate to high toxicity particularly rosemary and lemon balm extracts which showed pronounced larvicidal activity. These extracts can effectively control C. pipiens mosquitoes at the larval stage. The current findings indicate that the most potent extract against the mosquito larvae was rosemary extract which demonstrated absolute larvicidal action with a toxicity index of 100 % when applied at a concentration of 80 μg/mL (Fig. 1).
3.2 Impact of LC50 and LC90 concentrations of the tested extracts on the total carbohydrate, lipid, and protein contents of the C. pipiens larvae
Additionally, the data presented in Fig. 2 shows the effect of treatment with the LC50 and LC90 concentrations of the tested extracts on the total carbohydrate, lipid, and protein contents of the C. pipiens larval tissue homogenate. For total carbohydrate content, the rosemary and lemon balm extracts were the most effective, significantly reducing the total carbohydrate content. The LC90 concentration caused a decrease in the total carbohydrate content to less than 4.0 mg/g in the tissues homogenate of C. pipiens larvae, compared to its content of 16.3 mg/g in the control group (Fig. 2A). Similarly, rosemary and lemon balm extracts also reduced the total lipid contents to reach about 0.0002 mg/g against 0.0009 mg/g for the larval tissue homogenate of the control group following the exposure to LC90 concentration (Fig. 2B). The other tested extracts revealed almost the same pattern of weak effectiveness toward larval lipid profile even when the LC90 concentrations of those extracts were applied.
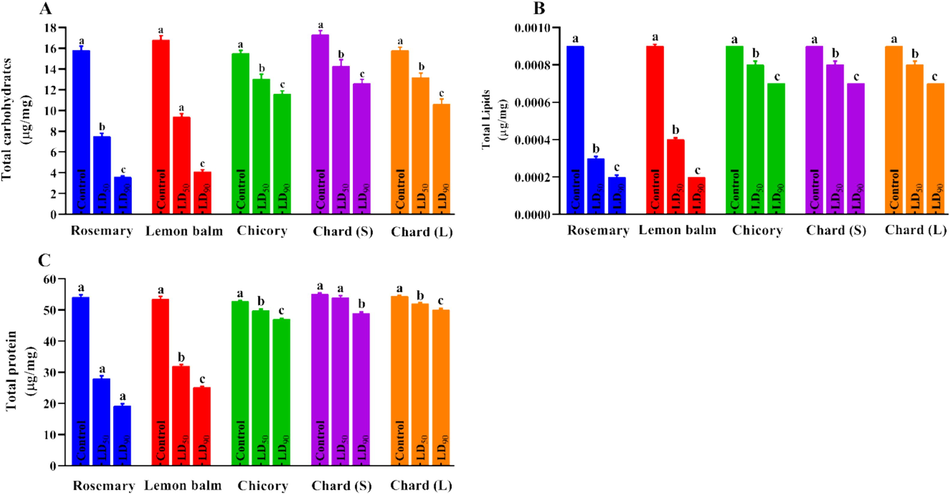
- Effect of LC50 and LC90 of the tested extracts on the total carbohydrate (A), total lipid (B), and total protein (C) contents of the C. pipiens larval tissue homogenate 3 days following exposure. Data illustrated as Mean ± SE, (n = 3). Chard (s) = chard stem; Chard (L) = chard leaves. Different letters are significantly different between the treated and control groups (P < 0.05).
The total protein contents were reduced when lethal concentrations of these four extracts were applied, with very slight activity recorded for other tested extracts. The total protein contents were reduced to 19.2 and 25.1 mg/g of the C. pipiens larval tissue homogenate treated with the LC90 concentrations of rosemary and lemon balm extracts, respectively compared to 54.5 mg/g in the control group (Fig. 2C).
3.3 Impact of LC50 and LC90 concentrations of the tested extracts on the activity of AChE and GST and of the C. pipiens larvae
Data illustrated in Fig. 3 disclose the outcomes of the effects of LC50 and LC90 concentrations of different tested extracts on the activity of acetylcholinesterase in the C. pipiens larvae after exposure. The obtained findings revealed different patterns of enzymatic activity among tested extracts. The AChE activity was reduced to 2 to 3-fold after treatment with the LC50 concentrations of rosemary and lemon balm, and this reduction became much more pronounced in those larvae exposed to the LC90 concentrations, while other extracts exert comparable activity to the control group.
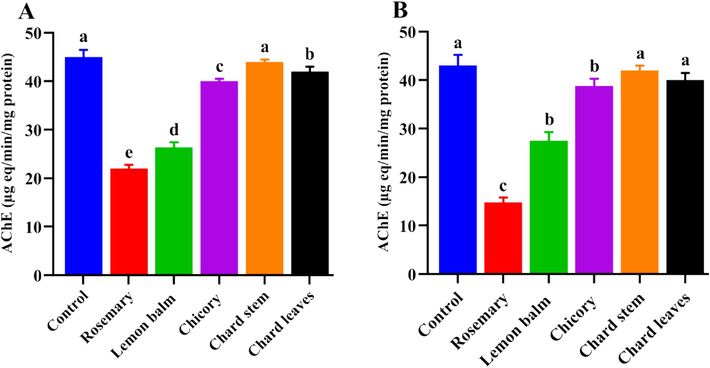
- Effect of lethal concentrations, LC50 (A), and LC90 (B) of different tested extracts on the enzymatic activity of Acetylcholinesterase (AChE) in the third instar larvae of the C. pipiens evaluated 3 days post-exposure. Data presented as Mean ± SE, (n = 3). Different letters are significantly different between the treated and control groups (P < 0.05).
Otherwise, the GST enzyme activity was moderately increased under exposure to rosemary and lemon balm extracts compared to the control larvae. However, chicory and stem and leaves chard extracts did not have significance compared to the untreated larvae when applied for either AChE or GST enzyme activity evaluation (Fig. 3 and Fig. 4). Importantly, based on the larvicidal potency, the methanolic extracts of rosemary and lemon balm had the lowest LC50 values of 9.795 and 26.505 μg/mL, respectively, indicating high larvicidal influence. According to the obtained results, it became important to identify the kinds of natural constituents present in the extracts that showed the most effectiveness, “rosemary and lemon balm”. Recently, the analytical tools specifically the liquid chromatography (LC) connected with masses detectors (MS) gave an obvious image of the precursor's masses that were characterized for specific natural compounds.
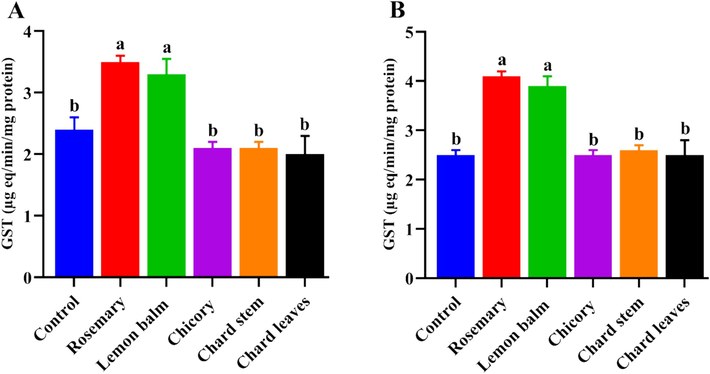
- Effect of tested lethal concentrations, LC50 (A), and LC90 (B) of different tested extracts on the enzymatic activity of Glutathione S-transferase (GST) in the third instar larvae of the mosquito, C. pipiens evaluated 3 days post-treatment. Data presented as Mean ± SE, (n = 3). Different letters are significantly different between the treated and control groups (P < 0.05).
In this context, we performed UPLC-MS/MS with the aid of molecular networking through the GNPS platform to explain the biological activity response depending on (Fig. 5 and Fig. 6; Table 2 and Table 3).
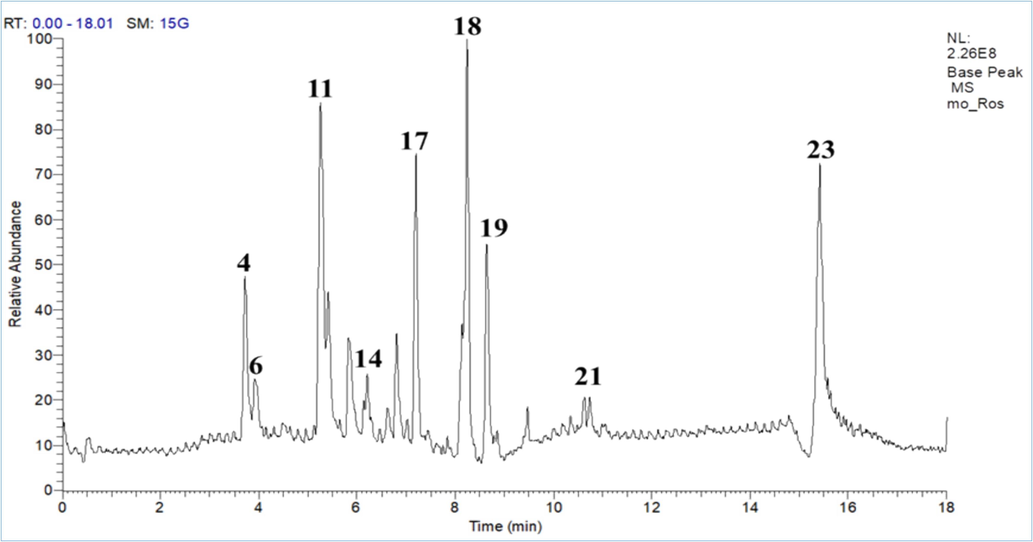
- Base peak chromatogram of R. officinalis L. leaves extract.
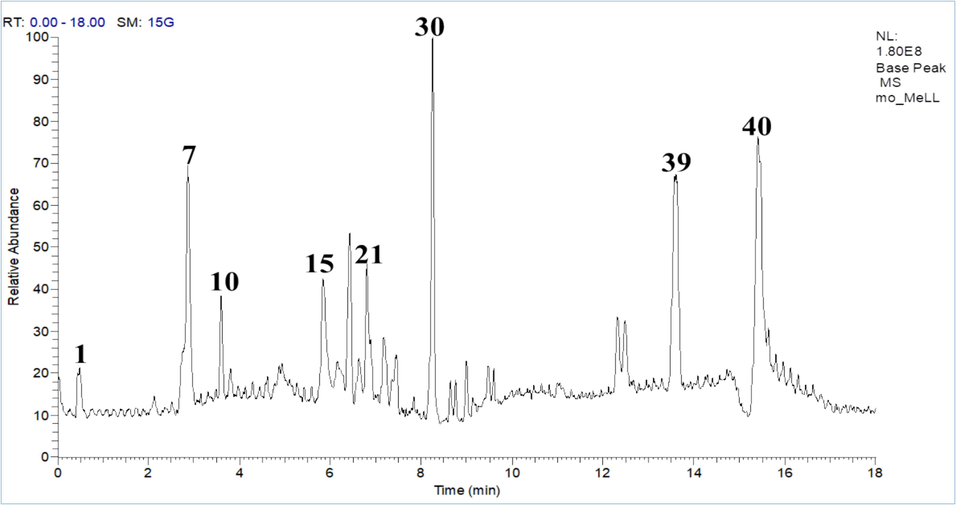
- Base peak chromatogram of M. officinalis L. leaves extract.
NO. | Rt (min) | Precursor mass (− or +) | Fragments | Compound Name | Chemical Class | Ref. |
---|---|---|---|---|---|---|
1 | 0.19 | 191 | 147, 127, 103, 93, 87 | Quinic acid | Cyclohexanecarboxylic acid | (Borrás-Linares et al., 2014) |
2 | 2.83 | 477 | 315, 299, 285 | Cirsimarin | Flavone glycoside | |
3 | 3.07 | 461 | 283, 297 | Homoplantaginin | Flavonoid glycoside | (Borrás-Linares et al., 2014) |
4 | 3.72 | 345 | 301, 283 | Rosmanol | Diterpene lactone | (Borrás-Linares et al., 2014) |
5 | 3.84 | 329 | 314, 285, 268 | Tri-hydroxy-di-methoxyflavonol | Flavonoid | |
6 | 3.91 | 345 | 301, 283 | Rosmanol isomer | Diterpene lactone | (Borrás-Linares et al., 2014) |
7 | 4.29 | 331 | 313, 283, 285, 287, 269 | Carnosic acid | Benzenediol abietane diterpene | (Borrás-Linares et al., 2014) |
8 | 4.47 | 313 | 283, 298 | Cirsimaritin | Flavonoid | (Borrás-Linares et al., 2014) |
9 | 5.26 | 359 | 283, 300, 329 | Rosmanol methyl ether | Diterpene lactone | (Borrás-Linares et al., 2014) |
10 | 5.41 | 299 | 284, 227, 137 | Diosmetin | Methylated flavone | (Velamuri et al., 2020) |
11 | 5.56 | 329 | 314, 285, 268 | Tri-hydroxy-di-methoxyflavonol | Diterpenoid | Asmaey, MA. 2023 |
12 | 5.83 | 343 | 315, 299, 269, 243 | Rosmadial | Phenolic diterpene lactone | (Borrás-Linares et al., 2014) |
13 | 6.07 | 283 | 268 | Genkwanin | Methylated flavone | (Borrás-Linares et al., 2014) |
14 | 6.21 | 315 | 285 | Rosmaridiphenol | Diphenolic diterpene | (Borrás-Linares et al., 2014) |
15 | 6.73 | 359 | 283, 329, 300 | Epirosmanol methyl ether | Diterpene lactone | (Borrás-Linares et al., 2014) |
16 | 6.82 | 487 | − | Asiatic acid | Pentacyclic triterpenoid | (Borrás-Linares et al., 2014) |
17 | 7.21 | 345 | 301, 287 | 12-Methoxy-carnosic acid | Benzenediol abietane diterpene | (Borrás-Linares et al., 2014) |
18 | 8.50 | 453 | 407 | Micromeric acid | Triterpenoid | (Borrás-Linares et al., 2014) |
19 | 8.62 | 391 | 207, 181, 149, 113 | Bis(2-ethylhexyl) phthalate | Phthalate ester | Nasr et al., 2018 |
20 | 8.69 | 455 | − | Betulinic acid | Pentacyclic triterpenoid | (Borrás-Linares et al., 2014) |
21 | 10.73 | 311(+) | 161, 135 | Avobenzone | Dibenzoyl methane derivative | GNPS |
22 | 11.18–12.69 | 282.27 (+) | 265, 247, 149, 135 | 9-Octadecenamide | Fatty amides | GNPS |
23 | 15.42 | 305 | 248, 174.9, 159, | 3-(2-Carboxyethylpentasulfanyl) propanoic acid | Fatty acid derivatives | GNPS |
m/z: Precursor mass, (−): negative ion,(+): positive ion
No. | Rt (min) | Precursor mass (− or + ) | Fragments | Compound Name | Chemical Class | Ref. |
---|---|---|---|---|---|---|
1 | 0.48 | 387 | 341, 179 | Hydroxyjasmonic acid glucoside | hydroxyjasmonic acid glucoside | (Ben et al., 2020) |
2 | 1.82 | 137 | 93 | 4-Hydroxybenzoic acid | monohydroxybenzoic acid | (Miron et al., 2013) |
3 | 1.83 | 153 | 123, 109 | Protocatechuic acid | dihydroxybenzoic acid | (Miron et al., 2013, Wang et al., 2015) |
4 | 2.26 | 179 | 161, 135, 121 | Caffeic acid | Phenolic acid | (Wang et al., 2015) |
5 | 2.38 | 151 | 136, 109 | Vanillin | organic compound | (Tsiaka et al., 2022) |
6 | 2.74 | 521 | 359, 197, 179, 161 | Rosmarinic acid hexoside | hydroxycinnamic acid ester glycoside | (Silva et al., 2023) |
7 | 2.87 | 359 | 197, 179, 161 | Rosmarinic acid | hydroxycinnamic acid ester | (Ben et al., 2020) |
8 | 2.88 | 719 | 359, 161 | Sagerinic acid | cyclobutane lignans | (Ben et al., 2020) |
9 | 2.94 | 193 | 178, 149, 134 | Ferulic acid | hydroxycinnamic acid | (Wang et al., 2015) |
10 | 3.60 | 205 | 179, 162, 161, 133 | Caffeic acid vinyl ester | hydroxycinnamic acid derivative | |
11 | 3.87 | 353 | 191 | Chlorogenic acid | Phenolic acid | (Tsiaka et al., 2022) |
12 | 4.33 | 395 | 197 | Dimer (3,4-dihydroxyphenyl) lactic acid | hydroxy monocarboxylic acid | (Miron et al., 2013) |
13 | 4.87 | 345 | 330, 315, 300, 287 | Rosmanol | Diterpene lactone | (Gürbüz et al., 2019) |
14 | 4.93 | 593 | 285 | Kaempferol-3-O-rutinoside | flavonol glycoside | |
15 | 5.90 | 487 | 469, 443, 305 | 3,6,19-trihydroxy-Urs-12-en-28-oic acid | Pentacyclic triterpenoid | GNPS |
16 | 6.16 | 301 | 301, 283, 185, 229, 257 | Sternbin | Flavanone | GNPS |
17 | 6.18 | 377 | 329, 309, 265 | 1-decoxy-1,4-dioxo-4-[(E)-prop-1-enoxy]butane-2-sulfonic acid | GNPS | |
18 | 6.21 | 455 (+) | 409, 391, 265, 191 | Micromeric acid | Pentacyclic triterpenoid | GNPS |
19 | 6.43 | 293 | 276, 275, 257, 197 | 13-Hydroxy-9,11,15-octadecatrienoic acid | unsaturated hydroxy fatty acid | GNPS |
20 | 6.63 | 325 | 197, 191, 183, 147 | 10-(4-Sulfophenyl)decanoic acid | Organic Fatty acid derivative | GNPS |
21 | 6.84 | 507 | 279, 153 | 16-Acetyloxy-3,11-dihydroxy-4,8,10,14-tetramethyl-9,11,15,16-tetrahydro-3H-cyclopenta[a]phenanthren-17-ylidene]-6-methylhept-5-enoic acid | GNPS | |
22 | 6.90 | 555 | 493, 359, 225 | Salvianolic acid K isomer | Lignans | (Silva et al., 2023) |
23 | 7.18 | 345 | 330, 315, 299, 286 | Rosmanol | Diterpene lactone | (Gürbüz et al., 2019) |
24 | 7.21 | 399 | 353, 191, 179, 135 | CQA ester | Phenolic acid ester | (Liu et al., 2022) |
25 | 7.37 | 473 (+) | 455, 427, 409, 249 | Maslinic acid (2-hydroxyoleanolic acid) | pentacyclic triterpenoid | GNPS |
26 | 7.44 | 455 (+) | 409, 391, 265, 191 | Micromeric acid isomer | Pentacyclic triterpenoid | GNPS |
27 | 7.59 | 561 | 515, 399, 353, 191, 179, 161, 133 | diCQA ethyl ester | Phenolic acid ester | (Liu et al., 2022) |
28 | 7.67 | 677 | 515 | CQA hexosyl hexoside | Phenolic acid glycoside | (Liu et al., 2022) |
29 | 7.85 | 325 | 257, 216, 197, 183, 163 | 10-(4-Sulfophenyl)decanoic acid | Organic Fatty acid derivative | GNPS |
30 | 8.28 | 457(+) | 439. 411, 407 | Ursolic acid | pentacyclic triterpenoid | GNPS |
31 | 8.52 | 609 | 577, 551, 489 | Penta methoxy dihydro biapigenin | Biflavonoid | (Emam et al., 2021) |
32 | 8.65 | 391 | 331, 313, 279, 167, 149 | 6-Hydroxylithocholic acid | Steroid | GNPS |
33 | 8.74 | 477 | 431. 345, 264 | 3,4-dihydroxy-2-methyloctadecoxy]-6-(hydroxymethyl)oxane-3,4,5-triol | GNPS | |
34 | 8.99 | 593 (+) | 533, 431 | ND | ||
35 | 9.60 | 535 | 507, 447, 405,300 | longirostreroneA | organic heterotricyclic compound | GNPS |
36 | 12.34 | 309 | 267, 255, 215, 209, 146, 157, 97 | 1-[2-methyl-6-[(2S,3R,4S,5S,6R)-3,4,5-trihydroxy-6-(hydroxymethyl)oxan-2-yl]oxyphenyl]ethanone | GNPS | |
37 | 12.49–14.84 | 339 | 275, 265, 183 | [5-Acetyloxy-3-(hydroxymethyl)-2-oxo-6-propan-2-ylcyclohex-3-en-1-yl] 3-methylpentanoate | GNPS | |
38 | 12.60 | 325 | 265, 239, 216, 197, 189, 183 | 10-(4-Sulfophenyl) decanoic acid | Organic Fatty acid derivative | GNPS |
39 | 13.60 | 282 | 265, 247 | Oleic acid | Fatty acid | GNPS |
40 | 15.40 | 305 | 299, 191, 179, 162, 147 | 3-(2-Carboxyethylpentasulfanyl)propanoic acid | GNPS | |
41 | 16.14 | 327 | 283, 269, | (10E,15E)-9,12,13-trihydroxyoctadeca-10,15-dienoic acid | Fatty acid | GNPS |
3.4 Metabolomic profiling of the most active extracts using UPLCMS/MS analysis
The phytochemical analysis of R. officinalis L. leaves extract (Fig. 5 and Table 2) exploited 23 metabolites that represented different classes of compounds including, benzoic acid derivatives, lipids of triterpenes derivatives, and methylated flavonoids that represented the most three classes, as well as fatty acids derivatives (Fig. 7). On the other hand, the phytoconstituents of M. officinalis L. leaves extract (Fig. 6 and Table 3) introduced 41 compounds arranged in different classes such as steroidal derivatives, flavonoids and their glycosides derivatives, phenolic acid derivatives, and fatty acid derivatives (Fig. 8).
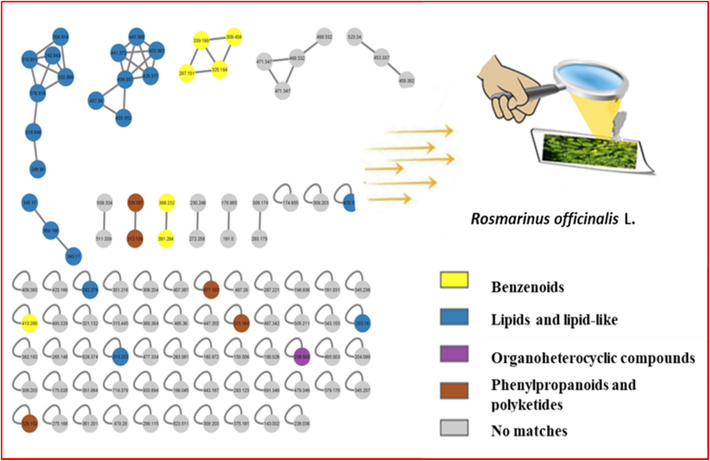
- Molecular networking (MNW) was prepared using MS/MS data from R. officinalis L. (rosemary) leaf extract. Nodes are labelled with a parent mass.
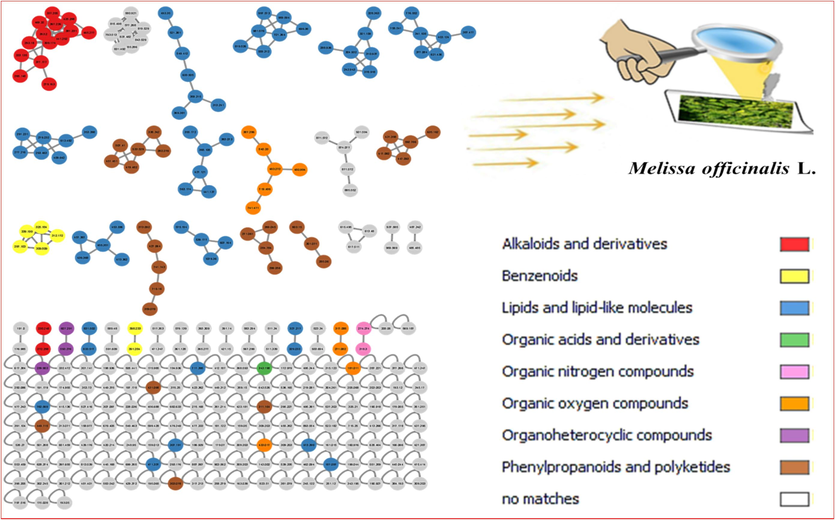
- Molecular networking (MNW) was prepared using MS/MS data from M. officinalis L. (lemon balm) leaf extract. Nodes are labelled with a parent mass.
Globally, molecular networking (MN) was used to explore visually the variance in the recorded metabolome of the investigated species and to make metabolite identification and categorization easier. The developed MNs were then analyzed using a MolNetEnhancer methodology, which improves the data annotation by merging results from various computational tools.
Rosemary MN was constructed of 101 nodes arranged as 12 clusters, each with a minimum of two connected nodes, and 61 self-nodes that are represented as a pie chart (Fig. 7). While, the 289 nodes in the created lemon balm MN were divided into 36 clusters, each with a minimum of two connected nodes, and 138 single nodes that are represented as a pie chart (Fig. 8).
4 Discussion
Mosquitoes are an age-old nuisance pest and mosquito-borne diseases signify an increasing global health challenge. C. pipiens (Diptera: Culicidae) is a commonly spread mosquito in Egypt, transmitting dreadful diseases leading to high morbidity and sometimes mortality in humans and animals (Abdel-Shafi et al., 2016). Mosquito combating is necessary to prevent the spread of many pathogens that can be transmitted through these vectors, which is beneficial to humans and the environment as it reduces the spread of diseases and pollution of the environment and food chain. Additionally, using natural products to control the spread of mosquitoes will be useful in preventing the transmission of food pathogens carried by the mosquitoes and also in decreasing the negative side effects of synthetic chemical insecticides as environmental and food contaminants (Onen et al., 2023). Furthermore, employing environmentally safe natural substances for disease and vector control ensures safety for the environment and helps combat mosquito resistance to chemical insecticides (Niroumand et al., 2016; Tantawy et al., 2020; Demarque and Espindola, 2021). Natural products such as medicinal plant extracts and essential oils exert high larvicidal activities against many mosquitoes including C. pipiens larvae (Chellappandian et al., 2018; Sultan, 2020; El-Akhal et al., 2021; Hillary et al., 2024). In this study, four Egyptian plants, rosemary, lemon balm, chicory, and chard have been chosen based on their traditional uses and applied as natural larvicidal agents against the larvae of C. pipiens.
Current findings indicate that the rosemary and lemon balm extracts had the highest effectiveness in inducing mortality in larvae of C. pipiens compared to the other tested extracts (chicory and chard), wherein they recorded the lowest LC50 and LC90. When compared together rosemary extract exhibited a higher effect than the lemon balm extract. In the same context, several studies performed to increase the effectiveness of plant extracts or their essential oils as biocontrol agents for controlling the spread of mosquitoes including C. pipiens as reported in the published reports (Shalaby and Khater, 2005; Koliopoulos et al., 2010; Chafik and Afaf, 2020; Zeghib et al., 2020; Pavela et al., 2021; Ramzi et al., 2022; Priya et al., 2023).
The current results are promising and applicable; herein the rosemary and lemon balm herbals are available and can thrive in various geographical areas. Likewise, the exposure of the rosemary and lemon balm extracts also declined the carbohydrates, lipid, and total protein contents in the C. pipiens under exposure to LC50 and LC90. These results are consistent with previous studies (Uritu et al., 2018; Ramzi et al., 2022). Carbohydrates constitute the main source of glucose essential for variable biological processes in the insect body (Hamama et al., 2022). The depletion in the carbohydrate content may be attributed to utilizing the larval tissues' reserved carbohydrate sources as a result of plant extract stress (Shehata, 2018). Exposure to rosemary and lemon balm extracts led to the reduction of lipid and protein content in C. pipiens, possibly indicating a shift in energy metabolism towards lipid catabolism due to the stress induced by the active components in the extracts.
Sharma and colleagues suggested that the reduction in total protein content may be attributed to hormones that regulate protein synthesis interacting with the active components present in the tested plant extracts (Sharma et al., 2009; Sharma et al., 2011). Furthermore, Senthilkumar and coauthors (Senthilkumar et al., 2009) reported that the protein level in Anopheles Stephensi larvae treated with some botanical extract was reduced and ascribed that to the disruption action of the plant extracts on the protein synthesis mechanism. The obtained results are harmonious with those stated before (Chafik and Afaf, 2020; Alghamdi 2021). Chafik and Afaf found that exposure to the essential oils of R. officinalis causes a decrease in numerous morphometric parameters such as the width of the thorax, weight, and body volume of the larval stage 4 individuals of C. pipiens compared to the control (Chafik and Afaf, 2020). Meanwhile, Alghamdi found that the extracts of natural products from Juniperus procera and Dodonaea viscosa plants cause some morphological deformations in the C. pipiens larvae during the larval development stage into a pupa and in the emergence stage from a pupa into a complete insect (Alghamdi, 2021).
In the current study, the biochemical response following the exposure to LC50 and LC90 of the different tested extracts was studied by detecting the AChE and GST activities. Tested extracts decreased significantly (P < 0.05) the AChE activity compared to its activity in the control group. AChE is a pivotal enzyme to regulate the terminal nerve impulse, it is responsible for the hydrolysis of the neurotransmitter acetylcholine in insects. AChE is a well-known biomarker of neurotoxicity (Rajagopalan et al., 2023). The inhibitory effect of tested plant extracts on AChE activity is detailed in several published reports using various insecticides against different insect species including C. pipiens (Zhou et al., 2016; Al-Solami, 2021; Ali et al., 2021; Riaz, 2023).
Furthermore, GSTs play an essential role in the biotransformation and detoxification of exogenous substances, and in protecting organisms against oxidative stress. The increased level of GST activity indicated that the targeted larvae detoxification system was stimulated by the tested extracts. Here, the meaningful rise of GST activity under the LC50 and LC90 of rosemary and lemon balm extracts on the GST activity is in parallel with those reported (Zeghib et al., 2020) using rosemary extract and its essential oil (Chaudhari et al., 2021); using median lethal concentrations (50 and 90) of M. officinalis against C. pipiens and other insects.
The potent effect of the rosemary and lemon balm extracts is attributed to their active components that can be toxic to the mosquito vector, C. pipiens. The increased activity of R. officinalis L. compared to M. officinalis L. leaves extract could be explained by its high content of polyphenols and terpenoids, especially those belonging to rosmarinic acid derivatives. These types of metabolites have been identified that might enhance the toxicity toward C. pipiens larvae and agree with those results published before (David et al., 2000; Kannathasan et al., 2011; Hadidy et al., 2022). To establish a scientific structural-activity relationship, we should consider the shared compounds between the two active extracts and the compounds exclusive to rosemary, which is the most active one.
The shared compounds between the active extracts could contribute significantly to their combined larvicidal activity. For instance, terpenoids, such as rosmanol, and micromeric acid are found in both extracts and belong to a class of compounds recognized for their bioactivity, including insecticidal potential. However, their potential role in larvicidal activity warrants investigation. Rosmanol is a diterpene, while micromeric acid is a triterpenoid. Both are a large and diverse class of naturally occurring organic metabolites derived from isoprene.
On the other hand, there are compounds exclusively identified in rosemary such as rosmanol methyl ether, rosmadial, rosmaridiphenol, epi-rosmanol methyl ether, and others. We suggest that the presence of metabolites corresponding to terpenoids with rosmarinic acid derivatives may increase larvicidal activity. It is possible that the larvicidal activity of these extracts is due to the presence of these and other bioactive compounds. However, further research would be needed to determine the exact mechanism of action and to perform a detailed structure–activity relationship analysis.
The potential mechanisms that may explain the toxicity of extracts from R. officinalis and M. officinalis on C. pipiens larvae could be due to several factors. The presence of phenolic and terpenoid compounds with antioxidant properties may damage the gut epithelial membrane in mosquito larvae (War et al., 2012, Raguvaran et al., 2023). Additionally, the extracts may reduce feeding activity (Marques et al., 2016), hinder insect digestive enzymes and processes (Czerniewicz et al., 2017; Yadav and Kant-Upadhyay 2022), and inhibit detoxification enzymes, including glutathione S-transferase (Wang et al., 2014). Additionally, the synergistic interaction between fatty acids and polyphenolic compounds is known to play a crucial role in insecticidal activity (Tak et al., 2017). In summary, although we can propose hypotheses regarding the larvicidal activity of these compounds based on their known properties, it is crucial to undertake a comprehensive scientific approach. This approach should involve the isolation of individual compounds, conducting structure–activity studies, and performing mechanistic investigations. Only through such a rigorous scientific process can we establish a robust structural-activity relationship and fully elucidate the potential of these natural products for effective mosquito control.
Limitations and future perspectives
Further studies could focus on investigating the potential synergistic effects of combining the most effective extracts in comparison with other natural or common synthetic larvicides to broaden their applicability in mosquito control programs. It will also be important to isolate and elucidate the structure of the major compounds that are accountable for the observed activities. Additionally, large-scale field trials would be necessary to assess the environmental impact of this larvicide and larvae resistance to it compared to traditional larvicides. If successful, this research could lead to the development of a safe, sustainable, and effective tool for controlling mosquito populations.
5 Conclusion
In this study, four Egyptian herbal extracts were evaluated as natural larvicides against the third instar larvae of the mosquito C. pipiens. Based on the obtained outcomes, the extracts of R. officinalis L. (rosemary) and M. officinalis L. (lemon balm) were more effective against the larvae of C. pipiens. Their exposure caused a high mortality rate and recorded LC50 values of 9.795 and 26.505 μg/mL, respectively. It disrupted the biochemical and physiological parameters (total carbohydrates, total lipids, total protein, AChE, and GST) when compared with C. intybus (Chicory) and B. vulgaris (Chard) leaves and steam, which recorded LC50 values of 150.867, 179.128, and 363.093 μg/mL, respectively. Moreover, the results of the UPLC/MS revealed that rosemary and lemon balm extracts involved numerous bioactive compounds, such as flavonoids, terpenoids, phenolic acids, and alkaloids. In general, rosemary and lemon balm extracts can be considered promising natural control agents against C. pipiens mosquitos, and these actions are attributed to their contents of bioactive constituents. Further studies are required for the development and evaluation of a safe larvicidal formula based on these plant- extracts.
CRediT authorship contribution statement
Mostafa A. Asmaey: Writing – review & editing, Writing – original draft, Visualization, Validation, Software, Project administration, Methodology, Investigation, Formal analysis, Data curation, Conceptualization. Hanan Y. Aati: Writing – review & editing, Project administration. Mahmoud Emam: Writing – review & editing, Writing – original draft, Visualization, Software, Investigation, Formal analysis, Data curation. Nikolas Tsafantakis: Writing – review & editing, Supervision, Resources, Investigation, Conceptualization. Nikolas Fokialakis: Writing – review & editing, Supervision, Resources, Investigation, Conceptualization. Mohamed seif: Writing – review & editing, Writing – original draft, Visualization, Software, Data curation. Ahmed I. Hasaballah: Writing – review & editing, Writing – original draft, Supervision, Resources, Project administration, Methodology, Investigation, Conceptualization.
Acknowledgements
The authors would like to thank the Researchers Supporting Project number (RSP2024R504), King Saud University, Riyadh, Saudi Arabia.
Declaration of competing interest
The authors declare that they have no known competing financial interests or personal relationships that could have appeared to influence the work reported in this paper.
References
- Monitoring of synthetic insecticides resistance and mechanisms among malaria vector mosquitoes in Iran: a systematic review. Heliyon 2022e08830
- [Google Scholar]
- Assessment of the chemical profile and potential biocontrol of Amphora coffeaeformis against foodborne pathogens and Culex pipiens L. to ensure food safety. J. Food Saf.. 2024;44:e13124
- [Google Scholar]
- Mosquito identification and molecular xenomonitoring of lymphatic filariasis in selected endemic areas in Giza and Qualioubiya Governorates, Egypt. J. Egypt. Soc. Parasitol.. 2016;46:93-100.
- [Google Scholar]
- Midguts of Culex pipiens L. (Diptera: Culicidae) as a potential source of raw milk contamination with pathogens. Sci. Rep.. 2022;12:13183
- [Google Scholar]
- Toxicological and biological effects of some plant extracts on the house mosquito Culex pipiens, the dominant species in Jeddah governorate. Entomol. Res.. 2021;51:403-412.
- [Google Scholar]
- Bioactivity of medicinal plant extracts as toxicants and enzyme inhibitors against insect pests of stored commodities. J. Crop Prot.. 2021;10:95-109.
- [Google Scholar]
- Larvicidal activity of plant extracts by inhibition of detoxification enzymes in Culex pipiens. J. King Saud Univ.-Sci.. 2021;33:101371
- [Google Scholar]
- Phytochemical analysis and assessment of antioxidant and antimicrobial activities of some medicinal plant species from Egyptian flora. J. Appl. Biomed.. 2018;16:289-300.
- [Google Scholar]
- Chemical constituents from Colchicum palaestinum (Baker) C. Archer with the assessment of its antioxidant, wound scratch, and tyrosinase repressive potential. S. Afr. J. Bot.. 2023;157:209-218.
- [Google Scholar]
- Unravelling the Secrets of α‐Pyrones from Aspergillus Fungi: A Comprehensive Review of Their Natural Sources, Biosynthesis, and Biological Activities. Chem. & Biodiv.. 2023;20(11):e202301185
- [Google Scholar]
- Natural peroxides from plants: historical discovery, biosynthesis, and biological activities. Chem. Biodiv. 2024e202400644
- [Google Scholar]
- Larvicidal activities of Allium sativum L. and Zingiber officinale Rosc. extracts against filariasis vectors in Hadiya Zone, Ethiopia. Biomed. Res. Int.. 2023;2023:6636837
- [CrossRef] [Google Scholar]
- Belhumeur, P.N., Chen, D., Feiner, S., et al., 2008. Searching the world’s herbaria: a system for visual identification of plant species. In: Computer Vision–ECCV 2008: 10th European Conference on Computer Vision, Marseille, France, October 12–18, 2008, Proceedings, Part IV 10. Springer.
- LC-MS analysis and antioxidant activity of the hydro-alcoholic extract of Melissa officinalis L. from Algeria. Chem. J. Moldova. 2020;15:78-87.
- [Google Scholar]
- Functional and structural diversity of insect glutathione S-transferases in xenobiotic adaptation. Int. J. Biol. Sci.. 2022;18:5713.
- [Google Scholar]
- Rosmarinus officinalis leaves as a natural source of bioactive compounds. Int. J. Mol. Sci.. 2014;15:20585-20606.
- [Google Scholar]
- A rapid and sensitive method for the quantitation of microgram quantities of protein utilizing the principle of protein-dye binding. Anal. Biochem.. 1976;72:248-254.
- [Google Scholar]
- Chafik, M., Afaf, B.J., 2020. The evaluation of the larvicidal activity of the essential oil extracted from Officinalis rosmarinus on morphometric parameters Culex pipiens. 10.
- Essential oils and their bioactive compounds as eco-friendly novel green pesticides for management of storage insect pests: prospects and retrospects. Environ. Sci. Pollut. Res.. 2021;28:18918-18940.
- [Google Scholar]
- Toxicological effects of Sphaeranthus indicus Linn. (Asteraceae) leaf essential oil against human disease vectors, Culex quinquefasciatus Say and Aedes aegypti Linn., and impacts on a beneficial mosquito predator. Environ. Sci. Pollut. Res. Int.. 2018;25:10294-10306.
- [Google Scholar]
- Modeling the distribution of the West Nile and Rift Valley Fever vector Culex pipiens in arid and semi-arid regions of the Middle East and North Africa. Parasites Vectors. 2014;7:289.
- [CrossRef] [Google Scholar]
- Essential oil composition and larvicidal activity of six Mediterranean aromatic plants against the mosquito Aedes albopictus (Diptera: Culicidae) Parasitol. Res.. 2010;107:1455-1461.
- [CrossRef] [Google Scholar]
- Changes in the amounts of carbohydrates, phosphagen, and related compounds during the metamorphosis of the blowfly, Lucilia cuprina. J. Insect Physiol.. 1967;13:1575-1592.
- [Google Scholar]
- Role of phenolic compounds during antioxidative responses of winter triticale to aphid and beetle attack. Plant Physiol. Biochem.. 2017;118:529-540.
- [CrossRef] [Google Scholar]
- Differential toxicity of leaf litter to dipteran larvae of mosquito developmental sites. J. Invertebr. Pathol.. 2000;75:9-18.
- [Google Scholar]
- Challenges, advances and opportunities in exploring natural products to control arboviral disease vectors. Front. Chem.. 2021;9:779049
- [Google Scholar]
- ClassyFire: automated chemical classification with a comprehensive, computable taxonomy. J. Cheminf.. 2016;8:1-20.
- [Google Scholar]
- Colorimetric method for determination of sugars and related substances. Anal. Chem.. 1956;28:350-356.
- [Google Scholar]
- Essential oils extracted from different species of the Lamiaceae plant family as prospective bioagents against several detrimental pests. Molecules. 2020;25:1556.
- [Google Scholar]
- Chemical composition and larvicidal activity of Lavandula angustifolia subsp. angustifolia and Lavandula dentata spp. dentata essential oils against Culex pipiens larvae, vector of West Nile virus. Psyche: J. Entomol.. 2021;2021:1-7.
- [Google Scholar]
- Exploring natural products-based cancer therapeutics derived from egyptian flora. J. Ethnopharmacol.. 2021;269:113626
- [CrossRef] [Google Scholar]
- Eco-friendly dyeing of cotton fabrics using a blend of Olea europaea L. and Ziziphus spina-christi L. Leaf extracts: chemical profiling and color fastness properties. Egypt. J. Chem.. 2024;67:207-213.
- [Google Scholar]
- Phytochemical profiling of Lavandula coronopifolia poir. Aerial parts extract and its larvicidal, antibacterial, and antibiofilm activity against Pseudomonas aeruginosa. Molecules. 2021;26:1710.
- [Google Scholar]
- Insect glutathione transferases and insecticide resistance. Insect Mol. Biol.. 2005;14:3-8.
- [CrossRef] [Google Scholar]
- MolNetEnhancer: enhanced molecular networks by integrating metabolome mining and annotation tools. Metabolites. 2019;9:144.
- [Google Scholar]
- Chemical composition of four essential oils and their adulticidal, repellence, and field oviposition deterrence activities against Culex pipiens L. (Diptera: Culicidae) Parasitol. Res.. 2024;123:110.
- [Google Scholar]
- Dengue fever as a reemerging disease in upper Egypt: diagnosis, vector surveillance and genetic diversity using RT-LAMP assay. PLoS One. 2022;17:e0265760
- [Google Scholar]
- Potential anti-Alzheimer effects of selected Lamiaceae plants through polypharmacology on glycogen synthase kinase-3β, β-secretase, and casein kinase 1δ. Ind. Crop Prod.. 2019;138:111431
- [Google Scholar]
- Origin and status of Culex pipiens mosquito ecotypes. Curr. Biol.. 2022;32:R237-R246.
- [CrossRef] [Google Scholar]
- Larvicidal and repellent potential of Ageratum houstonianum against Culex pipiens. Sci. Rep.. 2022;12:21410.
- [CrossRef] [Google Scholar]
- Virulence of entomopathogenic fungi against Culex pipiens: impact on biomolecules availability and life table parameters. Saudi J. Biol. Sci.. 2022;29:385-393.
- [Google Scholar]
- Efficacy of plant products in controlling disease vector mosquitoes, a review. Entomol. Exp. Appl.. 2024;172:195-214.
- [Google Scholar]
- Mosquito larvicidal activity of methyl-p-hydroxybenzoate isolated from the leaves of Vitex trifolia Linn. Acta Trop.. 2011;120:115-118.
- [Google Scholar]
- Parathion and methyl parathion resistance in diamondback moth (Lepidoptera: Plutellidae) larvae. J. Econ. Entomol.. 1989;82:1299-1304.
- [Google Scholar]
- Karunaratne, S., Surendran, S., 2022. Mosquito control: a review on the past, present and future strategies.
- Larvicidal efficacy of fifteen plant essential oils against Culex pipiens L. mosquitoes in Egypt. Egypt. J. Vet. Sci.. 2023;54:183-192.
- [Google Scholar]
- Chemical basis of the sulfo-phospho-vanillin reaction for estimating total serum lipids. Clin. Chem.. 1972;18:199-202.
- [Google Scholar]
- Chemical composition and larvicidal evaluation of Mentha, Salvia, and Melissa essential oils against the West Nile virus mosquito Culex pipiens. Parasitol. Res.. 2010;107:327-335.
- [Google Scholar]
- Chemical profiling of Kaliziri injection and quantification of six caffeoyl quinic acids in beagle plasma by LC-MS/MS. Pharmaceuticals. 2022;15:663.
- [Google Scholar]
- Malpighia emarginata DC. bagasse acetone extract: phenolic compounds and their effect on Spodoptera frugiperda (JE Smith)(Lepidoptera: Noctuidae) Chil. J. Agric. Res.. 2016;76:55-61.
- [Google Scholar]
- Enrichment of antioxidant compounds from lemon balm (Melissa officinalis) by pressurized liquid extraction and enzyme-assisted extraction. J. Chromatogr. A. 2013;1288:1-9.
- [Google Scholar]
- Isolation and identification of Emericella nidulans secondary metabolites. Chem. Res. J. 2018;3:114-119.
- [Google Scholar]
- Metabolomic profiling of streptomyces griseorubens with the evaluation of their antioxidant and anticancer potentialities. Egypt. J. Chem. 2024
- [Google Scholar]
- An evidence-based review on medicinal plants used as insecticide and insect repellent in traditional Iranian medicine. Iran. Red Crescent Med. J.. 2016;18
- [Google Scholar]
- Mosquito-borne diseases and their control strategies: an overview focused on green synthesized plant-based metallic nanoparticles. Insects. 2023;14:221.
- [Google Scholar]
- Inhibitory effects of oil extract of green Acalypha (Acalypha wilkesiana) on antioxidant and neurotransmitter enzymes in Callosobruchus maculatus. J. Basic Appl. Zool.. 2019;80:1-13.
- [Google Scholar]
- Encapsulation of Carlina acaulis essential oil and carlina oxide to develop long-lasting mosquito larvicides: microemulsions versus nanoemulsions. J. Pest. Sci.. 2021;94:899-915.
- [Google Scholar]
- Bioactive molecules derived from plants in managing dengue vector Aedes aegypti (Linn.) Molecules. 2023;28:2386.
- [Google Scholar]
- Bioefficacy of isolated compound l-isoleucine, N-allyloxycarbonyl-, and dodecyl ester from entomopathogenic actinobacteria Actinokineospora fastidiosa against agricultural insect pests, human vector mosquitoes, and antioxidant activities. Environ. Sci. Pollut. Res. Int.. 2023;30:42608-42628.
- [CrossRef] [Google Scholar]
- Acetylcholinesterase biosensors for electrochemical detection of neurotoxic pesticides and acetylcholine neurotransmitter: a literature review. Environ. Res. 2023115724
- [Google Scholar]
- Insecticidal effect of wild-grown Mentha pulegium and Rosmarinus officinalis essential oils and their main monoterpenes against Culex pipiens (Diptera: Culicidae) Plants. 2022;11:1193.
- [Google Scholar]
- An extensive review on the consequences of chemical pesticides on human health and environment. J. Clean. Prod.. 2021;283:124657
- [Google Scholar]
- Effect of different plants extract on acetylcholinesterase activity of Aedes aegypti and Musca domestica adults. Pak. J. Sci.. 2023;74:12-19.
- [Google Scholar]
- Mosquitoes (Culicidae). Guide to Entomological Surveillance during Contingency Operations. Washington, DC: Walter-Reed Army Institute of Research; 2001.
- Larvicidal and adulticidal activities of some medicinal plants against the malarial vector. Anopheles Stephensi (Liston). 2009;104:237-244.
- [Google Scholar]
- Activity of Otostegia fruticosa (Lamiaceae) leaves extracts against lymphatic filariasis vector, Culex pipiens L. (Diptera: Culicidae) Egypt. Acad. J. Biol. Sci. A, Entomol.. 2020;13:175-186.
- [Google Scholar]
- Toxicity of certain solvent extracts of Rosmarinus officinalis against Culex pipiens larvae. Environ. Sci.. 2005;69:80.
- [Google Scholar]
- Anti-juvenile activity of Azadirachta indica extract on the development and morphometry of filaria vector, Culex quinquefasciatus (Diptera: Culicidae) Say. Parasitol. Res.. 2009;105:1193-1203.
- [Google Scholar]
- Status of carbohydrate, protein and lipid profile in the mosquito larvae treated with certain phytoextracts. Asian Pac. J. Trop. Med.. 2011;4:301-304.
- [Google Scholar]
- Repellent activity of Deverra triradiata (Apiaceae) extracts against Anopheles sergentii Theobald, Culex pipiens Liston and Culex antennatus Becker mosquitoes. J. Egypt. Soc. Parasitol.. 2018;48:599-604.
- [Google Scholar]
- Biological activity of Prunus domestica (Rosaceae) and Rhamnus cathartica (Rhamnaceae) leaves extracts against the mosquito vector, Culex pipiens L. (Diptera: Culicidae) Egypt. Acad. J. Biol. Sci. F. Toxicol. Pest Control. 2019;11:65-73.
- [Google Scholar]
- Phytochemical composition and bioactive potential of Melissa officinalis L., Salvia officinalis L. and Mentha spicata L. extracts. Foods. 2023;12:947.
- [Google Scholar]
- A semimicrotechnique for the estimation of cholinesterase activity in boll weevils. Ann. Entomol. Soc. Am.. 1964;57:367-371.
- [Google Scholar]
- Larvicidal efficacy of some natural products against Culex pipiens larvae. Egypt. Vet. Med. Soc. Parasitol. J.. 2020;16:102-113.
- [Google Scholar]
- Effects of rosemary, thyme and lemongrass oils and their major constituents on detoxifying enzyme activity and insecticidal activity in Trichoplusia ni. Pesticide Biochem. Physiol.. 2017;140:9-16.
- [Google Scholar]
- The larvicidal activity of natural inspired piperine-based dienehydrazides against Culex pipiens. Bioorg. Chem.. 2020;94:103464
- [Google Scholar]
- Facile synthesis, larvicidal activity, biological effects, and molecular docking of sulfonamide-incorporating quaternary ammonium iodides as acetylcholinesterase inhibitors against Culex pipiens L. Bioorg. Chem.. 2022;128:106098
- [Google Scholar]
- Assessing the phytochemical profile and potential of traditional herbal infusions against aldose reductase through in silico studies and LC-MS/MS analysis. Appl. Sci.. 2022;12:8361.
- [Google Scholar]
- Medicinal plants of the family Lamiaceae in pain therapy: a review. Pain Res. Manag.. 2018;2018
- [Google Scholar]
- Application of UHPLC-ESI-QTOF-MS in phytochemical profiling of sage (Salvia officinalis) and rosemary (Rosmarinus officinalis) Planta Med. Int. Open. 2020;7:e133-e144.
- [Google Scholar]
- Simultaneous determination of caffeic acid and its major pharmacologically active metabolites in rat plasma by LC-MS/MS and its application in pharmacokinetic study. Biomed. Chromatogr.. 2015;29:552-559.
- [Google Scholar]
- Inhibition of insect glutathione S-transferase (GST) by conifer extracts. Arch. Insect Biochem. Physiol.. 2014;87:234-249.
- [CrossRef] [Google Scholar]
- Mechanisms of plant defense against insect herbivores. Plant Signal. Behav.. 2012;7:1306-1320.
- [CrossRef] [Google Scholar]
- Guidelines for Laboratory Field Testing of Mosquito Larvicides. Geneva: WHO; 2005.
- Therapeutic and insecticidal potential of plant terpenes: a review. Int. J. Green Pharm.. 2022;16:14.
- [Google Scholar]
- Chemical composition and larvicidal activity of Rosmarinus officinalis essential oil against west Nile vector mosquito Culex pipiens (L.) J. Essent. Oil Bear. Plants. 2020;23:1463-1474.
- [Google Scholar]
- Aphicidal activity of Illicium verum fruit extracts and their effects on the acetylcholinesterase and glutathione S-transferases activities in Myzus persicae (Hemiptera: Aphididae) J. Insect Sci.. 2016;16:11.
- [Google Scholar]
- Effect of the entomopathogenic fungus, Beauveria bassiana, and its secondary metabolite on detoxifying enzyme activities and acetylcholinesterase (AChE) of the Sunn pest, Eurygaster integriceps (Heteroptera: Scutellaridae) Biocont. Sci. Technol.. 2009;19:485-498.
- [Google Scholar]