Leaching of rubidium from biotite ore by chlorination roasting and ultrasonic enhancement
⁎Corresponding authors at: Faculty of Metallurgical and Energy Engineering, Kunming University of Science and Technology, Kunming 650093, Yunnan, China. glin08@126.com (Guo Lin), guolin@kust.edu.cn (Guo Lin), zhanglibopaper@126.com (Libo Zhang)
-
Received: ,
Accepted: ,
This article was originally published by Elsevier and was migrated to Scientific Scholar after the change of Publisher.
Peer review under responsibility of King Saud University.
Abstract
Aiming at the problems of the low grade of rubidium (Rb) in biotite, and long leaching time and low leaching efficiency of Rb in mica ore, the chlorination roasting-assisted ultrasonic enhanced water leaching method was employed to extract Rb from biotite ore in this study. During the chlorination roasting process, the optimal conditions were obtained, namely roasting temperature 900 °C, roasting time 40 min, and the mass ratio of ore to calcium chloride 1:1, the optimum leaching rate was 96.75 %. Compared with conventional leaching, the ultrasonic field could greatly shorten the leaching time and realize fast and efficient leaching of Rb. The optimal conditions for ultrasonic enhanced leaching were: ultrasonic power 100 W, leaching temperature 60 °C, leaching time 20 min, liquid–solid ratio 4:1, the Rb leaching rate was 98.73 %, which was 40 min shorter than conventional leaching. The particle size and SEM results indicated that the samples by ultrasonic leaching were smaller, no agglomeration phenomenon in a large area, and the surface of the samples was relatively smooth.
Keywords
Biotite
Rb
Chlorination roasting
Water leaching
Ultrasonic
1 Introduction
Rubidium (Rb) is widely used because of its unique physical and chemical properties. As a significant element, the demand for Rb far exceeds its output. Rb is extremely active and has no a complex purification process of Rb. Therefore, how to efficiently extract Rb from low-grade rubidium-containing minerals is widely concerned (Xing et al., 2018; Zhang et al., 2020). The process of extracting rubidium salt from rubidium-containing ore including acid method (such as hydrochloric acid and sulfuric acid) and alkali method (Zhang et al., 2019). Due to the complicated process and high cost, the hydrochloric acid method was replaced by the sulfuric acid method (Chen et al., 2018). However, aluminum in the minerals will react with sulfuric acid to cause a passivation reaction, resulting in the consumption of large amount of sulfuric acid. In extraction process, the alkali is needed to neutralize the excess acid. In addition, the aluminum salt reacts with excess alkali to form aluminum hydroxide precipitate (Zhang et al., 2021). The disadvantage of the sulfuric acid method is that the aluminum hydroxide produced will adsorb a part of Rb, making it difficult to purify and remove impurities. Moreover, the treatment process needs to be added due to the introduction of sulfuric acid. The alkaline process mainly includes the chlorination roasting method and the limestone sintering method. The chlorinated roasting-water leaching method is currently widely used in the extraction of Rb from low-grade biotite ore due to its shorter process, lower energy consumption, excellent comprehensive utilization effect and higher metal recovery rate (Xing et al., 2021; Vieceli et al., 2016). Zheng et al. extracted rubidium from distinctive kaolin ore with chlorinated water leaching, and the extraction rate was 96.95 % at Rb grade 0.22 % (Zheng et al., 2016). Tian et al. used a chlorination roasting-water immersion method to extract rubidium from muscovite (Tian et al., 2020), and the results indicated that the extraction rate reached the highest (96.71 %) at Rb grade 0.24 % under roasting temperature of 800 °C and water leaching time of 30 min.
However, the above experiments were all carried out at relatively high Rb grades, and there are still some problems with the water leaching method such as long leaching time and low leaching efficiency (Jiang et al., 2018). In order to shorten the leaching time and improve the leaching efficiency, new approaches are desperately needed. Ultrasonic technology is a high-tech discipline that has emerged in recent years. The cavitational, mechanical, and thermal effects generated when ultrasonic waves are applied to the solution will stimulate the instantaneous high temperature and pressure to strengthen the hydrometallurgical reaction process, especially the leaching and mineral extraction process (Rahim Pouran et al., 2016; He et al., 2015). Zhang et al. found that the leaching rate of Ge reached 92.7 % under the optimal ultrasonic conditions for leaching Ge from germanium-containing slag (Zhang et al., 2016). However, it required 100 min to achieve the optimal Ge leaching rate by conventional leaching, with a leaching rate of 88.35 %. Xin et al. investigated the process of leaching zinc oxide concentrate with sulfuric acid enhanced by ultrasound-H2O2, the results indicated that the leaching rate by ultrasonic was 8.76 % higher than that of mechanical stirring (Xin et al., 2021). Zhang et al. investigated the process of leaching Rb from iron sulfate by conventional roasting, and found that the leaching rate of rubidium was only 87.3 % under conventional water immersion for 60 min (Zhang et al., 2022). To improve the leaching rate of Rb in biotite ore, this study proposed to strengthen the leaching of Rb from biotite ore by ultrasonic to improve the low leaching rate and long leaching time of traditional leaching method.
In the paper, Rb in biotite ore was leached by calcium chloride roasting-ultrasonic enhanced in aqueous solution. The effects of roasting temperature, holding time and mass ratio of ore to calcium chloride on Rb leaching rate were studied. The leaching time, temperature, liquid–solid ratio and ultrasonic power on Rb leaching rate were also investigated. The leaching residues were characterized by XRD, SEM and particle size to analyze the mechanism of ultrasonic enhanced leaching of Rb. This research provided a useful reference for the efficient extraction of Rb from biotite ore.
2 Experimental
2.1 Materials and analysis
The biotite ore in this study was provided by a company in Guangdong and its chemical composition analysis was presented in Table 1. The calcium chloride dihydrate (CaCl2·2H2O) was of analytical grade and produced from Shanghai Maclens Biochemical Technology Co., Ltd.
SiO2 | Al2O3 | K2O | TFe | MnO | MgO | PbO | Li2O | Na2O | Rb2O | Others |
---|---|---|---|---|---|---|---|---|---|---|
65.89 | 14.33 | 2.79 | 5.60 | 0.14 | 0.57 | 0.67 | 0.06 | 1.90 | 0.08 | 7.97 |
Before the experiment, the biotite and calcium chloride dihydrate were crushed to a particle size of < 150 µm, and the X-ray diffraction (XRD) pattern and particle size were shown in Fig. 1. SEM analysis of the raw ore and Elemental distribution of the raw ore was shown in Fig. 2. It could be found from Table 1, Fig. 1 and Fig. 2 that the biotite ore contained Si, Al, K, Fe, Mg, Pb, Na and other elements. It could also be observed that Rb was complex mineral composed of other elements. The raw material was primarily composed of SiO2, Rb2PbO2, Al2SiO5, and Rb2MgSi5O12. In addition, Fig. 1 also showed that the surface morphology of the sample was rough and evenly distributed. EDS results indicated that most of the samples are not only oxides of calcium, silicon and aluminum, but also contain some iron and calcium. And trace amounts of Rb might not be observed.
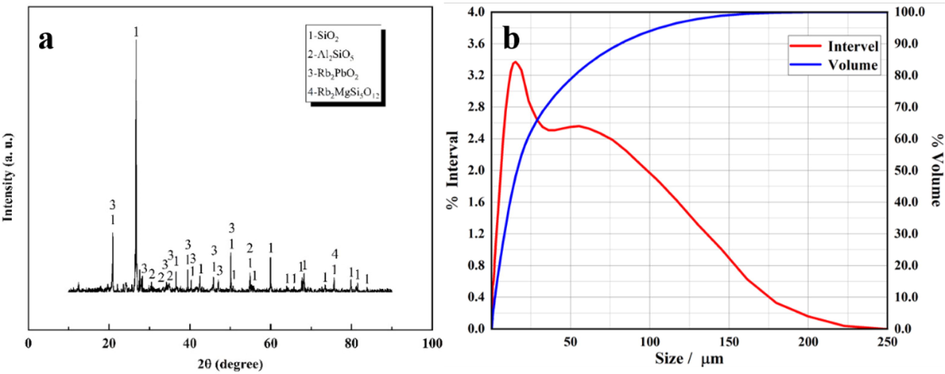
- (a) XRD pattern, (b) particle size analysis of the raw ore.
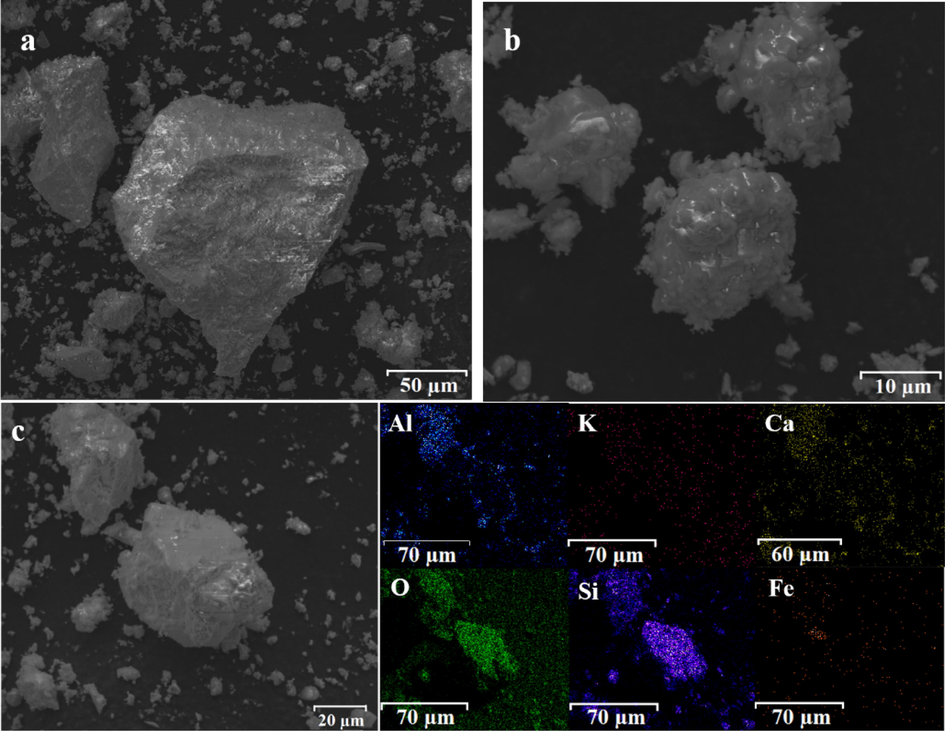
- (a, b) SEM and elemental (c) distribution of the raw ore.
The main equipment included high-speed pulverizer (GJ1001 Hebi Metallurgy Mechanical Device Co., Ltd), muffle furnace (SX2410 Wuhan Electric Furnace Co., Ltd), laser particle size distribution instrument (BT-9300HT Dandong Better Instrument Co., Ltd), low-temperature ultrasonic extractor (heating power 1000 W and the maximal ultrasonic power 1500 W) (VOSHIN-1500C Wuxi Voshin Instruments Co., Ltd), heat-collecting magnetic stirrer (heating power 600 W and stirring power 25 W) (DF-101S Shanghai Jin State apparatus Technology Co., Ltd), vacuum filtration device (DZF-6050 Gongyi Yuhua Instrument Co., Ltd), and drying oven (SHZ-D3 Gongyi Yuhua Instrument Co., Ltd), etc.
2.2 Experimental method
2.2.1 Chlorination roasting
After mixing biotite and calcium chloride, the chlorination roasting experiment was carried out in a muffle furnace to investigate the effects of roasting temperature, roasting time, and mass ratio of biotite ore to calcium chloride on the leaching rate of Rb. The effect of roasting factors on the leaching rate of Rb was investigated under stirring speed 200 rmp, leaching temperature 60 °C, leaching time 120 min, and liquid–solid ratio 4:1. The possible reactions of biotite ore with calcium chloride at high temperature were as follows (Shan et al., 2013):
2.2.2 Leaching experiment
The optimal roasting product was used as the leaching material. The conventional leaching and ultrasonic enhanced leaching experiments were carried out in a heat-collecting magnetic stirrer and a low-temperature ultrasonic extractor, respectively. The effects of leaching time, ultrasonic power, leaching temperature, and liquid-to-solid ratio on Rb leaching rate were investigated.
Rb in the biotite ore was converted to soluble rubidium chloride (RbCl), which was then transferred into solution by water leaching. The residue was collected, filtered, and analyzed the content of Rb to calculate the leaching rate by Eqs. (7).
3 Results and discussion
3.1 Chlorination roasting
3.1.1 Effect of calcination temperature
The effect of calcination temperature on the Rb leaching rate was examined under roasting time of 25 min and the mass ratio of biotite ore to calcium chloride of 1:1. The roasting product was crushed and used as raw material for leaching. The relationship between the leaching rate of Rb and the calcination temperature was shown in Fig. 3. Due to the melting point of CaCl2 was 773 °C (Zhang et al., 2020), in order to ensure the occurrence of chlorination reaction, the initial temperature was set at 700 °C. The results showed that with the increase of temperature, the leaching rate of Rb increased firstly and then decreased. The optimal leaching rate was 70.89 % at 900 °C. Before 900 °C, the reason for the lower leaching rate was mainly due to insufficient reaction of calcium chloride and ore. As the temperature increased, more and more rubidium-containing phases were occurred. When the temperature reached 950 °C, RbCl was wrapped by a large amount of calcium aluminosilicate, which was not conducive the leaching of Rb and resulted in a decrease of leaching rate (Wang et al., 2020). Moreover, the volatilization of RbCl at high temperature was also an important reason for the decrease of Rb leaching rate.
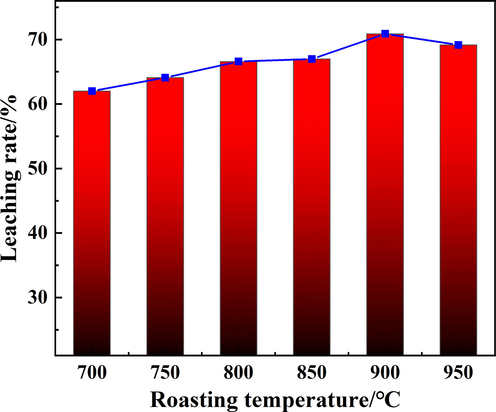
- The effect of roasting temperature on the Rb leaching rate.
The XRD pattern of rubidium ore roasted at different temperatures were shown in Fig. 4. It can be found that RbClO3 was generated during roasting process, which indicated calcium chloride reacted with rubidium compound in the mineral. RbClO3 was an intermediate product, and the peak intensity of RbClO3 gradually decreased with increasing temperature. At 900 °C, the peak intensity of RbClO3 almost disappeared. At the same time, the peak of RbCl increased, which indicated that part of RbClO3 was reduced to RbCl. When the temperature continued to rise, rubidium compound reacted with other substances. Meanwhile, the peak of calcium chloride also weakened with the increase of temperature, suggested that the calcium chloride reacted. Moreover, calcium chloride combined with silica and lead silicate to generate CaPb8Si6O21. In a word, observing the XRD at different temperature, it can be found that more rubidium at 900 °C existed in the form of chloride.
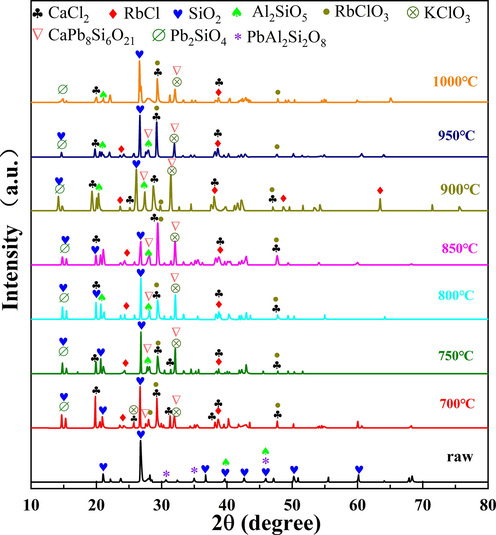
- XRD pattern of rubidium ore roasted at different temperatures.
3.1.2 Effect of the roasting time
The relationship between roasting time and leaching rate of Rb was investigated at roasting temperature of 900 °C, and a mass ratio of biotite ore to calcium chloride of 1:1. The results were shown in Fig. 5. At 40 min, the leaching rate of Rb was close to the maximum. Before 40 min, the leaching rate of Rb increased rapidly due to the roasting time was too short, the reaction of the chlorination process was insufficient, and part of Rb in the form of Rb-containing phase still existed in the biotite. After 40 min, the chlorination reaction of Rb-containing phase tended to be stable, and the leaching process of Rb gradually slowed down. Based on the practical applications, the longer the roasting time, the higher the energy consumption and cost, while the leaching rate not increased significantly. Therefore, further experiments were carried out at roasting time of 40 min.
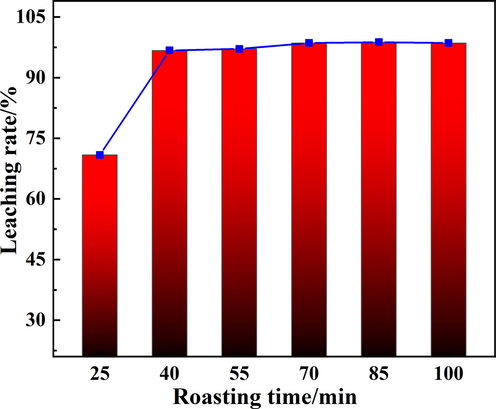
- The effect of roasting time on the Rb leaching rate.
3.1.3 Effect of mass ratio of biotite to calcium chloride
The effect of mass ratio of biotite to calcium chloride on Rb leaching rate was carried out at roasting temperature of 900 °C, roasting time of 40 min and the results were presented in Fig. 6. When the ratio was 0.5:1, the leaching rate reached 86.98 %. It was due to the formation of calcium silicate from excess calcium chloride during roasting process, resulting in an incomplete leaching process. With the increase of the ratio of biotite ore to calcium chloride, the leaching rate of Rb reached the maximum value under 1:1. As the amount of ore increases, the Rb-containing phase in biotite ore could not be transformed into RbCl completely, which resulted in a decrease of leaching rate. Therefore, the optimal roasting conditions were roasting temperature 900 °C, roasting time 40 min, and mass ratio of biotite ore to calcium chloride 1:1. Under these conditions, the leaching rate of Rb in biotite ore reached 96.75 %.
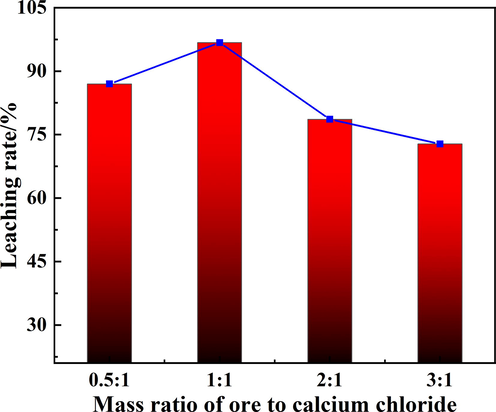
- The effect of mass ratio of ore to calcium chloride on the Rb leaching rate.
3.2 Ultrasonic enhanced leaching
3.2.1 Effect of ultrasonic power
The relationship between ultrasonic power and Rb leaching rate was investigated under leaching temperature 60 °C, leaching time 20 min, and liquid–solid ratio 4:1. The result was shown in Fig. 7. During the roasting process, the main components of raw materials and additives were calcium chloride, calcium aluminosilicate, silicates and a small amount of rubidium salt. Water was a polar molecular structure with a V-shaped structure and adjacent water molecules were associated by hydrogen bonds (Kim et al., 2021). As the temperature increased, in addition to enhancement in thermal motion, the hydrogen bonds associated with adjacent water molecules in the aqueous solution were partially dissociated. Based on the polar characteristics, water was a good solvent. Chloride compounds had an ionic bond structure. In an aqueous solution, the negatively charged part of the polar water molecule surrounds the metal ion, while the positively charged part surrounds the chloride ion, thereby causing the metal chloride ion to leave the crystal lattice and enter the solution. The soluble rubidium chloride was obtained by leaching and filtering roasted biotite ore.
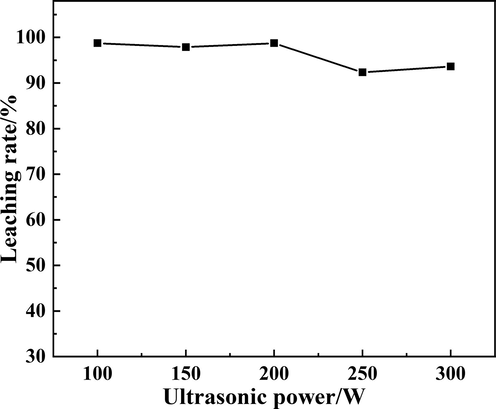
- The effect of ultrasonic power on the Rb leaching rate.
It could be seen in Fig. 7 that the Rb leaching rate reached 98.73 % under ultrasonic power 100 W, which indicated that ultrasound improved the leaching efficiency significantly. It may due to that the mechanical and cavitation effects of ultrasonic, which can generate instantaneous high temperature, high pressure and powerful jets to accelerate mass and heat transfer and promote the degree of the reaction (Zhou et al., 2021). However, with the increase of ultrasonic power, the Rb leaching rate was constant or even tended to decrease, which showed that the leaching efficiency would be weakened at higher ultrasonic power. This might be due to the coalescence of bubbles generated by cavitation under high ultrasound power (Bhangu and Ashokkumar, 2016), or at higher ultrasonic power, the cycle and decompression times were shorten so that the liquid molecules did not separate to form voids (Zou et al., 2020; Li et al., 2018). Hence, ultrasonic cavitation could not occur. In general, the inclusions were opened, and part of the RbCl wrapped by a large amount of calcium aluminosilicate were better leached under the ultrasonic, which improved the leaching efficiency of Rb.
And then, by calculation, the energy consumption of conventional leaching is 2250 kJ, while the energy consumption of ultrasonic leaching is only 1800 kJ, and the energy consumption of 20 % can be saved by ultrasonic leaching. Compared the energy consumption of ultrasound and conventional, it can be reflected the advantages of ultrasound.
3.2.2 Effect of leaching time
The effect of leaching time on Rb leaching rate via conventional water leaching and ultrasonic treatment was carried out and presented in Fig. 8. The conventional leaching was performed with stirring speed 200 rpm, leaching temperature 60 °C, liquid-to-solid ratio 4:1, leaching time 30–180 min, and the ultrasonic enhanced leaching was conducted under ultrasonic power 100 W, leaching temperature 60 °C, liquid-to-solid ratio 4:1, leaching time 10–60 min.
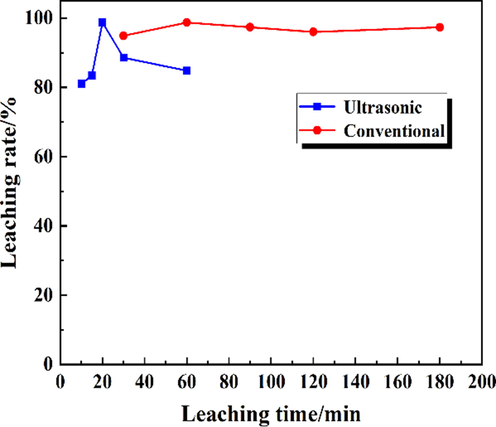
- The influence of leaching time on the Rb leaching rate.
During the conventional leaching process, the leaching rate of Rb increased firstly and then remained stable. Subsequent fluctuations were within a controllable range. The leaching rate reached the maximum value of 98.7 % at 60 min. Under the ultrasonic-enhanced leaching, the change trend of leaching rate was increase first and then decrease. This may be because the particles become very fine and agglomeration occurs under long-term ultrasound, which reduces the leaching rate (Hansen et al., 2022). The Rb leaching rate reached the highest value of 98.73 % at 20 min, indicating that the leaching time was greatly shortened under the ultrasonic.
3.2.3 Effect of leaching temperature
Temperature was also an important parameter in the leaching process, and usually the leaching process could be promoted as the temperature increased. To compared conventional leaching and ultrasonic-enhanced leaching, the effect of temperatures on the Rb leaching rate was investigated and the results were shown in Fig. 9. During the conventional leaching, the stirring speed was 200 rpm, the leaching time was 60 min, the liquid-to-solid ratio was 4:1, and the leaching temperature was set to 30–70 °C. In the ultrasonic enhanced leaching, the ultrasonic power was 100 W, the leaching time was 20 min and other factors was the same as the conventional.
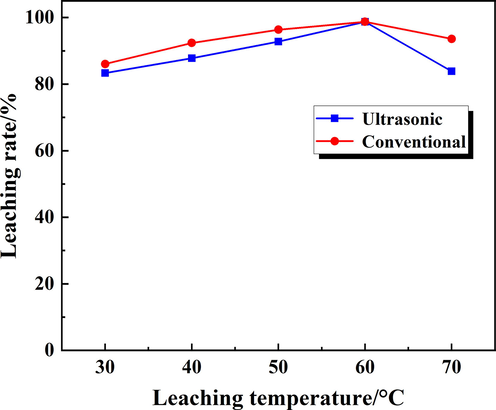
- The effect of leaching temperature on the Rb leaching rate.
During conventional leaching process, the leaching rate of Rb increased slowly with the increase of temperature. At 60 °C, the leaching rate of Rb reached the highest value of 98.7 %. When the temperature was below 60 °C, as the temperature increased, the diffusion coefficient and rate constant were increased, which was conducive to the progress of the leaching reaction. In addition, the leaching process need to undergo five processes: external diffusion, internal diffusion, analysis process, soluble matter diffusion through solid membrane and soluble product diffusion into solution (Faraji et al., 2020; Daryabor et al., 2017). As the leaching temperature increased, the diffusion process intensified, and when it reached a certain temperature, the diffusion process tended to balance. In a certain range, the diffusion process would be restricted to making the influence of temperature on the leaching rate more complicated. During the ultrasonic enhanced leaching, the leaching rate increased linearly with the temperature between 30 °C and 60 °C. While the leaching temperature was 60 °C, the leaching rate reached 98.73 %, which attributed to the mechanical and cavitation effects of ultrasonic and further promoted the diffusion process of the leaching reaction, therefore, the leaching rate could reach the maximum value in a short time.
3.2.4 Effect of liquid-to-solid ratio
The liquid-to-solid ratio was another important factor in the leaching process. A low liquid-to-solid ratio was difficult to complete the reaction, while a high liquid-to-solid ratio will cause more solution consumption and cumbersome treatment processes. The effects of conventional leaching and ultrasonic-enhanced leaching on Rb leaching rates at different liquid–solid ratios were compared. In conventional leaching, the stirring speed was 200 rpm, the leaching time was 60 min, the leaching temperature was 60 °C, the liquid-to-solid ratio was set to 2:1, 3:1, 4:1, 5:1, respectively. In ultrasonic leaching, the ultrasonic power was 100 W, the leaching time was 20 min and other factors were same as the conventional. The results were presented in Fig. 10.
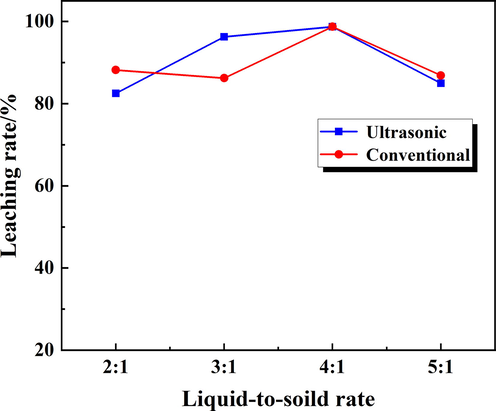
- Effect of liquid to solid ratio on the Rb leaching rate.
The Rb leaching rate was relatively low under a lower liquid–solid ratio, which was mainly because the liquid–solid mixture was viscous and resulted in an insufficient stirring and adverse to the mass transfer process of the leaching reaction. As the liquid-to-solid ratio increased, the calcine reacted fully with the solution, the leaching rate of Rb also increased and reached the maximum at liquid-to-solid of 4:1 ratio. During ultrasonic enhanced leaching, although the Rb leaching rate was lower under liquid-to-solid ratio 2:1, while the strengthening effect of the ultrasonic field was reflected when the liquid-to-solid ratio was increased to 3:1, which indicated that in a short leaching time, ultrasonic-enhanced leaching could achieve the same Rb leaching rate as conventional leaching (Cui et al., 2019).
3.3 Characterization of the filter residue
SEM and particle size distribution of the samples included before and after leaching (optimal leaching conditions) were examined and presented in Fig. 11. As shown in Fig. 11(a), (b), and (c), the microstructure of the particles changed obviously, and the obvious agglomeration phenomenon almost disappeared after conventional and ultrasonic leaching, which indicated that the dense structure was broken under optimal leaching conditions. Moreover, compared with conventional leaching, the surface of the particles obtained by ultrasonic leaching was smoother. Fig. 11(d) showed the particle size distribution before and after leaching. After conventional leaching, the particle size decreased from 19.71 to 18.08. This was because agitation during conventional leaching could facilitate the dispersion of aggregated particles. After ultrasonic leaching, the average diameter was found to be further reduced from 19.71 to 18.33. A possible explanation was that the generation of many shock waves caused by cavitation broke up aggregated particles into smaller particles, which was more conducive to the improvement of the leaching rate of roasted ore.
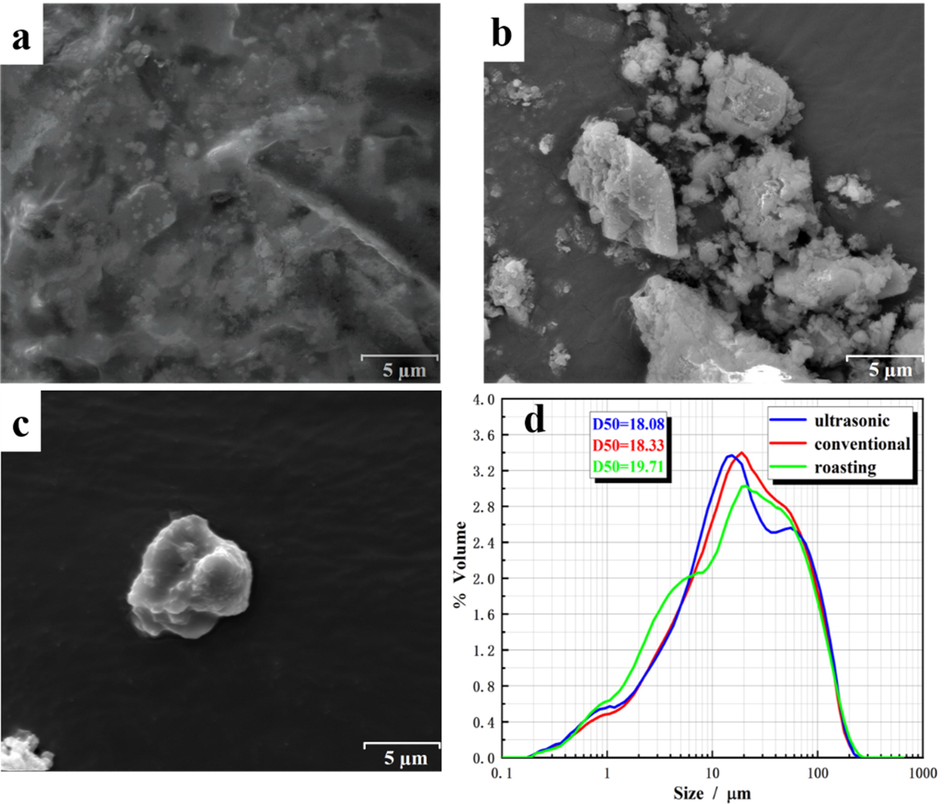
- SEM analysis: (a) optimal roasting product, (b) after conventional leaching (c) after ultrasonic-assisted leaching and (d) particle size distribution.
4 Conclusion
An improved process including the chlorination roasting and ultrasonic enhanced leaching to extract rubidium from biotite ore was proposed. The optimal calcination conditions were roasting temperature 900 °C, roasting time 40 min, and the mass ratio of biotite to calcium chloride 1:1. The optimal conventional leaching conditions were leaching temperature 60 °C, leaching time 60 min, liquid–solid ratio 4:1, and the leaching rate of Rb reached 98.7 % The optimal ultrasonic enhanced leaching conditions were liquid–solid ratio 4:1, ultrasonic power 100 W, leaching temperature 60 °C, leaching time 20 min, and the leaching rate of Rb was 98.73 %. Compared with conventional leaching, the leaching rate of Rb was slightly improved by ultrasonic method. The introduction of ultrasonic greatly shortened the leaching time from 60 min (conventional leaching) to 20 min (ultrasonic enhanced leaching). The energy consumption of the conventional was 2250 kJ, and the ultrasonic one was 1800 kJ. The chlorination roasting-ultrasonic enhanced water leaching provides a feasible method for industrial extraction of Rb.
Acknowledgements
This work was supported by the Hubei Provincial Department of Education Science and Technology Research Program Young Talent Project (Q20201102).
Declaration of Competing Interest
The authors declare that they have no known competing financial interests or personal relationships that could have appeared to influence the work reported in this paper.
References
- A cleaning process for the removal and stabilisation of arsenic from arsenic-rich lead anode slime. J. Cleaner Prod.. 2018;176:26-35.
- [Google Scholar]
- The Role of Oxalic Acid in the Leaching System for Recovering Indium from Waste Liquid Crystal Display Panels. ACS Sustainable Chem. Eng.. 2019;7(4):3849-3857.
- [Google Scholar]
- Solvent extraction of cadmium and zinc from sulphate solutions: Comparison of mechanical agitation and ultrasonic irradiation. Ultrason Sonochem.. Jan 2017;34:931-937.
- [Google Scholar]
- Frequency controlled agglomeration of pt-nanoparticles in sonochemical synthesis. Ultrason. Sonochem.. Apr 2022;85:105991.
- [Google Scholar]
- Recovery of cathode materials and Al from spent lithium-ion batteries by ultrasonic cleaning. Waste Manag.. Dec 2015;46:523-528.
- [Google Scholar]
- Ultrasound-assisted leaching of cobalt and lithium from spent lithium-ion batteries. Ultrason. Sonochem.. Nov 2018;48:88-95.
- [Google Scholar]
- Sulfuric Acid Baking—Water Leaching for Gold Enrichment and Arsenic Removal from Gold Concentrate. Minerals.. 2021;11(12)
- [Google Scholar]
- Ultrasound augmented leaching of nickel sulfate in sulfuric acid and hydrogen peroxide media. Ultrason. Sonochem.. Jan 2018;40(Pt A):1021-1030.
- [Google Scholar]
- Ultrasound and UV assisted Fenton treatment of recalcitrant wastewaters using transition metal-substituted-magnetite nanoparticles. J. Mol. Liq.. 2016;222:1076-1084.
- [Google Scholar]
- Modified calcination conditions of rare alkali metal Rb-containing muscovite (KAl2[AlSi3O10](OH)2) Rare Met.. 2013;32(6):632-635.
- [Google Scholar]
- Non-isothermal kinetic studies of rubidium extraction from muscovite using a chlorination roasting-water leaching process. Powder Technol.. 2020;373:362-368.
- [Google Scholar]
- Optimization of Lithium Extraction from Lepidolite by Roasting Using Sodium and Calcium Sulfates. Miner. Process. Extr. Metall. Rev.. 2016;38(1):62-72.
- [Google Scholar]
- Extraction of lithium, rubidium and cesium from lithium porcelain stone. Hydrometallurgy. 2020;191
- [Google Scholar]
- Recovery of Zn and Ge from zinc oxide dust by ultrasonic-H2O2 enhanced oxidation leaching. RSC Adv.. 2021;11(53):33788-33797.
- [Google Scholar]
- Clean and efficient process for the extraction of rubidium from granitic rubidium ore. J. Cleaner Prod.. 2018;196:64-73.
- [Google Scholar]
- Rubidium extraction from mineral and brine resources: A review. Hydrometallurgy 2021:203.
- [Google Scholar]
- Energy-efficient and simultaneous extraction of lithium, rubidium and cesium from lepidolite concentrate via sulfuric acid baking and water leaching. Hydrometallurgy. 2019;185:244-249.
- [Google Scholar]
- Efficient co-extraction of lithium, rubidium, cesium and potassium from lepidolite by process intensification of chlorination roasting. Chem. Eng. Process. - Process Intensificat.. 2020;147:107777
- [Google Scholar]
- Separation and recovery of cesium sulfate from the leach solution obtained in the sulfuric acid baking process of lepidolite concentrate. Hydrometallurgy. 2021;199
- [Google Scholar]
- Simultaneous extraction of lithium, rubidium, cesium and potassium from lepidolite via roasting with iron(II) sulfate followed by water leaching. Hydrometallurgy. 2022;208
- [Google Scholar]
- Comparison of ultrasonic-assisted and regular leaching of germanium from by-product of zinc metallurgy. Ultrason. Sonochem.. Jul 2016;31:143-149.
- [Google Scholar]
- A chlorination roasting process to extract rubidium from distinctive kaolin ore with alternative chlorinating reagent. Int. J. Miner. Process.. 2016;157:21-27.
- [Google Scholar]
- Recycling of LiCoO2 cathode material from spent lithium ion batteries by ultrasonic enhanced leaching and one-step regeneration. J. Environ. Manage.. 2021;277
- [Google Scholar]
- Extraction of Indium from By-products of Zinc Metallurgy by Ultrasonic Waves. Arabian J. Sci. Eng.. 2020;45(9):7321-7328.
- [Google Scholar]