Translate this page into:
Modified cotton fabrics with poly (3-(furan-2-carboamido) propionic acid) and poly (3-(furan-2-carboamido) propionic acid)/gelatin hydrogel for UV protection, antibacterial and electrical properties
⁎Corresponding author. ghadasci@yahoo.com (Ghada M. Taha)
-
Received: ,
Accepted: ,
This article was originally published by Elsevier and was migrated to Scientific Scholar after the change of Publisher.
Peer review under responsibility of King Saud University.
Abstract
This research aimed to prepare smart cotton fabrics with multi functions for antibacterial activity, UV protection and electrical conductivity via in situ coating with conductive polymer and conductive hydrogel. Therefore, 3-(furan-2-carboamido) propionic acid was synthesized followed by polymerization using ceric ammonium nitrate. In addition, cotton fabrics coated with 3-(furan-2-carboamido) propionic acid via in situ polymerization and by the hydrogel that based on poly (3-(furan-2-carboamido) propionic acid) and gelatin which have been performed via in situ gelation process. The chemical structure and morphology of the 3-(furan-2-carboamido) propionic acid (monomer) and the synthesized polymer (PFu) were investigated by H1NMR, IR, SEM, TGA and DSC. Where, the treated fabrics (PFu-T and PFu-G-T) are characterized by SEM, FTIR and contact angle. Furthermore, the AC electrical conductivity and dielectric properties of PFu, PFu-T, PFu-G-T and blank were investigated over the frequency range of 20 Hz–10 MHz at room temperature using impedance spectroscopy where the electric conductivity values are 1.74 × 10-5, 7.5 × 10-8, 4 × 10-7, 8.24 × 10-11 (S·cm)-1, respectively. In addition, the anti-bacterial activity of PFu-T, PFu-G-T and blank was assessed versus gram-positive and gram-negative bacteria where, PFu-G-T shows activity against Escherichia coli and Staphylococcus aureus. Moreover, PFu-T, PFu-G-T showed high UV protection especially for PFu-G-T.
Keywords
Smart fabrics
Poly (3-(furan-2-carboamido) propionic acid)
Gelatin
Hydrogel
Electric conductivity and anti-bacterial
1 Introduction
Nowadays many researches are interested to fabricate smart cotton fabrics with multi-functions, while the antibacterial and electrical conductive fabrics have attracted attention to their versatility, especially in health care. Smart fabrics can be defined as fabrics that are manufactured and modified to sense and transfer different external or environmental which can be chemical, mechanical, magnetic, thermal, electrical, optical, etc. (Tao, 2001; Cherenack and van Pieterson, 2012). Cotton fabrics are the most popular fabrics all over the world due to their significant features such as softness, comfortable, flexibility, easy to care, affinity to skin, hygroscopic and regeneration property (Lu et al., 2007; Saravanan et al., 2009; Zhang et al., 2013), although their ability to absorb large amount of humidity which is considered a good media to grow microorganisms which lead to undesirable effects, both on the human health and on the cotton fabrics properties such as mechanical strength, unpleasant odor, stains and discoloration.
Amongst antibacterial agents applied for developing fabrics are chitosan (El-Bisi et al., 2016; Xu et al., 2018; Xu et al., 2019), β-Cyclodextrin (Selvam et al., 2012; Wang and Cai, 2008), N-halamines (Liu et al., 2015; Pan et al., 2018), quaternary ammonium compounds (Elena and Miri, 2018; Liu et al., 2014; Ma et al., 2018; Simoncic and Tomsic, 2010) and metals/metal oxides (Hebeish et al., 2014; Hassabo et al., 2019; Gao et al., 2019).
For the last few years, research and development towards wearable electronic fabrics with multi functions as antibacterial and hydrophobicity such as health monitoring, protection, safety, and healthy lifestyle gained strong interest (Lymberis and Paradiso, 2008). Conductive fabrics can be manufactured by different material such as conductive polymers such as polypyrrole (Lv et al., 2019), polyaniline (Nassar et al., 2013) and polyfuran (González-Tejera et al., 2008), metal nanoparticles (Park et al., 2012), and carbons (Kowalczyk et al., 2019; Ko et al., 2018).
Conducting Polymers based on heterocycle compounds have great attention due to their good properties such as mechanical, good electrical, electrochemical, and optical properties. Conducting polymers can be classified into intrinsically conducting polymers (ICPs) and extrinsically conducting polymers (ECPs). Where the most used in electrical conductive textile is intrinsically conducting polymers (ICPs) which essentially contain a conjugated π-electron backbone responsible for electrical charge (Dai, 2004). Conductive polymers can be provided as solid or liquid dispersions or solutions. Where, ICPs have been deposited via chemical and electrochemical oxidation on the fabric surface (Kim et al., 2003). Furthermore, conductive polymers have a great advantage which can be seen in different applications by incorporating enzymes, antibodies and other biological moieties (Kotwal and Schmidt, 2001; Rivers et al., 2002; Kim et al., 2007). Polyfuran has less interest than polythiophene, polypyrrole, and polyaniline, etc., due to its high oxidation potential (González-Tejera et al., 2008). Many scientists overcome this problem by changing the reaction conditions with various composites and copolymers based on polyfuran (González-Tejera et al., 2008).
Furthermore, the cotton treated with hydrogel is used in different applications such as biomedical application (Štular et al., 2017; Pinho and Soares, 2018). Hydrogels are 3D cross-linked hydrophilic polymer with high porosity and high water content without dissolution reverse to their cross-linked which may be chemical or physical crosslink (Zheng and Wang, 2015). Hydrogels can be classified into two kinds; natural such as polysaccharides-based and polypeptides (proteins)-based, and synthetic such as PVA, PEG. Gelatin is a polypeptide (proteins)-based derived from collagen which is the most candidate for preparing hydrogels used in biomedical applications due to its large number of functional groups and is easily cross-linked (Jaipan et al., 2017). Electrically conductive hydrogels are fabricated with adding different conducting materials such as conductive polymers, metal nanoparticles, or carbon-based materials.
Eventually, in order to manufacture cotton fabrics with anti-bacterial property, UV protection and electrical conductivity; cotton fabrics were grafted with new bioactive and electrical conductive polymer that based on 3-(furan-2-carboamido) propionic acid. Moreover, the swelling and comfort properties of the cotton fabrics have been increased by the treatment of cotton fabrics with hydrogel based on gelatin and poly (3-(furan-2-carboamido) propionic acid) that was synthesized via in situ gelation process. The chemical structure of the synthesized polymer was confirmed with H1NMR analysis. The electrical conductivity of the grafted fabrics was investigated and the anti-bacterial activity of them was evaluated against Gram-positive bacteria (Staphylococcus aureus) and Gram-negative bacteria (Escherichia coli). Notably, the synthesized polymer is not synthesized or applied before.
2 Material and method
2.1 Materials
furan-2-carbohydrazide, succinic anhydride (Merck), toluene (Modern Lab chemicals, Egypt), ceric ammonium nitrate solution (Merck), ethanol (Modern Lab chemicals, Egypt), gelatin (sigma), sodium dodecyl benzene sulfonate (SDBS))Merck(, Di-methylol ethylene urea)Sigma), Di-aldhyde Glutaric acid)Sigma), All of the chemical reagents used in the experiments were analytical grade and used as raw materials without further purification. Water used is distilled.
2.2 Methods
2.2.1 Synthesis of 3-(furan-2-carboamido) propionic acid
3-(furan-2-carboamido) propionic acid was prepared as reported in literature (Kassem et al., 2019). In brief, a solution of furan-2-carbohydrazide (1.29 g, 0.01 mol) and succinic anhydride (1.02 g, 10 mmol) in toluene (20 mL) was stirred at room temperature for 24 hrs. The solvent was evaporated under reduced pressure at 50 °C to give a residue, which was titrated with diethyl ether (25 mL) to afford a solid which was filtered, dried and crystallized from ethanol to afford 3-(furan-2-carboamido) propionic acid as a yellowish solid.
Yield: 80%; mp 148–149 °C; IR (KBr) cm−1, ν: 3321 (OH), 3228 (NH), 3154 (CH), 1729 carboxylic (C = O), 1686 amide (C = O); 1H NMR (DMSO‑d6) δ/ppm: 3.04 (t, 2H, J = 6.2 Hz, CH2), 3.15 (t, 2H, J = 6.2 Hz, CH2), 6.70 (m, 1H, furan H-4), 7.58 (d, 1H, J = 7.4 Hz, furan H-3), 8.12 (d, 1H, J = 7.6 Hz, furan H-5), 9.24 (br. s, 1H, NH exchangeable), 9.50 (br. s, 1H, NH exchangeable), 11.84 (s, 1H, OH). MS m/z: 226 (M+, 12%). Anal. calcd. for C9H10N2O5 (226.19): C, 47.79; H, 4.46; N, 12.39; Found: C, 48.0; H, 4.48; N, 12.50.
2.2.2 Poly 3-(furan-2-carboamido) propionic acid synthesis
A solution of specific amount of ceric ammonium nitrate solution (CAN) (0.1 M) and SDBS (0.005 g) were stirred for 5 min. After that, 3-(furan-2-carboamido) propionic acid (0.3 g) dissolved in ethanol (20 mL) was dropped wisely to the emulsifier solution with continuous stirring for 20 min. The reaction vessel was kept stirring for 24 h at 80 °C for complete polymerization. The mixture was poured into ethanol (500 mL) and stirred for extended two hrs where polymer was collected on Buchner funnel with filter paper and washed for several times with ethanol before drying at 60 °C for 24 h.
2.2.3 Treatment of cotton fabrics with poly (3-(furan-2-carboamido) propionic acid)
Cotton fabrics were treated with poly (3-(furan-2-carboamido) propionic acid) via in situ chemical oxidation polymerization using ceric ammonium nitrate. Where, emulsion solution of sodium dodecyl benzenesulfonate SDBS (0.005 g) were stirred in distilled water (15 mL) for 5 min. A solution of (0.3 g) 3-(furan-2-carboamido) propionic acid dissolved in ethanol (20 mL) was then drop wisely added to the emulsifier solution with continuous stirring for 20 min. After that, five samples of cotton fabrics were immersed in solutions with continuous shaking in water bath at 80 °C for 15 min. Finally, a solution of specific amount of ceric ammonium nitrate solution (CAN) (0.1 M) added drop wisely to the reaction vessel. The reaction vessels were kept stirring around 2hrs for complete polymerization, after that Samples were removed, dried, cured and kept for further characterization.
2.2.4 Treatment of cotton fabrics with poly(3-(furan-2-carboamido) propionic acid)/gelatin hydrogel
Functionalization of cotton fabrics with hydrogel have been performed via in situ gelation process. A homogenous polymeric solution of 1gm gelatin and 0.1 g of 3-(furan-2-carboamido) propionic acid was prepared and a solution of specific amount of ceric ammonium nitrate solution (CAN) (0.1 M) added drop wise to the solution with shaking for 30 min. The solutions were then transferred to Petri-dishes and three pre-weighted textile subtracts were added to the solutions. After that 0.5 mL of di-methylol ethylene urea (textile crosslinker) was added to the solutions. Finally, the cross linking agent Di-aldhyde Glutaric acid was added to the solution under shaking for 5 min.
2.3 Characterization
The reaction progress was checked by pre-coated TLC Silica gel 0.2 nm F254 nm [Fluka], visualized under UV lamp 254 & 366 nm. IR spectra were performed on a JASCO FT-IR-6100 Fourier transform infrared spectrophotometer using the KBr pellet disk method for transmittance measurements. The H1NMR and C13NMR spectra were measured on Bruker Avance II 400 MHz spectrometer using deuterated Di-methyl sulfoxide DMSO‑d6 as the solvent. The antibacterial activities of treated fabrics were quantitatively evaluated against both gram-positive bacteria (Staphylococcus aureus (ATCC 25923)) and gram-negative bacteria (Escherichia coli (ATCC 35218)). Where, a suspension of each bacterial strain was freshly prepared by inoculating fresh stock culture from the tested reference strain into broth tube containing 7 mL of Muller Hinton Broth. The inoculated tubes were incubated aerobically at 37 °C for 24 h. Serial dilutions were carried out for each strain and dilution matching with 0.5 Mc-Farland scale standard was selected for the screening of antimicrobial activities. Antimicrobial susceptibility of tissues was determined using diffusion method. A volume of 100 μL of cell culture suspension matching with 0.5 Mc-Farland of target strains was spread onto the plates. To investigate the antibacterial activity of target tissues, they were added into individual plates. They were left for 1 h at 25 °C to allow a period of pre-incubation diffusion. The plates were re-incubated aerobically at 37 °C for 24 h to allow bacterial growth. After incubation, plates were observed and the zones of inhibition were measured to evaluate the antimicrobial activity for each of the treated cotton fabrics. The experiment was carried out in triplicates for statistical relevance and the Mean ± SE of results was calculated.
The ultraviolet protection factors (UPF) of untreated and treated fabrics were measured by the AATCC 183–2010 using UV–vis spectrophotometer. The control reference was measured as air. UPF was calculated from the transmission spectra of the fabrics in the scope of 290–400 nm (Hassabo et al., 2019) using the following equation:
Water uptake percentage of hydrogels was determined according to the difference between the weight of swollen samples (Ws) and the weight before swelling (Wd). Where, pre-weighed samples were immersed in 5 mL H2O for 24 hrs.
The percentage of water uptake was calculated using Equation:
Impedance spectroscopy was carried out using the Gw LRC meter-8110G interfaced to a computer with frequency ranging from 20 Hz to10 MHz at the room temperature, 303 K. Samples were mounted on the conductivity sample holder with stainless steel electrodes under spring pressure.
Complex impedance data, Z* can be represented by its real, Z' and imaginary, Z“ parts by the relation:
The equations for the dielectric constant, ε', the dielectric loss, ε′′, the real electrical M' and the imaginary electrical modulus M“ can be shown as:
where εo is the permittivity of the free space, A is electrode contact area and t is thickness of the sample, R is the resistance, C is the capacitance and , f being the frequency in Hz.
3 Results and discussion
3.1 Synthesis and polymerization of poly (3-(furan-2-carboamido) propionic acid), and the treated cotton fabrics
The general synthetic routes of 3-(furan-2-carboamido) propionic acid and its related polymer are outlined on Scheme 1. Furan homopolymer can be synthesized through free radical system, anionic system, cationic system, ɤ-Ray Irradiation and Stereospecific Systems (Gandini, 1977). Diaz’s mechanism is believed to be the most applicable mechanism for polyfuran where electron-transfer step occurs to form a radical cation with several resonance forms then followed by alternating chemical and electron-transfer reactions (González-Tejera, de la Blanca et al., 2008). On the other hand, the oxidative decarboxylation of carboxylic acid by ceric ammonium nitrate has been reported by A. Sezal Sarac and his team (Sheldon and Kochi, 1968). The H1NMR spectrum confirmed that the polymerization occurs via C6 and carboxylic group. Where, Fig. 1 show H1NMR of poly(3-(furan-2-carboamido) propionic acid), H1NMR analysis (DMSO‑d6) δ/ppm: 2.51(2H, CH2, C10), 3.15 (2H, CH2, C16), 2.42(, 2H, CH2, C15), 1.36(2H, CH2, C11),6.9 (1H, furan H-4), 7.56 (1H, J = 7.4 Hz, furan H-3), 7.56 (1H, J = 7.6 Hz, furan H-5), 0.86 (br. s, 1H, NH exchangeable, H9,17), 7.89 (br. s, 1H, NH exchangeable, H8,18), 10.18 (1H, OH). Fig. 2a shows FTIR of 3-(furan-2-carboamido) propanoic acid.Polymerization reaction mechanism of 4-(2-(Furan-2-carbonyl)hydrazinyl)-4-oxobutanoic acid.
HNMR of poly 3-(furan-2-carboamido) propionic acid.
FTIR of 3-(furan-2-carboamido) propionic acid, treated cotton fabrics and untreated cotton fabrics.
Herein, cotton fabrics were treated in situ with poly (3-(furan-2-carboamido) propanoic acid). Where, the expected mechanism between cotton fabrics and the synthesized compound would occur via free radical polymerization based on the fact of CeIV salts such as CeIV ammonium nitrate considerably importance gaining in the grafting of cellulose. Where, the free radicals obtained from cellulose oxidizing capable of initiating polymerization on cellulose through a single electron transfer (Hebeish and Guthrie, 2012). According to the previous studies, the expected mechanism of grafted cellulosic cotton fabrics with poly (3-(furan-2-carboamido) propanoic acid) and its hydrogel based gelatin outlined on Schemes 2 and 3. Where, Fig. 2a, b show FTIR of the treated cotton fabrics with poly (3-(furan-2-carboamido) propanoic acid) and the treated cotton fabrics with its related hydrogel respectively. FTIR chart of the treated cotton fabrics with poly(3-(furan-2-carboamido) propanoic acid) shows bands at 3330 related to (OH), 3269 related to (NH), 2882 corresponding to —CH stretching of cotton, band at 1704 related to carboxylic (C⚌O) and band at 1638 related to amide (C⚌O) appear. While, Fig. 2b shows bands at 3288 corresponding to —OH overlaps with stretching vibration of —NH. Another band at 2945 cm−1 results from the stretching vibration of aliphatic —CH, one intense band at 1532 cm−1 was assigned to vibration of-NH amide bending and another band at 1632 cm-1corresponds to —C⚌O stretching vibration. In addition, weak band at 2018 related to aromatic C—H bending.Mechanism of cotton fabrics treatment with PFu.
Mechanisum of cotton fabrics treatment with PFu/Gelatin hydrogel.
3.2 SEM of treated fabrics with poly 3-(furan-2-carboamido) propionic acid
Fig. 3(a, b, c) shows SEM images of (a) control cotton fabrics, (b) cotton treated with poly 3-(furan-2-carboamido) propionic acid, (c) cotton fabrics treated with the gelatin hydrogel/ poly 3-(furan-2-carboamido) propionic acid, at magnifications 1000, 4000 and 8000. Where, Fig. 3a shows smooth surface of untreated fabrics, 3b reflects a smooth rough thin layer of poly 3-(furan-2-carboamido) propionic acid on the surface of cotton fabrics, while 3c shows thick layer of gel on the surface which makes fibers closed to each other.SEM images of (a) control cotton fabrics, (b) cotton treated with poly 3-(furan-2-carboamido) propionic acid, (c) cotton fabrics treated with the gelatin hydrogel/ poly 3-(furan-2-carboamido) propionic acid.
3.3 Swelling value
Water uptake value or swelling value of the synthesized hydrogel and treated cotton fabrics with hydrogel is shown in Table 1. It is clearly obvious that swelling values in presence of PFu comparing with gelatin hydrogel decrease and this can be back to its lipophilic properties. Moreover, swelling ratio of polymers/gelatin hydrogels is larger than that of treated cotton/hydrogel and this is may be back to the degree of crosslinking.
Sample type
Swelling value
Gelatin hydrogel
162
Gelatin/ furan derivative hydrogel
142
Cotton / gelatin hydrogel
150.2
Cotton/ gelatin / furan hydrogel
130
3.4 Evaluation of ultra-violet protection factor (UPF)
Heterocyclic compounds have been reported to own a significant UV absorption property (Czajkowski et al., 2006; Tragoonwichian et al., 2008; Ocal et al., 2016). Table 2 shows Ultra-Violet Protection Factor (UPF) of untreated cotton fabrics, cotton treated with gelatin hydrogel, cotton treated with furan derivative, and cotton treated with furan derivative gelatin hydrogel. It is obvious that treated cotton fabrics have excellent UV protection comparing with that untreated according to standard methods, where the evolution of UPF value between 0 and 50 while between 15 and 24 is good, 25–39 is very good, and 40- greater than 50 is excellent UV protection (Ocal et al., 2016). And this means preventing the transmission of UVA and UVB through cotton fabrics.
Samples
UPF value
Blank
3.6
PFu-T
35
G-T
226
PFu-G-T
245
3.5 Contact angle measurements
Fig. 4 shows the contact angle of cotton fabrics treated with gelatin hydrogel and cotton fabrics treated with poly (3-(furan-2-carboamido) propionic acid)/gelatin hydrogel. Where, the obtained data agree the swelling value and this can be back to the lipophilicity of PFu.Contact angle of (a) cotton fabrics treated with gelatin hydrogel and (b) cotton fabrics treated with furan derivative gelatin hydrogel.
3.6 Evaluation of the anti-bacterial activities of treated cotton fabrics
The Antibacterial properties of cotton treated with poly 3-(furan-2-carboamido) propionic acid, gelatin hydrogel and poly 3-(furan-2-carboamido) propionic/gelatin hydrogel are measured against Escherichia coli (E. coli) as gram-negative bacteria and Staphylococcus aureus (S. aureus) as Gram-positive bacteria. Table 3 shows the antibacterial properties of poly 3-(furan-2-carboamido) propionic acid/cotton (PFu-T), poly 3-(furan-2-carboamido) propionic /gelatin hydrogel/cotton (PFu-G-T), gelatin hydrogel/cotton (G-T). Where, PFu-T has moderate activity against Escherichia coli comparing to the reference drug tetracycline. On the other hand, the treated cotton with PFu-G-T showed high activity against Escherichia coli and Staphylococcus aureus compare to the reference drug and this may be back to chemical structure of hydrogel and the crosslinker agent that increase the functional groups and give anti-bacterial properties.
Bacteria
Samples
Grampositive(S. aureus)
Gramnegative(E. coli)
G-T
(20 mm)
(20 mm)
PFu-G-T
(40 mm)
(35 mm)
PFu-T
Growth
(25 mm)
Tetracycline
(25 mm)
(30 mm)
3.7 Electrical conductivity measurements
Polyheterocycles are known to possess a major advantage of prolonged π-electron conjugation in their backbone, which enhance their electroactive properties, where conduction process occurs via π-electron delocalization along the polymer chain (Bredas and Street, 1985; Nalwa, 1989).
Further, the previous studies had been reported that the electrical conductivity value of PFu homopolymer depending on the doping type where it ranging from 10-6 to 80 Scm−1, while its value in undopping state is (10-11 S cm−1) (González-Tejera et al., 2008; Nalwa, 1989; Tourillon and Garnier, 1982). Moreover, the electric conductivity of polyfuran composite (Gök et al., 2005; Gök et al., 2004) and polyfuran co-polymers (Li et al., 2004) have been reported.
Herein, the electric conductivity value of the synthesized derivative of undopping polyfuran homopolymer is 1.74 × 10-5 S·cm−1, where the derivative effect on increasing the conductivity. And this can be explained in Scheme 4 where conjugation occurs via furan ring and the tail which lead to increase the charge mobilization. On the other hand, the electro-negativity of oxygen is bigger than that of carbon in the carbonyl group and this work to form negative and positive charge on the oxygen and carbon atoms respectively. Moreover, the lone pair of nitrogen atom enters the game and this let the positive charge to mobilize from carbon to nitrogen atom.Conjugation mechanism of 3-(furan-2-carboamido) propionic.
The electric conductivity of PFu coated cotton fabrics and its related hydrogel coated cotton fabrics have been studied, where the electric conductive values of PFu-T, PFu-G-T are 7.5 × 10-8, 4 × 10-7 S·cm−1, respectively comparing to that of blank cotton fabric which its value is 8.24 × 10-11 S·cm−1. The electronic exchange nature was studied via plotted log σ(ω) versus log f curves at room temperature for PFu, PFu-T, PFu-G-T and Blank samples, as shown in Fig. 5. For all the samples, it is observed that σ(ω) increases with increase in frequency. In general, the behavior of electrical conductivity with frequency in solids follows Jonsher's power law, given as:
-
During the polymerization process, rigid and hydrophobic chains of conducting polymers tend to twist and wrap in water and then form separate colonies of conducting polymers which decrease electrochemical efficiency (Ding et al., 2018; Gan et al., 2018; Hu et al., 2019). The use of di-methylol ethylene urea in PFu-- acts as a textile crosslinker as well as a mediator to control the growth of PFu and improve the diffusion.
-
Usually, the conduction at low frequency is due to the transport charge carrier but at high frequency is due to the localize charge carrier. This means the adding of gel in the structure may be increase the bonding lengths. Therefore, the mobility of charge carriers, consequently increase of conductivity at low frequency and decrease polarization strength at high frequency.
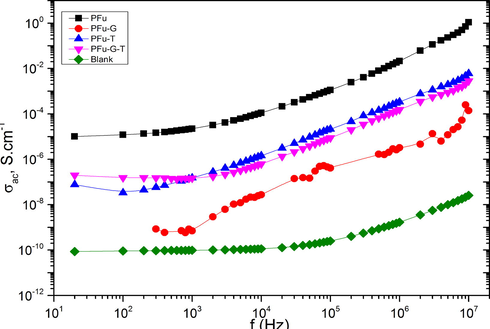
- Frequency dependence of the electrical conductivity measured at room temperature of PFu, Fu-T, Fu-G-T and blank.
Fig. 6 presents the frequency dependence of the real part of impedance Z' and the imaginary part of impedance Z'' using a double logarithmic scale for PFu, PFu-T, and PFu-G-T. From Fig. 8, the Z' and Z“ decrease slowly depending on the samples type, and continuously with an increase in frequency. The curves of Z” measured higher 5 kHz are a straight line with a negative slope of −1 in the double logarithmic representation. This fact indicates that imaginary part, Z″, is in inverse proportion to the frequency. In this case, the conduction species are frozen-in, so the dc conduction can be neglected and the ac conduction is dominant in high frequency. This is to say, the textile actually acts as an ideal capacitor with Fu-Gelatin being ideal insulator at higher frequency. This amazing result implies that PFu can sustain the intrinsic dielectric properties at high frequency.Double logarithmic plot of frequency dependence of real (Z') and imaginary (Z''') parts impedance.
Fig. 7 shows the -z''-z' plots, which leads to a distortion of the semicircles. The bulk resistances were calculated from the intersection of real impedance part at low frequency, in addition the bulk resistance for textile at room temperature is rapidly decreased when was treated by polyfuran.Nyquist impedance plots from PFu, PFu-T, PFu-G-T and blank at room temperature and the bulk resistance.
The conductivity behavior of polymer and polymer textiles can be understood from dielectric studies (Ramesh et al., 2002). The dielectric constant, ε', values indicate of stored charge. Its variation and that of dielectric loss, ε'', with frequency at room temperatures for samples PFu, PFu-T and PFu-T-G are shown in Fig. 8a, b respectively. There are no cognizable relaxation peaks observed in the frequency range employed in this study. Both dielectric constant and dielectric loss increase sharply at low frequencies indicating that electrode polarization and space charge effects have occurred corroborative non-Debye dependence (Qian et al., 2001; Govindaraj et al., 1995). On the other hand, at high frequencies, alternate of the electric field occurs so fast that there is no excess ion diffusion in the direction of the field. Polarization due to charge accumulation at electrode decreases, leading to the observed decrease in both dielectric constant and loss (Ramesh et al., 2002).Plot of dielectric constant (ε’) (a) and dielectric loss (b) Vs. frequency.
The dielectric constant and dielectric loss decreases due to the lower charge carrier density when textile treated by Fu-polymer. When gelatin was added to Fu-G-Textiles, the degree of Fu dispersed of in textiles increases resulting in the decrease in number of charge carrier density. A further analysis of the dielectric behavior would be more successfully achieved using electric moduli, which suppresses the effects of electrode polarization (Ramesh and Arof, 2001; Shastry and Rao, 1991).
The dependence of Z“ and M” with the frequency measured at room temperature for Fu-polymer, Fu-T and Fu-G-T samples are represented in Fig. 9. Both electric parameters are altogether considered in order to separate the localized (short-range conduction associated with the dielectric relaxation) and the non-localized (long-range associated with the free charge carrier) conductions. Moreover, the separation of Z“ and M” peaks shows a localized relaxation due to the short range conductivity in the polymer and textiles, which treated by polymer samples. [PFu, PFu-T and PFu-T-G are 65, 7 and 4 KHz separation between Z“ and M” peaks, respectively]The dependence of Z“ and M” with the frequency measured at room temperature for Fu-polymer, Fu-T and Fu-G-T.
4 Conclusion
This research aimed to prepare smart cotton surface with multi functions such as antibacterial activity, UV protection and good conductivity by coating with bioactive/conductive poly (3-(furan-2-carboamido) propionic) via in situ oxidation polymerization and its related bioactive/conductive hydrogel (poly 3-(furan-2-carboamido) propionic/gelatin) hydrogel via in situ gelation process. The ac electric conductivity of (PFu, PFu-T, PFu-G-T) and blank was studied at room temperature where the electric conductivity values are 1.74 × 10-5, 7.5 × 10-8, 4 × 10-7, 8.24 × 10-11 (S·cm−1) respectively. Moreover, treated cotton fabric showed good antibacterial as well as excellent UV protection giving it an added feature in biological applications especially those functionalized with hydrogel. In conclusion, the new derivative of polyfuran (poly (3-(furan-2-carboamido) propionic)) can be considered to be a new generation of bioactive / conductive polymers application.
Acknowledgements
The authors are thankful to National Research Centre (NRC), Egypt, financial support under project file No. AR110105, 2018, scientific center of excellence and Textile research centre of excellence for facilities provided.
Declaration of Competing Interest
The authors declare the following financial interests/personal relationships which may be considered as potential competing interests: National Research Centre (NRC), Egypt, financial support under project file No. AR110105, 2018.
References
- Polarons, bipolarons, and solitons in conducting polymers. Acc. Chem. Res.. 1985;18(10):309-315.
- [Google Scholar]
- Synthesis of reactive UV absorbers, derivatives of monochlorotriazine, for improvement in protecting properties of cellulose fabrics. Dyes Pigm.. 2006;71(3):224-230.
- [Google Scholar]
- Conducting polymers. In: Dai L., ed. Intelligent Macromolecules for Smart Devices: From Materials Synthesis to Device Applications. London: Springer London; 2004. p. :41-80.
- [Google Scholar]
- Nanocellulose-mediated electroconductive self-healing hydrogels with high strength, plasticity, viscoelasticity, stretchability, and biocompatibility toward multifunctional applications. ACS Appl. Mater. Interfaces. 2018;10(33):27987-28002.
- [Google Scholar]
- Super hydrophobic cotton fabrics via green techniques. Der Pharma Chemica. 2016;8(19):57-69.
- [Google Scholar]
- Formation of contact active antimicrobial surfaces by covalent grafting of quaternary ammonium compounds. Colloids Surf., B. 2018;169:195-205.
- [Google Scholar]
- Conductive and tough hydrogels based on biopolymer molecular templates for controlling in situ formation of polypyrrole nanorods. ACS Appl. Mater. Interfaces. 2018;10(42):36218-36228.
- [Google Scholar]
- The behaviour of furan derivatives in polymerization reactions. In: Polym. Chem.. Springer; 1977. p. :47-96.
- [Google Scholar]
- Construction of durable antibacterial and anti-mildew cotton fabric based on P(DMDAAC-AGE)/Ag/ZnO composites. Carbohydr. Polym.. 2019;204:161-169.
- [Google Scholar]
- Preparation and characterization of polyfuran/poly(2-fluoroaniline) conducting composites. J. Polym. Sci., Part B: Polym. Phys.. 2004;42(18):3359-3367.
- [Google Scholar]
- Polymers, composites, and characterization of conducting polyfuran and poly(2-bromoaniline) J. Appl. Polym. Sci.. 2005;98(5):2048-2057.
- [Google Scholar]
- Polyfuran conducting polymers: Synthesis, properties, and applications. Synth. Met.. 2008;158(5):165-189.
- [Google Scholar]
- Preparation, conductivity, complex permittivity and electric modulus in AgI⋅ Ag2O⋅ SeO3⋅ MoO3 glasses. Solid State Ionics. 1995;76(1–2):47-55.
- [Google Scholar]
- Development of multifunctional modified cotton fabric with tri-component nanoparticles of silver, copper and zinc oxide. Carbohydr. Polym.. 2019;210:144-156.
- [Google Scholar]
- The chemistry and technology of cellulosic copolymers. Vol vol. 4. Springer Science & Business Media; 2012.
- Development of improved nanosilver-based antibacterial textiles via synthesis of versatile chemically modified cotton fabrics. Carbohydr. Polym.. 2014;113:455-462.
- [Google Scholar]
- Elastomeric conductive hybrid hydrogels with continuous conductive networks. J. Mater. Chem. B. 2019;7(15):2389-2397.
- [Google Scholar]
- Gelatin-based hydrogels for biomedical applications. MRS Commun.. 2017;7(3):416-426.
- [Google Scholar]
- Synthesis and anticancer activity of new ((Furan-2-yl)-1,3,4-thiadiazolyl)-1,3,4-oxadiazole acyclic sugar derivatives. Chem. Pharm. Bull.. 2019;67:888-895.
- [Google Scholar]
- Characteristics of electrically conducting polymer-coated textiles. Mol. Cryst. Liq. Cryst.. 2003;405(1):161-169.
- [Google Scholar]
- Effect of immobilized nerve growth factor on conductive polymers: electrical properties and cellular response. Adv. Funct. Mater.. 2007;17(1):79-86.
- [Google Scholar]
- High durability conductive textile using MWCNT for motion sensing. Sens. Actuators, A. 2018;274:50-56.
- [Google Scholar]
- Electrical stimulation alters protein adsorption and nerve cell interactions with electrically conducting biomaterials. Biomaterials. 2001;22(10):1055-1064.
- [Google Scholar]
- Electrically conductive composite textiles modified with graphene using sol-gel method. J. Alloy. Compd.. 2019;784:22-28.
- [Google Scholar]
- Electrochemical copolymerization of furan and 3-methyl thiophene. J. Mater. Sci.. 2004;39(7):2395-2398.
- [Google Scholar]
- Antimicrobial cotton containing N-halamine and quaternary ammonium groups by grafting copolymerization. Appl. Surf. Sci.. 2014;296:231-236.
- [Google Scholar]
- Self-assembled antibacterial coating by N-halamine polyelectrolytes on a cellulose substrate. J. Mater. Chem. B. 2015;3(7):1446-1454.
- [Google Scholar]
- Structure and performance ofBombyx mori silk modified with nano-TiO 2 and chitosan. Fibers Polym.. 2007;8(1):1-6.
- [Google Scholar]
- High-performance textile electrodes for wearable electronics obtained by an improved in situ polymerization method. Chem. Eng. J.. 2019;361:897-907.
- [Google Scholar]
- Smart fabrics and interactive textile enabling wearable personal applications: R&D state of the art and future challenges. In: 2008 30th Annual International Conference of the IEEE Engineering in Medicine and Biology Society. IEEE; 2008.
- [Google Scholar]
- Synthesis and characterization of cationic carbon black pigment with quaternary ammonium groups and its dyeing properties for wool fabrics. Colloids Surf., A. 2018;549:43-49.
- [Google Scholar]
- Phase transitions in polypyrrole and polythiophene conducting polymers demonstrated by magnetic susceptibility measurements. Phys. Rev. B. 1989;39(9):5964.
- [Google Scholar]
- Synthesis and characterization of polyaniline nanocomposites. KGK, Kaut. Gummi Kunstst.. 2013;66(9):39-46.
- [Google Scholar]
- (2016) Synthesis of Novel Heterocyclic Imine Type UV Absorbers for Application on Cotton Based Textile Materials. J. Chem. 2016
- [Google Scholar]
- Fabrication of cotton fabrics through in-situ reduction of polymeric N-halamine modified graphene oxide with enhanced ultraviolet-blocking, self-cleaning, and highly efficient, and monitorable antibacterial properties. Colloids Surf., A. 2018;555:765-771.
- [Google Scholar]
- Highly stretchable electric circuits from a composite material of silver nanoparticles and elastomeric fibres. Nat. Nanotechnol.. 2012;7(12):803.
- [Google Scholar]
- Cotton-hydrogel composite for improved wound healing: Synthesize optimization and physicochemical characterization—Part 1. Polym. Adv. Technol.. 2018;29(12):3114-3124.
- [Google Scholar]
- Impedance study of (PEO) 10LiClO4–Al2O3 composite polymer electrolyte with blocking electrodes. Electrochim. Acta. 2001;46(12):1829-1836.
- [Google Scholar]
- Structural, thermal and electrochemical cell characteristics of poly (vinyl chloride)-based polymer electrolytes. J. Power Sources. 2001;99(1–2):41-47.
- [Google Scholar]
- Dielectric behaviour of PVC-based polymer electrolytes. Solid State Ionics. 2002;152:291-294.
- [Google Scholar]
- Synthesis of a novel, biodegradable electrically conducting polymer for biomedical applications. Adv. Funct. Mater.. 2002;12(1):33-37.
- [Google Scholar]
- A review on influential behaviour of biopolishing on dyeability and certain physico-mechanical properties of cotton fabrics. Carbohydr. Polym.. 2009;76(1):1-7.
- [Google Scholar]
- Antibacterial effect of novel synthesized sulfated β-cyclodextrin crosslinked cotton fabric and its improved antibacterial activities with ZnO, TiO2 and Ag nanoparticles coating. Int. J. Pharm.. 2012;434(1):366-374.
- [Google Scholar]
- Ac conductivity and dielectric relaxation studies in AgI-based fast ion conducting glasses. Solid State Ionics. 1991;44(3–4):187-198.
- [Google Scholar]
- Photochemical and thermal reduction of cerium (IV) carboxylates. Formation and oxidation of alkyl radicals. J. Am. Chem. Soc.. 1968;90(24):6688-6698.
- [Google Scholar]
- Structures of novel antimicrobial agents for textiles - a review. Text. Res. J.. 2010;80(16):1721-1737.
- [Google Scholar]
- Stimuli-responsive hydrogels for textile functionalisation: A review Hidrogeli, obutljivi na draljaje, za funkcionalizacijo tekstilij. Tekstilec. 2017;60(2):76-96.
- [Google Scholar]
- Smart Fibres, Fabrics and Clothing: Fundamentals and Applications. Elsevier; 2001.
- New electrochemically generated organic conducting polymers. J. Electroanal. Chem. Interfacial Electrochem.. 1982;135(1):173-178.
- [Google Scholar]
- Broad ultraviolet protection by copolymerization of 2-[3-(2H-benzotriazol-2-yl)-4-hydroxyphenyl] ethyl methacrylate and 2-hydroxy-4-acryloyloxybenzophenone on cotton via admicellar polymerization. J. Appl. Polym. Sci.. 2008;108(6):4004-4013.
- [Google Scholar]
- Incorporation of the antibacterial agent, miconazole nitrate into a cellulosic fabric grafted with β-cyclodextrin. Carbohydr. Polym.. 2008;72(4):695-700.
- [Google Scholar]
- Surface modification by carboxymethy chitosan via pad-dry-cure method for binding Ag NPs onto cotton fabric. Int. J. Biol. Macromol.. 2018;111:796-803.
- [Google Scholar]
- One-pot fabrication of durable antibacterial cotton fabric coated with silver nanoparticles via carboxymethyl chitosan as a binder and stabilizer. Carbohydr. Polym.. 2019;204:42-49.
- [Google Scholar]
- Antibacterial cotton fabric grafted with silver nanoparticles and its excellent laundering durability. Carbohydr. Polym.. 2013;92(2):2088-2094.
- [Google Scholar]
- Superadsorbent with three-dimensional networks: From bulk hydrogel to granular hydrogel. Eur. Polym. J.. 2015;72:661-686.
- [Google Scholar]