Translate this page into:
Multi-method analysis of the nonlinear influence of moisture content on the surface structure and oxidation characteristics of bituminous coal
*Corresponding author: E-mail address: djhu0418@gmail.com (Dongjie Hu).
-
Received: ,
Accepted: ,
Abstract
To investigate the nonlinear influence mechanism of moisture content on the self-ignition propensity of bituminous coal, multiple advanced analytical techniques, including scanning electron microscopy-energy dispersive X-ray spectroscopy (SEM-EDS), synchronous thermal analysis, gas adsorption, closed-coal oxidation, and ReaxFF molecular dynamics simulations, were employed to conduct a multi-method analysis of coal samples with varying moisture contents. Surface morphology, elemental distribution, thermal characteristics, oxidation reactivity, and pyrolysis products were examined at the micro and molecular levels. Results revealed that under identical experimental conditions, compared to raw coal (1.3% moisture content) and other samples with moisture content above 5%, the coal sample with 5% moisture content exhibited higher surface roughness, more developed pore structure, and higher oxygen content. This sample also demonstrated the lowest ignition apparent activation energy (88.80 KJ·mol-1), highest oxygen adsorption capacity, and fastest oxygen consumption rate. ReaxFF force field simulations of Wiser coal molecule pyrolysis indicated that moisture content between 5% and 10% favored the generation of self-ignition indicator gases such as acetylene and ethylene. The self-ignition propensity of coal samples with moisture content above 5% weakened as moisture content increased. This research has revealed a nonlinear relationship between moisture content and the oxidation characteristics of coal, providing a theoretical foundation and technical guidance for optimizing coal storage and transportation conditions, enhancing coal utilization efficiency, and improving safety measures.
Keywords
Coal self-ignition
Moisture content
Molecular simulation
Oxidation characteristics
Surface morphology
1. Introduction
Moisture content plays a crucial role in affecting coal storage, transportation safety, and utilization efficiency [1,2], but its underlying mechanism remains incompletely understood. Moisture can absorb heat generated during coal self-ignition, delaying temperature rise, and inhibiting ignition [3,4], but it can also alter the coal’s surface structure, increasing adsorption sites and active groups, thereby promoting self-ignition [5,6]. Therefore, understanding how the moisture content affects the microscopic surface morphology and surface adsorption characteristics of coal, as well as the nonlinear mechanisms of moisture in the self-ignition process, is essential for improving coal storage and transportation safety and efficiency.
The impact of coal moisture content on the self-ignition process is complex and manifests differently at various stages. Studies by Jia [7] and Zhang [8] showed that moisture primarily exhibits physical inhibition effects in the early stages of coal self-ignition, reducing coal’s heat release and thermal conductivity, thereby suppressing temperature rise. However, as oxidation progresses, moisture evaporation leads to increased coal body fissures and active sites, accelerating the oxidation process. Research by Fan [9] and Liu [10] further highlighted the complex relationship between moisture content and coal self-ignition tendency, indicating that the effect of moisture on coal oxidation is closely related to coal metamorphic degree and moisture content, with coal containing less than 8% moisture being more prone to self-ignition. Studies by Li [11] and Li [12] focused on the effects of low humidity and specific moisture content ranges on coal self-ignition, enriching the understanding of moisture’s role in the coal self-ignition process. In addition to affecting the self-ignition process, moisture significantly influences the coal’s pore structure and gas adsorption characteristics, which in turn affects the occurrence of gases such as coalbed methane. Studies by Guo et al. [13], Nie et al. [14], and Zhang et al. [15] indicated that the presence of moisture occupies coal pore spaces, reducing coal’s gas adsorption capacity, especially in low-rank coals. Hao’s et al. [16] revealed that the impact of increased moisture on bituminous coal leads to reduced porosity but has little effect on coal’s sample volume and fracture development. Wang’s et al. [17] molecular simulation study explored the influence of moisture on coal gangue adsorption characteristics from a microscopic perspective, providing new insights into understanding the interaction between moisture and coal rock. Despite these valuable insights from the above studies, the relationship between water content and spontaneous combustion, surface morphology, pore structure, and adsorption properties needs to be investigated in depth and at multiple scales.
Taking into account the previous research, this study focuses on bituminous coal from Shenyang Linsheng Coal Mine (LS). Six coal samples were prepared, including raw coal (1.3% moisture content) and five with moisture contents of 5%, 10%, 15%, 20%, and 25%, achieved through water mist spraying. Scanning electron microscopy-energy dispersive X-ray spectroscopy (SEM-EDS) [18,19] was used to analyze the surface morphology and variations in C, N, O, and S elements with moisture content. Synchronous thermal analysis [20-23] measured the characteristic temperatures, oxidative exothermic properties, and activation energy changes during coal oxidation at different moisture levels. Gas adsorption experiments [24] assessed the oxygen adsorption capacity and rate under standard temperature and pressure. A self-developed closed-coal oxidation test apparatus [25-27] measured oxygen depletion and consumption rates over time for the six samples with varying moisture contents. Using the ReaxFF force field, the reaction kinetics of oxygen-coal molecular systems (Wiser bituminous coal model) with different numbers of H2O molecules were simulated [28,29], and the generation of self-ignition indicator gases was analyzed. Through multi-method analysis of the experimental results, the nonlinear influence of moisture on the surface structure and oxidation characteristics of bituminous coal was elucidated, offering valuable insights for preventing coal self-ignition and optimizing coal resource utilization.
2. Materials and Methods
2.1. SEM-EDS
A Quanta 450 Field Emission Scanning Electron Microscope (FEG-SEM) from FEI (USA) coupled with a Bruker XFlash 6|30 EDS spectrometer was used. The operational procedure included sample preparation and fixation, vacuum mode selection and adjustment, electron beam parameter adjustment, imaging condition optimization, target area selection, and EDS acquisition parameter setting and spectrum collection.
2.2. Synchronous thermal analysis experiment
A NETZSCH STA 449F5-QMS 403D synchronous thermal analyzer was used for synchronous thermal analysis. Each coal sample weighed 10 mg, with temperatures ranging from 30℃ to 800℃, a heating rate of 10℃·min-1, and a gas flow rate of 50 mL·min-1 (N2:O2 = 4:1).
2.3. Gas adsorption experiment
The oxygen adsorption characteristics of coal samples were studied using a Quantachrome Instruments H-Sorb 2600 gas adsorption analyzer. The experimental conditions were set at 25℃ and 0.1 MPa. Prior to the experiment, coal samples with different moisture contents were dried under a vacuum at 105℃ for 4 hours.
2.4. Closed-coal sample oxidation experiment
A self-developed closed-coal oxidation device was employed to study the oxygen consumption changes over time during low-temperature oxidation of bituminous coal [25-27], The relationship between the oxygen consumption rate and oxygen content of the coal samples was analyzed.
2.5. Molecular system pyrolysis simulation
Using MestReNova software, the Wiser coal molecular model was constructed and optimized in the Forcite module of Material Studio [30]. Reaction systems containing oxygen, coal molecules, and varying numbers of H2O molecules were constructed. The AC module was used to optimize density, and the lowest energy system model was selected after 30 annealing optimizations in the Forcite module.
Molecular reaction kinetics calculations were performed using Large-scale Atomic/Molecular Massively Parallel Simulator (LAMMPS) software with the ReaxFF force field. The simulation conditions included an microcanonical ensemble (NVE) ensemble, Berendsen temperature control, a time step of 0.2 fs, a heating rate of 5 K/ps, a temperature range of 300–1000 K, and recording reaction trajectories every 200 K.
3. Results and Discussion
3.1. SEM-EDS test spectra
The SEM-EDS experimental results are shown in Figure 1. EDS spectral scanning revealed that the carbon content peaked highest at 92.57% with 25% moisture content and its lowest at 87.32% with 5% moisture content, possibly due to fluctuations in organic matter content and coal structure. The oxygen content was highest at 9.03% with 5% moisture content and lowest at 4.51% with 25% moisture content.
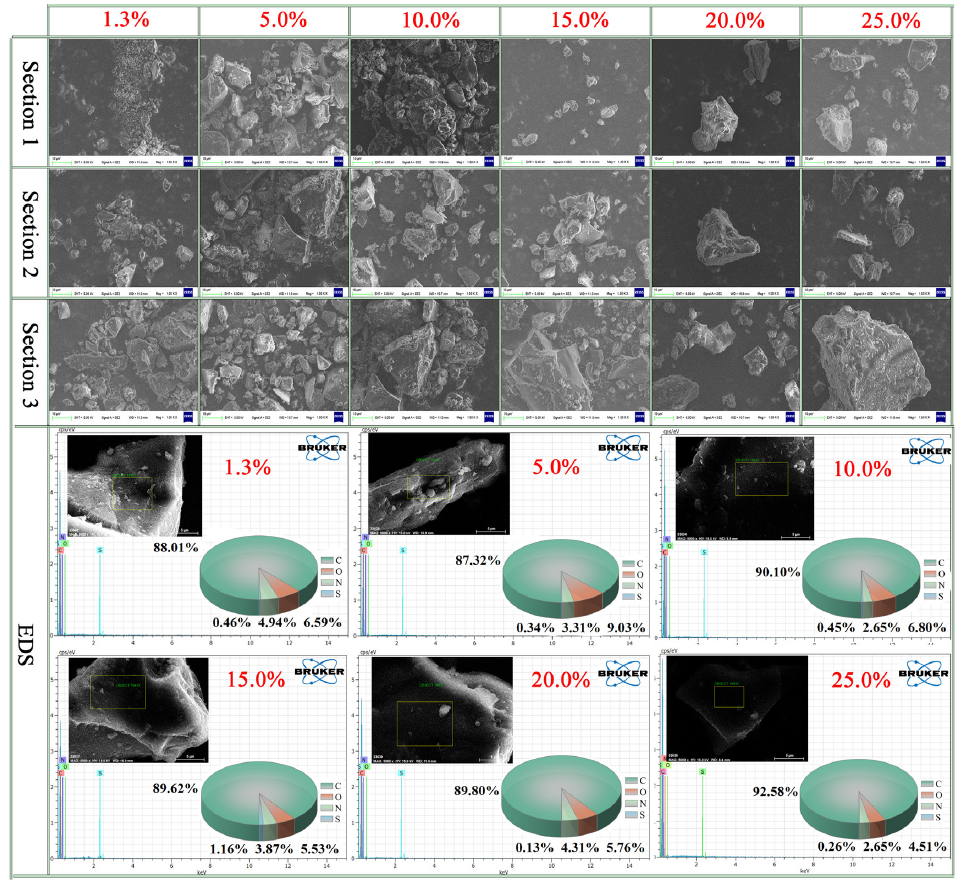
- Scanning electron microscopy-energy dispersive X-ray spectroscopy (SEM-EDS) test results of coal samples with different moisture contents.
This variation is closely related to the presence of moisture, as higher moisture content may lead to restructuring of the coal surface, altering the exposure degree of surface elements and thus affecting the oxygen content detected by SEM-EDS. In addition, higher moisture content may dissolve more water-soluble oxygen-containing compounds in the coal, resulting in decreased oxygen content. Nitrogen content fluctuated irregularly with changes in moisture content, peaking at 15% (3.87%) and reaching its lowest at 10% and 25% (2.65%), which is related to the distribution of mineral matter and organic nitrogen compounds in the coal samples. The sulfur content was highest at 15% moisture content (1.16%) and lowest at 20% (0.13%), with its variation possibly related to the formation and decomposition of sulfides and sulfates in the coal samples. Numerous scholars’ research has shown that higher O element content in coal correlates with increased self-ignition propensity [31,32], indicating that the coal sample with 5% moisture content has the strongest self-ignition tendency.
The SEM analysis of three regions from each coal sample highlighted notable differences in pore structure and surface morphology, which directly influence their self-ignition properties. The coal sample with 1.3% moisture content exhibited a relatively rough surface and large pores in all three regions, with these pores being unevenly distributed and partially interconnected, facilitating oxygen entry and diffusion and increasing the risk of self-ignition. Compared with other samples, the 5% moisture content sample showed the roughest surface, with large and unevenly distributed pores and numerous interconnected pores, making it the most prone to self-ignition among all samples. In contrast to the 5% moisture content sample, the 10.0% moisture content sample had a smoother surface but medium-sized pores with uniform distribution and good interconnectivity between pores, also presenting a high self-ignition risk.
The 15.0% moisture content sample showed characteristics intermediate between other samples in the three regions, with a relatively rough surface and medium-sized pores uniformly distributed. Both the 25.0% and 20.0% moisture content samples exhibited relatively flat surfaces with smaller, densely packed pores in all three regions. In particular, the 25.0% moisture content sample had a complex and compact pore structure, greatly limiting oxygen diffusion and making it the most difficult to self-ignite. Although the 20.0% moisture content sample had dense pores, its surface was slightly flatter compared to the 25.0% moisture content sample.
3.2. Synchronous thermal analysis results
The purpose of synchronous thermal analysis experiments was to study the oxidation properties of coal samples in their moist state. Therefore, samples were not dried before the experiments. The results of synchronous thermal analysis experiments are shown in Figure 2.
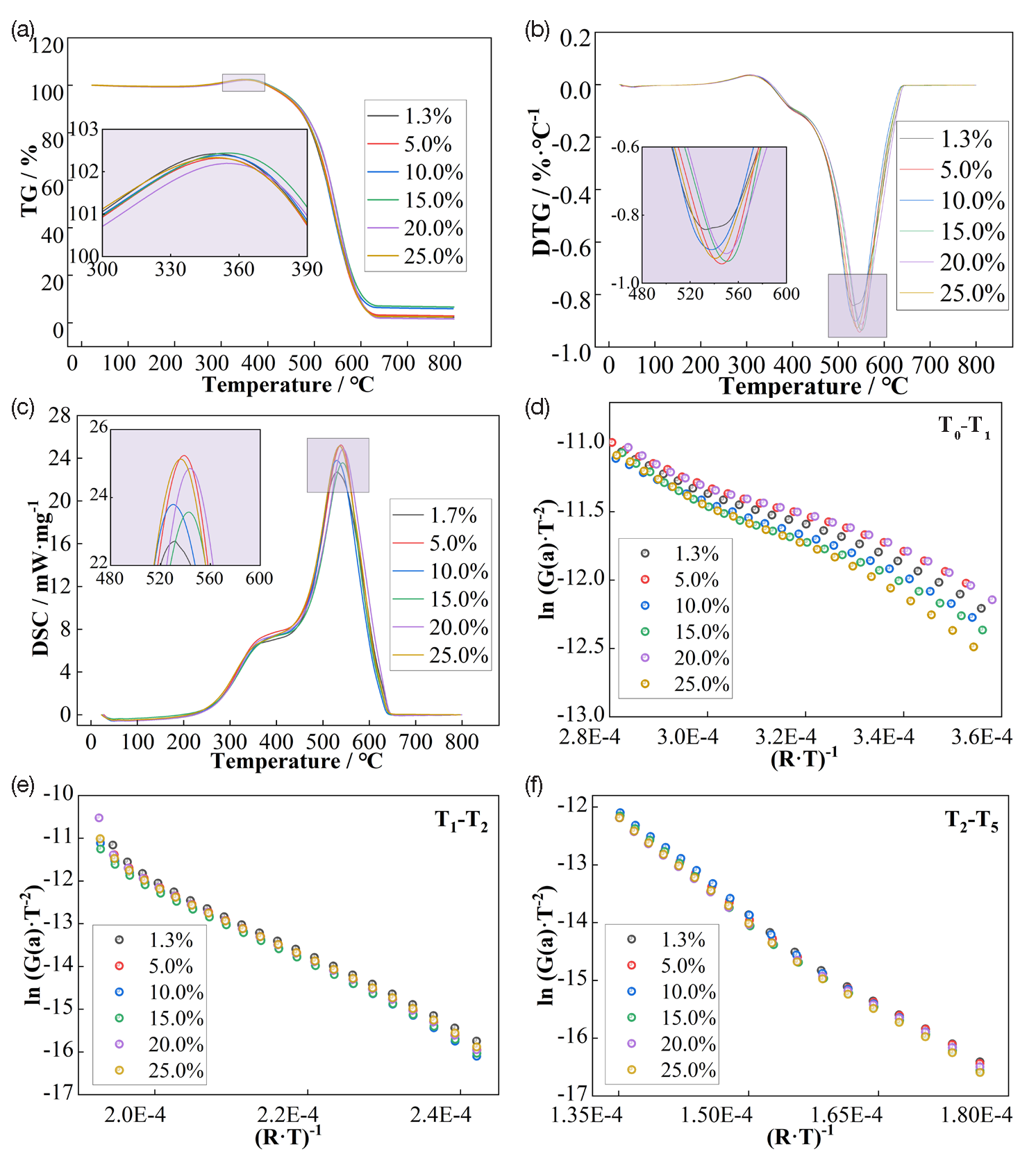
- (a-f) Results of synchronous thermal analysis experiments.
Characteristic temperature points for coal samples with different moisture contents were determined according to the method in reference [33,34]: initial temperature (T0), the endpoint of water loss and weight loss (T1), maximum oxygen absorption weight gain temperature (T2), ignition point temperature (T3), maximum weight loss rate point temperature (T4), and burnout temperature (T5). The thermal parameters obtained from the DSC curves in Figure 2(c) are shown in Table 1. The ignition point temperature of the LS coal sample was lowest at 5% moisture content, about 9℃ lower than that of the raw coal (1.3% moisture content).
Moisture content/% |
T0 /°C |
T1 /°C |
T2 /°C |
T3 /°C |
T4 /°C |
T5 /°C |
Evaporative heat absorption peak |
Oxidative exothermic peak |
Combustion exothermic peak |
|||
---|---|---|---|---|---|---|---|---|---|---|---|---|
peak position /°C |
Heat release/ (J·g-1) |
peak position /°C |
Heat release/ (J·g-1) |
peak position /°C |
Heat release/ (J·g-1) |
|||||||
1.3 | 25.8 | 182.6 | 353.4 | 486.2 | 544.0 | 632.0 | 81.0 | -75.5 | | 378.7 | 503.9 | | 531.7 | 3140.8 |
5.0 | 22.3 | 180.7 | 351.4 | 477.4 | 548.3 | 634.8 | 82.7 | -81.3 | 377.6 | 528.5 | 538.9 | 3295.7 |
10.0 | 27.4 | 183.8 | 351.3 | 484.3 | 546. V | 634.8 | I 80.0 | -80.0 | 377.6 | 482.8 | 530.1 | 3083.6 |
15.0 | 23.9 | 181.0 | 354.6 | 482.2 | 550.7 | 635.1 | 1 80.0 | -83.4 | 380.5 | 508.8 | 542.6 | 3041.8 |
20.0 | 24.5 | 189.4 | 348.8 | 492.4 | 531.3 | 634.8 | 1 83.3 | -81.2 | 378.9 | 514.0 | 543.8 | 3309.0 |
25.0 | 26.2 | 190.4 | 1353.2 | 1486.4 | 538.1 | K35.6 | 1 79.3 | -87.1 | 379.18 | 515.6 | 539.1 | 3324.4 |
The peak values and peak areas in the DSC curves (Figure 2(c)) from the synchronous thermal analysis experiments of coal samples can be used to evaluate the thermal stability of coal samples and related thermodynamic information. Table 1 shows that in the water evaporation phase of LS coal samples, the peak temperatures were relatively stable, ranging from 79.3 to 83.3°C. This suggests that moisture in coal samples with varying water content primarily exists as free water and is minimally influenced by the overall moisture content. The endothermic amount increased slightly with increasing moisture content, but the change was not significant. In the oxidative exothermic stage, the peak temperatures did not differ greatly, around 380°C, but the oxidative exothermic amount was highest at 528.48 J·g-1 for the coal sample with 5% moisture content.
Based on the TG curves in Figure 2(a), the reaction process of coal can be roughly divided into three: T0-T1 water loss and weight loss stage, T1-T2 oxidation weight gain stage (oxygen absorption weight gain stage), and T2-T5 combustion stage. According to the Arrhenius formula, the corresponding activation energies were calculated using the TG curve data for each stage: water loss activation energy, ignition apparent activation energy, and combustion activation energy.
Based on the references [35,36] and describing the coal oxidation process as a first-order reaction, the calculation formula for the activation energy of coal at each stage can be simplified as Eqs. (1) and (2):
Where β is the heating rate, ℃·min-1; A is the pre-exponential factor, s-1; E is the activation energy, KJ·mol-1; R is the molar gas constant, 8.314 J·(mol·k)-1; α is the conversion rate of coal oxidation at each stage (calculated by Eq. (2)), %; m0 is the initial weight of the coal sample at each stage, mg; m∞ is the final weight of the coal sample at each stage, mg; m is the weight of unreacted coal sample at t, mg.
Defining the left side of Eq. (1) as the dependent variable (Y-axis coordinate) and (R·T)-1 as the independent variable (X-axis coordinate), the relationships between ln(G(a)·T-2) and (R·T)-1 for different stages of coal samples are shown in Figure 2(d), 2(e), and 2(f), obtained from the TG curves. By linear fitting of the experimental results in Figure 2, the reaction activation energies of coal samples at different stages were obtained, as shown in Table 2.
Moisture content /% | T0-Ti / KJ-mol’1 | TrT2 / KJ-mol’1 | T2-T5 / KJ-mol’1 | Order of reaction n |
---|---|---|---|---|
1.30 | 14.22 | 89.50 | 102.42 | 1 |
5.00 | 12.98 | 88.80 | 104.12 | 1 |
10.00 | 14.71 | 92.51 | 107.41 | 1 |
15.00 | 15.94 | 89.86 | 106.17 | 1 |
20.00 | 13.26 | 89.95 | 102.82 | 1 |
25.00 | 17.84 | 93.65 | 106.09 | 1 |
From the activation energy of the T0-T1 water loss and weight loss stage, when the moisture content is 5% and 10%, the water loss activation energy is lower than that of raw coal and other moisture-content coal samples. This indicates that when the coal sample is at 5–10% moisture content, the water in the coal is more easily evaporated. When the moisture content of coal is in this range of 5–10%, although the presence of water in coal occupies a certain number of adsorption sites, forming hydrogen bonds stronger than intermolecular interactions, it increases the porosity in coal during the heating and evaporation process, making it easier for adsorbed gas molecules to desorb and leave the coal body. When there is more water in the coal and water molecules occupy all pores, the hydrogen bonding between coal and water molecules, as well as between water molecules, along with intermolecular forces and cohesive forces, make it more difficult for water molecules to evaporate completely.
The activation energy of the T1-T2 oxidation weight gain stage, known as the ignition apparent activation energy [37], indicates the minimum energy required for chemical reactions between coal macromolecules and oxygen. By comparing this value, the self-ignition tendency of different coal samples can be assessed. As shown in Table 2, the ignition apparent activation energy of the LS coal sample at 5% moisture content is 88.80 KJ/mol, lower than that of raw coal at 89.50 KJ/mol, indicating a higher propensity for self-ignition in the 5% moisture content sample.
3.3. Gas adsorption experiment results
Gas adsorption experiments were conducted to examine how coal’s oxygen adsorption behavior varies with different moisture contents under standard temperature and pressure, simulating environmental conditions in goaf areas. Figure 3 illustrates the oxygen adsorption curves for six groups of LS raw coal and coal samples with moisture contents of 5%, 10%, 15%, 20%, and 25%.
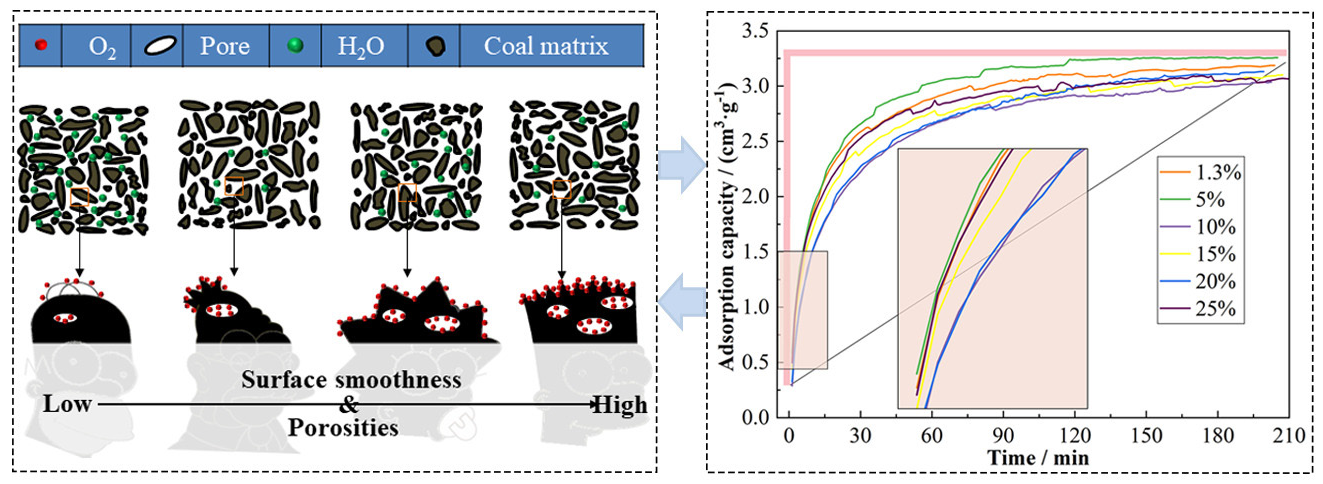
- Experimental curves of oxygen adsorption of coal samples with different moisture content.
After drying, coal samples with varying moisture contents demonstrated different oxygen adsorption capacities under standard conditions. There was no clear positive or negative correlation between their adsorption capacities and moisture contents. The coal sample with 5% moisture content exhibited the fastest oxygen adsorption rate and the highest amount of adsorbed oxygen. With increasing moisture content, the rates and amounts of oxygen adsorption displayed varied trends compared with raw coal. Specifically, samples with 25% and 10% moisture content had similarly lower oxygen adsorption amounts compared to those with 15% and 20% moisture content. However, the oxygen adsorption rate for the 25% sample exceeded that of the 10%, 15%, and 20% samples but was still lower than that of raw coal and the 5% sample. This behavior is likely due to higher moisture content leading to more hydrogen bonds between water and coal functional groups (such as carbonyl and hydroxyl), which drying alone cannot completely remove, leaving residual moisture that decreases oxygen affinity and increases resistance to gas diffusion.
Combined with the SEM test results, when the moisture content is below the critical value, water has little effect on the coal morphology, neither significantly increasing the surface roughness and the size and number of internal pores, nor can it interact with coal to form more adsorption sites and oxygen-philic groups on the coal surface. When the moisture content is at the critical value and after water evaporation, the coal surface becomes roughest, with increased fissures and pores, providing more active sites for gas adsorption.
When the moisture content exceeds the critical value, the effect of water on coal adsorption performance is mainly negative. As moisture content increases, water forms hydrogen bonds with functional groups in coal, thereby reducing the affinity between coal and gas and lowering the adsorption amount and rate of coal. In addition, the presence of water hinders gas diffusion, limiting its adsorption rate. After exceeding the critical moisture content, a further increase in water has a gradually weakening effect on coal adsorption performance. At this point, the coal pores are basically occupied by water, and further increase in moisture content has little effect on porosity and specific surface area.
3.4. Closed-coal sample oxidation test
This study utilized a self-developed closed-coal oxidation test device to monitor the oxygen volume fraction in a closed circulation pipeline for Linsheng Mine coal samples with different moisture contents under standard temperature and pressure. The relationship between oxygen consumption rate and oxygen volume fraction was calculated based on these values. The test device, principles, and results are shown in Figure 4. As can be seen from Figure 4, the oxygen volume fraction decreases exponentially with increasing test time. Due to the increase in the relative content of other gases, competitive adsorption occurs among different gas molecules, causing active sites on the coal molecule surface to be occupied. Although coal can still react with oxygen in this state, the reaction rate is very slow, and this state is called the “asymptotic oxygen equilibrium” state. The calculated oxygen consumption rate of coal under standard temperature and pressure is positively correlated with the oxygen volume fraction, and there is a linear function relationship between the two.
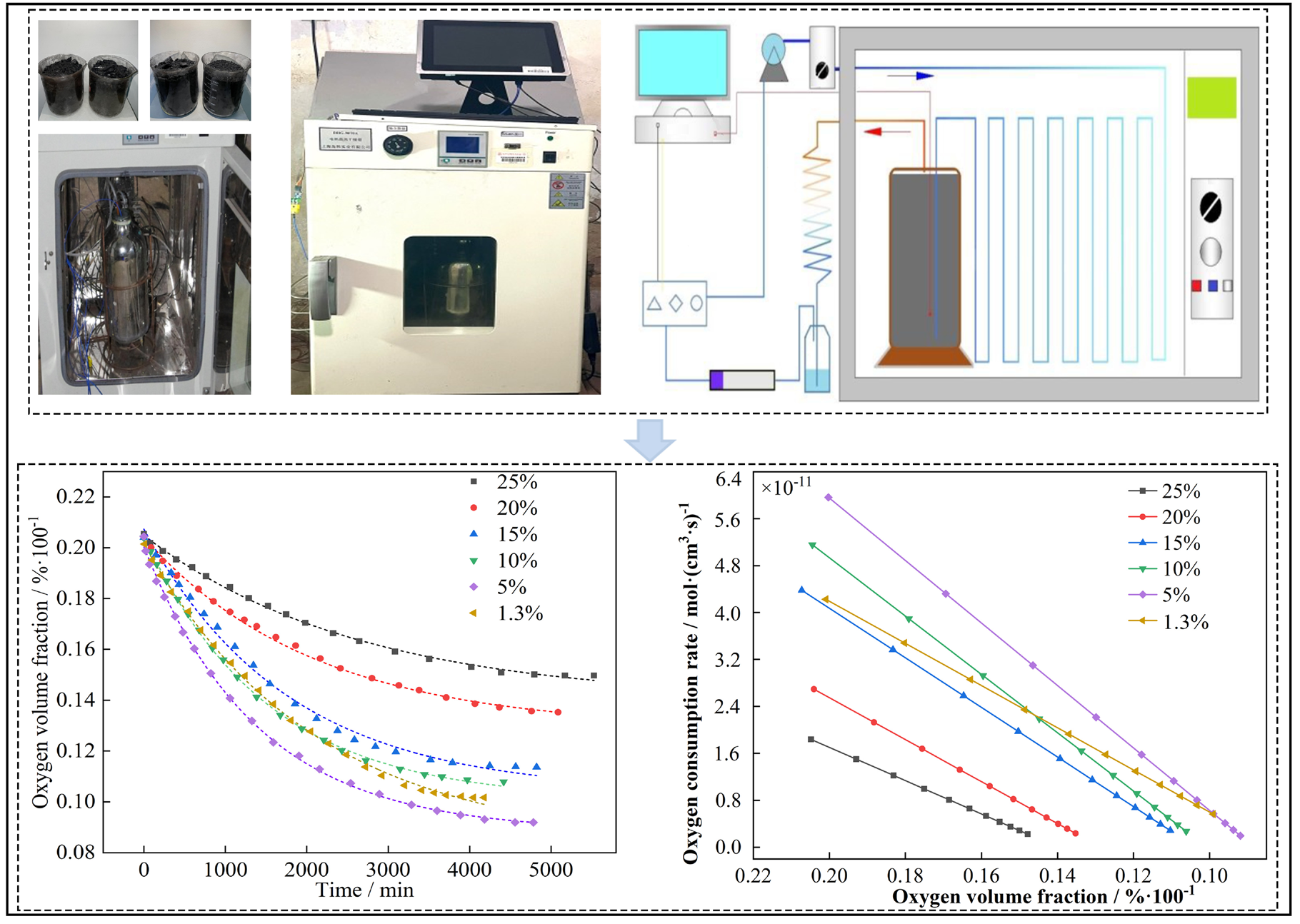
- Closed-coal oxidation test device and test results.
The calculation principles of the test parameters are as follows [27]:
Assuming that the oxygen volume fraction C(τ) in the sealed container approximately follows a negative exponential function distribution, i.e.,
Where C0 is the oxygen volume fraction value in the coal sample container before the closed-coal oxidation test begins; λc is the decay rate of the oxygen volume fraction in the coal sample container; Cb is the oxygen volume fraction value in the coal sample container when the test reaches the “asymptotic oxygen equilibrium” state; τ is the test time, min.
Taking the logarithm of Eq. (3) yields an equation suitable for linear regression, and regression analysis determines λc. Eq. (4) can be derived.
The rate of change in molar mass of oxygen consumption for the coal sample in the container:
Where is the volumetric oxygen consumption rate of the coal sample in the closed-coal oxidation test, mol·(cm3·s)-1.
Substitute Eq. (3) into Eq. (5) to obtain for oxygen volume fraction C(τ):
Eq. (6) indicates that the volumetric oxygen consumption rate of the coal sample container is linearly proportional to the oxygen volume fraction. It suggests that for loosely packed coal bodies, the oxygen consumption rate directly relates to the oxygen volume fraction.
When examining the influence of moisture on closed-coal oxidation, the 5% moisture content sample consumed the most oxygen under standard conditions, resulting in the lowest oxygen volume fraction (approximately 9.2%) at equilibrium, with the highest oxygen consumption rate. Conversely, the 25% moisture content sample had the highest oxygen volume fraction (about 15%) at equilibrium, with the lowest oxygen consumption rate. The oxygen volume fractions for raw coal and the 10% moisture content sample at equilibrium were 10.7% and 10.1%, respectively, with minimal differences. However, the oxygen consumption rate for raw coal was initially higher than that for the 10% sample, but as oxidation continued, the rate for the 10% sample surpassed that of raw coal. This might be due to the decrease in moisture content and the increase in reactive sites over time for the 10% sample.
From the results of the closed-coal oxidation test, the effect of moisture on the oxygen consumption characteristics of coal samples under standard temperature and pressure is nonlinear. When oxidizing under standard temperature and pressure, coal samples with 5% moisture content may be in a range where moisture promotes the oxidation reaction rate. The presence of moisture may promote certain hydrolysis reactions or other water-related reactions that consume oxygen. At the same time, moisture may provide active sites during the reaction process, promoting the oxidation reaction. Therefore, coal samples with this moisture content may exhibit higher reaction rates and greater oxygen consumption.
Furthermore, different moisture contents may lead to the formation of different products in the oxidation reaction. At 5% moisture content, coal may form some highly reactive intermediate products during oxidation, leading to faster oxygen consumption. However, when the moisture content exceeds a certain threshold, the products formed may consume less oxygen, resulting in slower oxygen consumption. When the moisture content exceeds 5%, this moisture-induced oxygen consumption gradually surpasses the moisture-promoted reaction, leading to reduced oxygen consumption.
When the moisture content is very low, there may not be enough moisture to participate in the oxidation reaction effectively. Under low-temperature conditions, moisture may take a longer time to be released and participate in the reaction. Therefore, coal samples with low moisture content may require more time to form active sites, resulting in slower reaction rates and less oxygen consumption. Under low moisture content conditions, coal oxidation may choose some other reaction pathways, which are slower compared to the pathways at higher moisture content. This may lead to reduced reaction rates and decreased oxygen consumption.
3.5. Molecular system pyrolysis simulation
In simulation studies on the oxidation and adsorption characteristics of bituminous coal molecules, scholars often use the Wiser coal molecular model [38-41]. In this study, the bituminous coal molecular model and coal-oxygen reaction systems with different water molecule contents were used to perform pyrolysis simulations under the ReaxFF force field. The changes in the total number of molecules in the reaction system and the number of coal self-ignition indicator gas molecules (CO2, CO, C2H2, C2H4) generated during the pyrolysis process were recorded as a function of calculation time [35,42]. The molecular modeling and simulation results are shown in Figure 5.
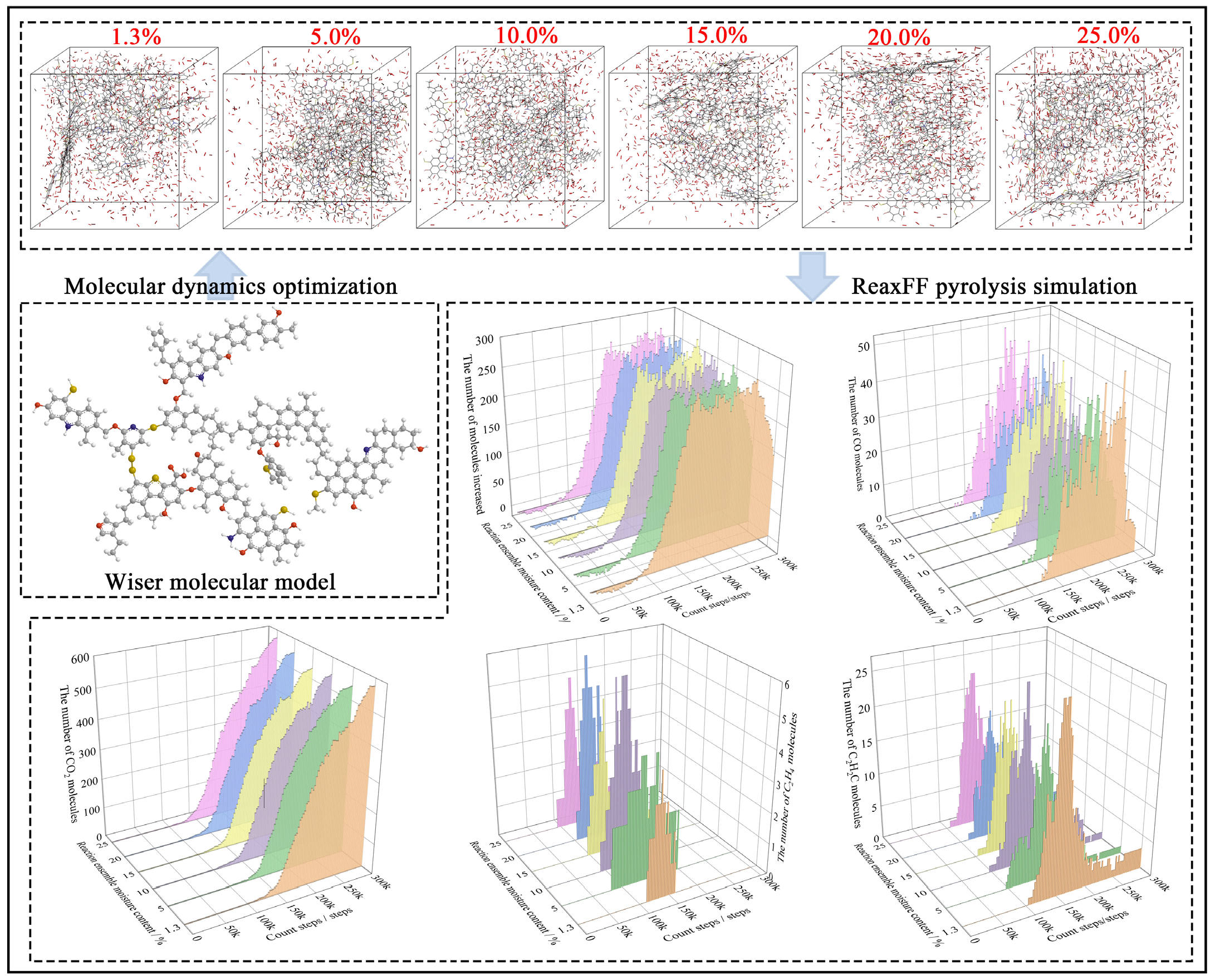
- Modeling and pyrolysis simulation of coal-oxygen molecular reaction systems with different moisture contents. ReaxFF: Reactive force field
The results in Figure 5 show that there is little difference in the total number of molecules during the pyrolysis process of reaction systems with different moisture contents. The changes in the number of coal self-ignition indicator gas molecules such as CO2, CO, C2H2, and C2H4 recorded in the pyrolysis simulation results have the following characteristics:
When the water molecule content in the reaction system is different, there are certain differences in the number of C2H2 molecules generated during the coal sample pyrolysis process and their initial generation time. The coal-oxygen reaction system with 10% moisture content was the earliest to show C2H2 molecules during the pyrolysis calculation process, followed by the coal-oxygen reaction system with 5% moisture content, while the coal-oxygen reaction system with 20% moisture content generated C2H2 molecules the latest. In terms of the amount of C2H2 molecules generated, the coal-oxygen reaction system of raw coal had the highest peak content during pyrolysis, and the stable amount of C2H2 molecules was also the highest in the later stages of calculation.
In terms of the generation of C2H4 molecules, the coal-oxygen reaction system with 5% moisture content was the earliest to show ethylene (C2H4) molecules during the pyrolysis calculation process. Under this moisture content condition, the existence time of ethylene molecules in the reaction system was the longest, but the peak content of generated C2H4 molecules was relatively small (close to that of raw coal).
In the pyrolysis process of the coal-oxygen reaction system, the generation of CO molecules for the 5% moisture content sample occurred slightly later than for raw coal but earlier than for the 25% and 20% moisture content systems. The amount of CO gas produced during pyrolysis was roughly the same across different moisture content systems.
The coal-oxygen reaction system with raw coal moisture content generated CO2 molecules slightly earlier than the other groups and the early generation amount of CO2 molecules was relatively large. The final number of CO2 molecules generated at the end of the reaction was also the highest.
The reasons for the earlier generation of ethylene and acetylene in coal samples with 5% and 10% moisture content lie in the balancing effect of moisture in the coal pyrolysis process, which both facilitates the cooling of the coal sample and provides suitable conditions for the decomposition of organic matter, causing the generation of volatile gases to occur earlier than under other moisture content conditions. Specifically:
Moderate moisture promotes coal pyrolysis: In the moisture content range of 5∼10%, the presence of water helps disperse and absorb heat from the coal sample. The cooling effect of moisture on the coal heating process inhibits rapid temperature rise, thereby slowing down the coal pyrolysis rate. However, moderate moisture does not excessively inhibit the pyrolysis of organic matter but provides appropriate temperature and medium conditions for the decomposition of organic substances.
Pyrolysis leads to the release of volatile gases: Coal pyrolysis refers to the decomposition of organic matter in coal into volatile gases and coke under high-temperature conditions. While heating with moderate moisture content, volatile organic matter in the coal sample is relatively easily released. These volatile organic compounds include ethylene and acetylene, which are important indicator gases in the coal self-ignition process.
Balanced influence of moisture on gas generation: With moderate moisture content, while water aids in cooling the coal, it also provides sufficient activity to promote the decomposition of organic matter. This balance results in a higher rate of gas generation under moderate moisture conditions. Ethylene and acetylene are generated during the high-temperature pyrolysis process of coal; hence, moderate moisture helps promote the earlier generation of these gases.
Synergistic effect of pyrolysis rate and gas generation: Moderate moisture content results in a moderate rate of organic matter pyrolysis in coal under high-temperature conditions while maintaining sufficient reaction activity. This synergistic effect causes coal to release gases such as ethylene and acetylene earlier under moderate moisture content conditions.
High moisture content delayed the generation of gases such as ethylene and acetylene, primarily due to moisture’s impact on coal pyrolysis and gas production processes. The main reasons include the following:
Moisture inhibits the coal pyrolysis process: When the moisture content of the coal sample is high, the heat absorption effect of water causes the temperature to rise slowly during the heating process. However, moisture simultaneously inhibits the pyrolysis of organic matter. Coal pyrolysis refers to the process of organic matter decomposing into volatile gases at high temperatures, but the presence of moisture may absorb some heat, causing the coal to reach the decomposition temperature of volatile organic matter more slowly.
Moisture reduces the coal sample’s temperature: The heat absorption effect leads to a slower temperature rise and a relatively lower final temperature of the coal. During heating, high moisture content coal samples experience slow temperature increases due to water evaporation and heat absorption, resulting in a relatively lower final temperature.
-
Inhibition of volatile gas release: Due to the inhibitory effect of moisture on the pyrolysis of organic matter, high moisture content may lead to a slower decomposition rate of volatile organic matter, slowing down the release of gases such as ethylene and acetylene. Under high moisture content conditions, volatile organic matter requires a higher temperature to be released, and the release rate is also relatively slow.
4. Conclusions
This study employed multi-method experimental methods, including SEM-EDS, synchronous thermal analysis, gas adsorption, closed-coal oxidation, and molecular system ReaxFF pyrolysis simulation to systematically investigate the nonlinear influence of moisture content on the surface structure and oxidation characteristics of bituminous coal. The main conclusions are as follows:
Through multi-method methods, including SEM-EDS, synchronous thermal analysis, gas adsorption, closed-coal oxidation, and ReaxFF pyrolysis simulation, this study systematically analyzed the nonlinear influence of moisture on the surface structure and oxidation characteristics of bituminous coal. A comprehensive analysis of the results revealed a significant nonlinear relationship between coal moisture content and self-ignition, with a critical moisture content (5% in this study) having significant impact.
At 5% moisture content, SEM-EDS results showed that the LS coal sample surface had high roughness and developed pores, conducive to oxygen adsorption and diffusion; synchronous thermal analysis indicated the lowest ignition apparent activation energy (88.80 KJ•mol⁻1); gas adsorption and closed-coal oxidation tests quantified the highest oxygen adsorption capacity and fastest oxygen consumption rate at this moisture level; molecular simulations confirmed that 5–10% moisture content favors self-ignition indicator gas generation. The consistency across these multi-method results reveals the intrinsic link between surface structure and oxidation activity of coal under critical moisture conditions, elucidating the microscopic mechanism of moisture’s nonlinear impact on self-ignition.
Below the critical moisture value, the coal surface is relatively flat with low porosity, unfavorable for oxygen adsorption and diffusion, leading to a reduced self-ignition tendency as moisture decreases; above the critical value, excess water occupies coal’s active sites, hindering oxygen diffusion and oxidation, thus weakening self-ignition as moisture increases. This nonlinear pattern is consistently reflected in multiple results, including SEM-observed surface morphology changes, higher ignition apparent activation energy from thermal analysis, and decreased oxygen adsorption and consumption rates in gas adsorption and closed oxidation tests.
Acknowledgment
The authors acknowledge the financial support from the National Natural Science Foundation of China (51774170) & (51974149).
CRediT authorship contribution statement
Yu Liu & Yan Chen: Data curation, Writing – original draft. Dongjie Hu: Investigation, Methodology. Zongxiang Li & Chunhua Zhang: Data curation, Funding acquisition. Xiaodong Zhang: Supervision.
Declaration of competing interest
The authors declare that they have no known competing financial interests or personal relationships that could have appeared to influence the work reported in this paper.
Declaration of generative AI and AI-assisted technologies in the writing process
The authors confirm that there was no use of AI-assisted technology for assisting in the writing of the manuscript and no images were manipulated using AI.
References
- The effect of moisture content and air-drying on spontaneous combustion characteristics of two Turkish lignitesa. Fuel. 2003;82:1685-1693. https://doi.org/10.1016/S0016-2361(02)00402-7
- [Google Scholar]
- Study on the effect of extraneous moisture on the spontaneous combustion of coal and its mechanism of action. Energies. 2020;13:1969. http://dx.doi.org/10.3390/en13081969
- [CrossRef] [Google Scholar]
- Experiment study on moisture affected to critical temperature of coal spontaneous combustion. Coal Science and Technology. 2015;43:65-68+14. Available from: https://www.mtkxjs.com.cn//en/article/id/4e7546ae-5be0-4831-9120-f2eb3610523b
- [Google Scholar]
- The study of the critical moisture content at which coal has the most high tendency to spontaneous combustion. International Journal of Coal Preparation and Utilization. 2013;33:117-127. https://doi.org/10.1080/19392699.2013.769435
- [CrossRef] [Google Scholar]
- Effect of moisture on adsorption of methane on coal. Journal of Xi’an University of Science and Technology. 2011;31:379-382+387. http://dx.doi.org/10.1177/0263617418800905
- [PubMed] [Google Scholar]
- Effects of liquid water on coalbed methane adsorption characteristicsbased on the adsorption kinetic theory. Journal of China Coal Society. 2014;39:518-523. http://dx.doi.org/10.13225/j.cnki.jccs.2013.1260
- [Google Scholar]
- Experimental study on thermal characteristics of spontaneouscombustion process of coal with different moisture. Journal of China Coal Society. 2020;45:346-352. https://dx.doi.org/10.13225/j.cnki.jccs.2019.1080
- [Google Scholar]
- Study on the effect and mechanism of foreignmoisture on coal spontaneous combustion. Journal of Fuel Chemistry and Technology. 2020;48:1-10.
- [Google Scholar]
- Study on spontaneous combustion tendency of coals with different metamorphic grade at low moisture content based on TPO-DSC. Energies. 2019;12:3890. https://doi.org/10.3390/en12203890
- [CrossRef] [Google Scholar]
- Molecular structure model construction and pyrolysis mechanism study on low-rank coal by experiments and ReaxFF simulations. Journal of Analytical and Applied Pyrolysis. 2024;178:106387. http://dx.doi.org/10.1016/j.jaap.2024.106387
- [CrossRef] [Google Scholar]
- Low-temperature oxidation and self-heating accelerated spontaneous combustion properties of a Yima formation bituminous coal with various moisture contents. International Journal of Coal Preparation and Utilization. 2022;42:2722-2741. https://doi.org/10.1080/19392699.2021.1885382
- [CrossRef] [Google Scholar]
- Mutual conversion of active sites and oxygen-containing functional groups during low-temperature oxidation of coal. Energy. 2023;272:127151. https://doi.org/10.1016/j.energy.2023.127151
- [CrossRef] [Google Scholar]
- Experimental study on the effect of moisture on low-rank coal adsorption characteristics. Journal of Natural Gas Science and Engineering. 2015;24:245-251.
- [CrossRef] [Google Scholar]
- Sorption charateristics of methane among various rank coals: impact of moisture. Adsorption. 2016;22:315-325. http://dx.doi.org/10.1007/s10450-016-9778-9
- [CrossRef] [Google Scholar]
- Methane sorption behavior on tectonic coal under the influence of moisture. Fuel. 2022;327:125150. https://doi.org/10.1016/j.fuel.2022.125150
- [CrossRef] [Google Scholar]
- Experimental study on the effect of moisture content on bituminous coal porosity based on 3D reconstruction of computerized tomography. Natural Resources Research. 2020;29:1657-1673. https://doi.org/10.1007/s11053-019-09528-y
- [CrossRef] [Google Scholar]
- Experimental study and molecular simulation of spontaneous combustion of coal gangue by oxidation under different water contents. International Journal of Coal Preparation and Utilization. 2023;43:2046-2064. http://dx.doi.org/10.1080/19392699.2022.2151588
- [CrossRef] [Google Scholar]
- Poroperm characteristics of high-rank coals from Southern Qinshui Basin by mercury intrusion, SEM-EDS, nuclear magnetic resonance and relative permeability analysis. Journal of Natural Gas Science and Engineering. 2018;51:116-128. http://dx.doi.org/10.1016/j.jngse.2018.01.013
- [CrossRef] [Google Scholar]
- Fly ash characterization by SEM–EDS. Fuel. 2006;85:2537-2544. http://dx.doi.org/10.1016/j.fuel.2006.05.016
- [Google Scholar]
- Reactivity and catalytic effect of coals during combustion: Thermogravimetric analysis. Energy. 2024;291:130353. https://dx.doi.org/10.1016/j.energy.2024.130353
- [CrossRef] [Google Scholar]
- Inhibiting effect investigation of ammonium dihydrogen phosphate on oxidative pyrolysis characteristics of bituminous coal. Fuel. 2023;333:126352. https://dx.doi.org/10.1016/j.fuel.2022.126352
- [CrossRef] [Google Scholar]
- Investigation on oxidative pyrolysis characteristics of bituminous coal through thermal analysis and density functional theory. Applied Energy. 2023;349:121680. http://dx.doi.org/10.1016/j.apenergy.2023.121680
- [CrossRef] [Google Scholar]
- Gasification kinetic and thermodynamic parameters of fine coal using thermogravimetric analysis. Energy. 2023;268:126666. http://dx.doi.org/10.1016/j.energy.2023.126666
- [CrossRef] [Google Scholar]
- Thermodynamic variation law and influence mechanism of low-temperature oxidation of lignite samples with different moisture contents. Energy. 2023;262:125605. http://dx.doi.org/10.1016/j.energy.2022.125605
- [CrossRef] [Google Scholar]
- Effect of collapse column structure on oxidation and physicochemical characteristics of bituminous coal. Arabian Journal of Chemistry. 2024;17:105649. http://dx.doi.org/10.1016/j.arabjc.2024.105649
- [CrossRef] [Google Scholar]
- Dynamic distribution and prevention of spontaneous combustion of coal in gob-side entry retaining goaf. Plos One. 2022;17:e0267631. http://dx.doi.org/10.1371/journal.pone.0267631
- [CrossRef] [PubMed] [Google Scholar]
- Critical oxygen volume fraction of smothering quenched zone based on closed oxygen consumption experiment. Journal of China Coal Society. 2017;42:1776-1781. http://dx.doi.org/10.1371/journal.pone.0267631
- [Google Scholar]
- Simulation strategies for ReaxFF molecular dynamics in coal pyrolysis applications: A review. Journal of Analytical and Applied Pyrolysis. 2023;170:105882. https://doi.org/10.1016/j.jaap.2023.105882
- [CrossRef] [Google Scholar]
- Role of moisture content in coal oxidation during the spontaneous combustion latency. Energy. 2024;291:130336. https://doi.org/10.1016/j.energy.2024.130336
- [CrossRef] [Google Scholar]
- Conversion of bituminous coal to liquids and gases: Chemistry and representative processes. In: Magnetic Resonance. Netherlands: Springer; 1984.
- [Google Scholar]
- Characteristics of lean oxygen combustion and dynamic microreaction process of water-soaked coal. Fuel. 2023;332:126010. http://dx.doi.org/10.1016/j.fuel.2022.126010
- [CrossRef] [Google Scholar]
- Multi-scale pore fractal characteristics of differently ranked coal and its impact on gas adsorption. International Journal of Mining Science and Technology. 2023;33:389-401. http://dx.doi.org/10.1016/j.ijmst.2022.12.006
- [CrossRef] [Google Scholar]
- Thermal analysis for obtaining coal ignition characteristics in boilers-Part 1: Specific heat capacity, ignition temperature, and ignition heat. Journal of China Coal Society 2024-07-23:1-11. https://doi.org/10.13225/j.cnki.jccs.2024.0146
- [Google Scholar]
- Experimental investigation of coal particle size on the kinetic properties of coal oxidation and spontaneous combustion limit parameters. Energy. 2023;270:126890. https://doi.org/10.1016/j.energy.2023.126890
- [CrossRef] [Google Scholar]
- Experimental assessment of multi-parameter index gas correlation and prediction system for coal spontaneous combustion. Combust. Flame. 2023;247:112485. http://dx.doi.org/10.1016/j.combustflame.2022.112485
- [Google Scholar]
- Thermal behavior and chemical reactivity of coal gangue during pyrolysis and combustion. Fuel. 2023;331:125927. https://doi.org/10.1016/j.fuel.2022.125927
- [CrossRef] [Google Scholar]
- Effect of soaking on coal oxidation activation energy and thermal effect. Journal of China Coal Society. 2018;43:2518-2524. http://dx.doi.org/10.13225/j.cnki.jccs.2017.1443
- [Google Scholar]
- Construction of macromolecular model and analysis of oxygen absorption characteristics of Hongyang No. 2 coal mine. Arab. J. Chem.. 2023;16:104662. http://dx.doi.org/10.1016/j.arabjc.2023.104662
- [CrossRef] [Google Scholar]
- Study on the molecular structure model of tar-rich coal and its pyrolysis process. Journal of Molecular Structure. 2023;1286:135613. https://doi.org/10.1016/j.molstruc.2023.135613
- [CrossRef] [Google Scholar]
- Experimental and molecular dynamics study into the surfactant effect upon coal wettability. RSC Advances. 2021;11:24543-24555. http://dx.doi.org/10.1039/D1RA01882E
- [CrossRef] [PubMed] [PubMed Central] [Google Scholar]
- Reactive force field (ReaxFF) molecular dynamics investigation of bituminous coal combustion under oxygen-deficient conditions. Fuel. 2022;318:123583. https://dx.doi.org/10.1016/j.fuel.2022.123583
- [CrossRef] [Google Scholar]
- Study on the influence of key active groups on gas products in spontaneous combustion of coal. Fuel. 2023;344:128020. https://dx.doi.org/10.2139/ssrn.4313235
- [CrossRef] [Google Scholar]