Translate this page into:
Synthesis and characterization of some tetrazoles and their prospective for aerobic micro-fouling mitigation
⁎Corresponding author. maherni@yahoo.com (Maher I. Nessim)
-
Received: ,
Accepted: ,
This article was originally published by Elsevier and was migrated to Scientific Scholar after the change of Publisher.
Peer review under responsibility of King Saud University.
Abstract
Two series of tetrazole derivatives of the type N-(1H-tetrazol-5-yl)-1-(aryl)methanimine (101–106) and 1-(4-alkoxyphenyl)-N-(1H-tetrazol-5-yl)methanimine (107–111) were synthesized and characterized via conventional tools of analysis (elemental analysis, FT-IR and 1H NMR spectroscopy). These two synthesized series were biologically evaluated for their potentials against some microbial biofilm causing strains (micro bio-foulants). Biological activities were evaluated by MIC values and cell viability percentages of them. In case of compounds (107–111), 107 was the most potent antimicrobial one, where its MIC values were 10.666667 µg/ml; 12.82222 µg/ml and 21.43666 µg/ml for Staphylococcus aureus, Escherichia coli and Candida albicans respectively, whereas compound 106, (of group 101–106), MIC values were 16 µg/ml for all the tested microorganisms. Viability assay showed that 107 activity percentages were 96.99456%, 92.32886% and 89.09558% against Gm +ve bacteria, Gm −ve bacteria and yeast respectively, whereas 106 activity percentages were 95.255569%, 90.204675% and 86.710956% against Gm +ve bacteria, Gm −ve bacteria and yeast respectively. Two antimicrobial mode of actions were proposed and discussed depending on the two evaluated tetrazole groups.
Keywords
Tetrazoles
Synthesis
Aerobic micro fouling
Antimicrobials
Staphylococcus aureus
Escherichia coli aureginosa
1 Introduction
Heterocyclic compounds are generally wide-known as multifunctional antimicrobial agents (Omardien et al., 2018). Tetrazoles are an important class of heterocyclic compounds which possess wide spectrum of biological properties (Kumar et al., 2014). They have attracted much attention because of their unique structure and applications as antihypertensive, antialergic, antibiotic and anticonvulsant agents (Myznikov et al., 2007; Schocken et al., 1989; Butter, 1984; Mavromoustakos et al., 1999; Mekni and Bakloiti, 2008; Toney et al., 1998; Tamura et al., 1998; Lim et al., 2007). They are a class of synthetic organic heterocyclic compound, consisting of a 5-member ring of four nitrogen and one carbon atom (Nessim et al., 2018). From literature survey, it was found that tetrazole derivatives possess very interesting pharmacological and biological properties and are reported to exhibit variety of biological activities like antibacterial, antifungal and anticonvulsant, analgesic, anti-inflammatory, anti-tubercular activity and anticancer activity (Kumar et al., 2014).
Similarly, 1,5 disubstituted tetrazoles have long been known for their pharmaceutical activity as stimulants or depressants on the central nervous system and are reported to show oral antidiabetic and antithrombotic and antimicrobial properties (Mohite et al., 2009; Modarresi and Nasrollahzadeh, 2009). The development of tetrazole derivatives has been largely associated with wide scale of applications of these classes of compounds in medicine, biochemistry agriculture and large number of medicinally important tetrazole heterocyclic incorporated drugs approved by FDA.
Tetrazoles and its derivatives have been reported to possess antinociceptive anti-inflammatory antimicrobial and anticonvulsant properties. Benzotriazole moiety is a versatile lead molecule in pharmaceutical development and more favorable pharmacokinetic profile, wide range of biological activities (Maria Dorathi Anu et al., 2013). Accordingly, synthesis of tetrazole derivatives is obviously an important task in modern medicinal chemistry (Varadaraji et al., 2010).
Facet-attached microbial communities are known as biofilm. Growth of these biofilms can promote micro-biofouling or microbial fouling (Di Pippo et al., 2018). Biofouling could be defined as “the process in which prokaryotic and eukaryotic organisms adhere to solid surfaces immersed in water”. Biofouling is wide-known to be responsible of different problems via extra fuel and maintenance costs in water- related industries. Microbial films and macrobial fauna as barnacles, mussels, and tunicates which accumulate on ships increase drag forces and surface corrosion, thereby causing biofouling dilemma (Crisp, 1974; Haderlie, 1974). Nowadays, biofouling is regarded as critical issue compromising environmental problems such as membrane water and wastewater treatment (Dadrasnia et al., 2017). Different microbial strains are involved in micro biofouling process such as Staphylococcus aureus (Parsek and Greenberg, 2005; Bixler and Bhushann, 2011); Escherichia coli (Pohl et al., 2015; Wood et al., 2016) and Candida albicans (Fernandes et al., 2016). In our work, two series of tetrazole derivatives were synthesized, characterized and evaluated as per their anti-microbial activity.
2 Experimental
2.1 Raw materials
All chemicals and solvents purchased from international chemical companies
-
Alkyl halides, tetrabutylammonium bromide and potassium hydroxide (Merck), benzaldehyde, p-hydroxybenzaldehyde, p-tolualdehyde, p-anisaldehyde, and 1H-tetrazol-5-amine (Sigma-Aldrich) and p-chlorobenzaldehyde and p-nitrobenzaldehyde (Fluka). They were of analytical grade and used without further purification.
-
Ethanol (absolute), benzene and tetrahydrofuran (Sigma-Aldrich).
2.2 Synthesis
2.2.1 Preparation of N-(1H-tetrazol-5-yl)-1-(aryl)methanimine (101–106)
A solution of 1H-tetrazol-5-amine (0.010 mol) and aromatic aldehydes (0.010 mol) (1–6) in ethanol was refluxed for 2 h in water bath Scheme 1. The resultant solution was cooled; the solid was filtered off and recrystallized from petroleum ether. Compounds 101–106 were obtained as crystalline mass (Kumar et al., 2014).Preparation of N-(1H-tetrazol-5-yl)-1-(aryl)methanimine (101–106). 101: 1-phenyl-N-(1H-tetrazol-5-yl)methanimine. 102: N-(1H-tetrazol-5-yl)-1-(p-tolyl)methanimine. 103: 4-((1H-tetrazol-5-yl)imino)methyl)phenol. 104: 1-(4-methoxyphenyl)-N-(1H-tetrazol-5-yl)methanimine. 105: 1-(4-chlorophenyl)-N-(1H-tetrazol-5-yl)methanimine. 106: 1-(4-nitrophenyl)-N-(1H-tetrazol-5-yl)methanimine.
2.2.2 Preparation of 1-(4-alkoxyphenyl)-N-(1H-tetrazol-5-yl)methanimine (107–111)
These five compounds were synthesized in two steps, Scheme 2:
-
Step 1: preparation of 4-alkoxybenzaldehyde:
-
As illustrated (Nessim et al., 2015), the five 4-alkoxybenzaldehydes were prepared via the reaction of 4-hydroxybenzaldehydes with five different alkyl bromides (C4H9Br, C6H13Br, C8H17Br, C10H21Br and C12H25Br).
-
Step 2: Synthesis of (107–111):
-
As discussed in Scheme 1 (Kumar et al., 2014), the five 4-alkoxybenzaldehydes reacted with 1H-tetrazol-5-amine to form compounds (107–111).
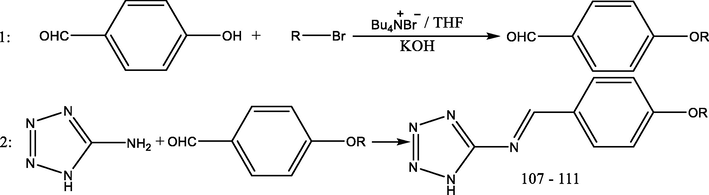
- Synthesis of compounds (107–111). 107: 1-(4-butoxyphenyl)-N-(1H-tetrazol-5-yl)methanimine. 108: 1-(4-hexylkoxyphenyl)-N-(1H-tetrazol-5-yl)methanimine. 109: 1-(4-octylkoxyphenyl)-N-(1H-tetrazol-5-yl)methanimine. 110: 1-(4-decylkoxyphenyl)-N-(1H-tetrazol-5-yl)methanimine. 111: 1-(4-dodecylkoxyphenyl)-N-(1H-tetrazol-5-yl)methanimine.
2.2.3 Preparation of stock standard solutions for biological assay
For each of the tested tetrazoles groups [(107–111) & (101–106)], stock standard solutions were freshly prepared and stored at 4 °C (Chemiasoft, 2011). Samples were dissolved in DMF that is widely known to have no antimicrobial activity against different microorganisms and is considered as negative control (Cooper, 1972).
2.2.4 Model Organisms
Identified bacterial isolates of Staphylococcus aureus, Escherichia coli and Candida albicans were provided from Cairo MIRCEN (MIRCEN).
2.2.5 Antimicrobial susceptibility test methods
-
Agar assay
Under sterilized conditions, for each of the synthesized tetrazole derivatives, agar-agar media (Balouiri et al., 2015) were freshly prepared and left till warmness and then seeded with 24 h grown bacterial suspensions (1–2 × 108 CFU/mL per McFarland standard), vortexed and poured onto Petri plates (4 mm height agar layer). Agar plates were then kept till dryness and stored at 4 °C for two hours. One millimeter wells were cork borered onto agar plates, inoculated with 100 µl of each of the test samples and incubated at 37 °C ± 2 for 48 h. Each experiment was done in triplicate (Parekh and Chanda, 2007). DMF was used as a negative control. Inhibition zones were measured, and activity indices were calculated according to Dut Jasuja et al. equation (Jasuja et al., 2014) [Eq. (1)].
Micro plate assay:
Recently, the 96-well Microtiter plate for MICs evaluation is commonly applied as time saver, a significant reducer of sample numbers experiment cost and place occupied, thus improving the repeatability of the results (Zhang et al., 2006). In a sterilized area, microbial suspensions were freshly cultured in suitable broth media (bacteria/yeast). About 0.125 μl of microbial cell suspension of 107 CFU/ml count were adjusted at 550 nm absorbance. Blank broth media were inoculated in the wells. Different sample concentrations were applied from 64 to 0.5 μg/ml via 10-fold serial dilutions. A volume of approximately 180 μl of a sample concentration is then injected to each well. The next step is to inoculate about 10 μl microbial suspension into each well. Some wells are specified to solvent, blank sample (without microbes), blank broth and untreated microbial suspensions. The 96-well micro plates were then shaker- incubated for 6–8 h at incubation temperature. Coloring agent (0.4% Resazurin) was applied to each of the 96 wells and the micro plates were incubated for two hours to accomplish dye reaction (Coffey and Anderson, 2014).
2.2.6 Cell cytotoxicity assay
Cell cytotoxicity assay is designed for screening the activity of a certain compound cellular effects such as cell proliferation or in the form of direct cytotoxic effects expressed by cell death. The metabolic activity of viable cells is commonly used as the basis for absorbance assay. Cell viable count is “a measure of the number of cells that are viable (i.e. alive and capable of growth) in a given area or volume”. One use of viable cell count is to estimate cytotoxicity. Continuous UV–Vis spectroscopic measurements of a treated microbial suspension optical density in correlation to a 100% live microbial could be employed to estimate viable cell numbers and percentage (Park et al., 2012; Mageswari and Subramanian, 2012). Microbial suspensions were prepared, adjusted to 0.5 O.D. McFarland standards (1–2 × 108 CFU/mL) and sterilized. For each test time interval, bacterial suspensions were centrifuged at 10,000 r.p.m. for 10 min (Sigma Centrifuge Model 3 K 300), and the supernatants were removed. A process of three subsequent washing s of the remaining pellets were carried out, suspended in sterilized deionized water and well-vortexed (Germany International Corp Vortex, Model VM-300). For each time interval, 24 mL of each test agent were injected and mixed with 6 mL of the bacterial suspension and their optical densities were measured (ATI Unicam 8625 UV/Vis Spectrophotometer). The 0-time untreated remaining bacterial suspensions were used as 100% live cells and optical density reading was measured and plate counted in CFU/mL. Eq. (2) (Park et al., 2012). Experiment was terminated after 48 hrs. of exposure.
3 Results and discussion
3.1 Characterization of synthesized compounds
3.1.1 Elemental analysis
Data obtained in Table 1 showed that the calculated percentages of the elements coincides with the observed ones.
Compound
C %
H %
N %
Cl
Melting Point °C
Ref.
Calc.
Obs.
Calc.
Obs.
Calc.
Obs.
Calc.
Obs.
101
55.48
55.21
4.07
4.16
40.44
40.58
–
–
210–12
Kumar et al. (2014)
102
57.74
57.52
4.85
4.95
37.41
37.52
–
–
236–39
Kumar et al. (2014)
103
50.79
50.71
3.73
3.84
37.02
36.98
–
–
130–33
104
53.20
53.22
4.46
4.53
34.47
34.36
–
–
225–27
105
46.28
46.18
2.91
2.96
33.73
33.81
17.07
17.05
256–56
Kumar et al. (2014)
106
65.34
65.26
7.44
7.56
27.21
27.18
–
–
248–50
107
62.70
62.81
7.37
7.28
24.37
24.25
–
–
246–49
108
64.73
64.80
7.99
7.86
22.20
22.25
–
–
240–42
109
66.44
66.39
8.51
8.58
20.39
20.36
–
–
232–35
110
67.89
67.77
8.95
9.01
18.85
18.90
–
–
228–31
111
69.14
69.21
9.33
9.29
17.53
17.49
–
–
220–22
3.1.2 Infra-red spectroscopy
See Table 2.
Cpd.
cm−1
NH
C⚌N
CHAromatic
CH Aliphatic
N⚌N
C—O—C
C⚌C
101
3394
1552
3087
2959
1451
–
1603
102
3386
1611
3199
2929
1449
–
1561
103
3327
1511
3195
2965
1451
–
1594
104
3383
1604
3199
2931
1451
–
1646
105
3383
1607
3199
2931
1450
–
1642
106
3254
1525
3071
2951
1525
–
1612
107
3391
1449
3200
2938
1449
1297
1654
108
3362
1511
3123
2952
1462
1263
1612
109
3389
1510
3125
2925
1466
1248
1605
110
3412
1510
3200
2926
1449
1258
1647
111
3413
1507
3203
2931
1454
1263
1645
3.1.3 Proton NMR spectroscopy of compounds (101–111)
101: C⚌CH at 9.38 singlet, 7.82 doublet, 7.55 doublet, 7.28 triplet and NH at 6.28 ppm singlet.
102: C⚌CH at 9.22 singlet, 7.68 doublet, 7.16 doublet, NH 6.32 singlet and CH3 Protons at 2.60 ppm singlet.
103: OH at 9.98, C⚌CH at 9.18 singlet, 7.68 doublet, 7.02 doublet and NH at 6.33 ppm singlet.
104: C⚌CH at 9.36 singlet, 7.95 doublet, 7.56 doublet, NH 6.36 singlet and OCH3 proton at 3.26 ppm singlet.
105: C⚌CH at 10.01 singlet, 7.95 doublet, 7.56 doublet and NH at 6.36 ppm singlet.
106: C⚌CH at 9.43 singlet, 8.40 doublet, 8.16 doublet and NH at 6.36 ppm singlet.
107: C⚌CH at 9.423 singlet, 7.826 doublet, 7.684 doublet, NH at 6.414 singlet, (e) CH2 at 4.328 triplet, CH2 Protons at 1.748 multiplet, CH3 protons at 0.848 triplet.
108: C⚌CH at 9.246 singlet, 7.825 doublet, 7.721 doublet, NH at 6.402 singlet, CH2 at 4.312 triplet, CH2 1.738 multiplet, and CH3 at 0.842 triplet.
109: C⚌CH at 9.864 singlet, 7.855 doublet, 7.113 doublet, NH at 6.441 singlet, CH2 at 4.063 triplet, CH2 at 1.728 multiplet, and CH3 at 0.857 triplet.
110: C⚌CH 9.285 singlet, 7.675 doublet, 7.482 doublet, NH at 6.421 singlet, CH2 at 4.214 triplet, CH2 at 1.728 multiplet and CH3 at 0.828 triplet.
111: C⚌CH at 9.114 singlet, 7.848 doublet, 7.763 doublet, NH at 6.460 singlet, CH2 at 4.062 triplet, CH2 at 1.715 multiplet and CH3 at 0.830 triplet.
3.2 Biological assays
3.2.1 Activity index
Screening the antibacterial activity of the synthesized tetrazoles, using diffusion techniques (Fig. 1A and B), revealed that they apparently exhibited antibacterial activities according to their main substituted groups together with the main skeleton activity. It is worth to mention that Gram-+ve bacteria showed more susceptibility towards the tested tetrazoles than Gram −ve bacteria and yeast. The composition of their microbial cell wall is considered to be a detrimental criterion influencing their response towards the introduced agents. Gram +ve bacterial cell wall main component is a thick layer of peptidoglycan, whereas peptidoglycan layer in Gram −ve bacteria are enclosed between an inner cytoplasmic layer and an outer membrane. In yeast, cell wall is made of polysaccharides, proteins, lipids and chitin that make its cell wall much thicker. So, yeast is more anti-microbially resistive than Gram −ve and Gram +ve bacteria respectively (Salton and Kim, 1996). For tetrazoles, (107–111), compound 107 revealed the highest activity index (Fig. 1A), whereas of the tetrazoles, (101–106), compound 106 was the most potent one (Fig. 1B).Activity index of the tested groups (A) & (B) against Staphylococcus aureus, Escherichia coli & Candida albicans. (Standard deviation ± 0.02).
3.2.2 Micro plate evaluation
Results of MIC (µg/ml) of the synthesized tetrazoles against Staphylococcus aureus were observed to be lower than Escherichia coli and Candida albicans respectively. Compound 107 seemed to be more toxic against tested microbes, where low concentration was needed to inhibit microbial growth (MIC value = 10.66666667 µg/ml) (Figs. 2 and 3A). While a minimum concentration of compound 106 (16 µg/ml) was fair enough to inhibit the growth of all the tested organisms (Figs. 2 and 3B).Micro plate MIC evaluation of Staphylococcus aureus, Escherichia coli & Candida albicans treated with tested tetrazoles.
MICs (µg/ml) of Staphylococcus aureus, Escherichia coli & Candida albicans treated with tested tetrazoles groups (A) & (B). (Standard deviation ± 0.02).
3.2.3 Cell viability and cytotoxicity assay
Cell Viability percentage can indirectly express sample activity and its cytotoxicity. Screening of cell viability (%) of Staphylococcus aureus, Escherichia coli and Candida albicans exposed to the two tetrazoles series, (101–106) and (107–111), are illustrated in Fig. 4A and B. Of the tetrazoles series (107–111) compound 107 showed highest activity, (average activity percentage = 93.282623%), whereas the highest average activity percentage one of series (101–106) belonged to compound 106 (90.723733%).Cell viability % of Staphylococcus aureus, Escherichia coli & Candida albicans treated with tested tetrazoles (A) & (B) after 48 h of exposure.
3.2.4 Antibacterial mode of action
Yet, a little is published discussing tetrazoles antimicrobial mechanism. Few suggestions were reported.
In the present work, the two evaluated tetrazoles series are suggested to have two logical modes of actions.
-
Considering series (107–111), alkyl chain length seems to control their antimicrobial mechanism. Precisely, the alkyl chain length affects their trend which may be attributed to molecules lipophilicity enhancing the attacking process into microbial cell membranes leading to deterioration of its transmembrane signaling leading cytotoxic effects and death. So as the chain length decrease the efficiency increase (Gao et al., 1999).
-
In series (101–106), another electrostatic mode of action is proposed. Efficacy increases with the positively charged tetrazole ring bearing electron-withdrawing group (Suzen et al., 2006). Then, electrostatic interaction with negatively charged microbial cell membranes forms reactive oxygen species. Reactive oxygen species are the by-products of an oxidative stress resulted from the electrostatic interaction.
-
Generally, antimicrobial action of an agent involves inhibition of enzymes involved in cell wall biosynthesis, nucleic acid (DNA) metabolism and protein synthesis responsible for membrane structure. Microbes have their protection mechanisms against external oxidative stresses (OS) expressed in altered enzymatic activities. The generally accepted aromatic tetrazoles mode of action entails inhibition of ergosterol in the microbial membrane. Recently, proposed mechanisms of antimicrobial mode of action depend mainly on the hypothesis of electron transfer, reactive oxygen species and oxidative stress that is considered as unifying process (Kovacic and Abadjian, 2017).
Tetrazoles act as free radical scavengers. For a certain extent, their antioxidant potential depends on substituted terminal groups and structure conjugation (Chand et al., 2018). Bacterial responses to tetrazoles oxidative stress are expressed as the generation of reactive oxygen species (ROS) leading to the oxidative damage to cellular proteins. In addition, utilization of electron transfer processes (ET) could also be responsible of different physiological alterations (Kovacic and Abadjian, 2017; Kashmiri and Mankar, 2014; Kovacic and Somanathan, 2010; McDonnell and Denver Russell, 1999).
4 Conclusion
-
Tetrazoles of the two tested series [(107–111) and (101–106)] were in vitro synthesized and evaluated for their anti-micro biofouling activity.
-
Compound 107 of the tested group, (107–111), showed the highest efficacy. It possessed 96.99456%, 92.32886% and 89.09558% antimicrobial activity percentages against Gm +ve bacteria, Gm −ve bacteria and yeast respectively.
-
On the other hand, compound 106 of group, (101–106), was the most potent tetrazole. Its antimicrobial activity percentages were 95.25557% against Gram positive bacteria, 90.20468% against Gram negative bacteria and 86.71096% against tested yeast. It is worth to mention that all the tested tetrazoles proved their promising anti-micro biofouling activity.
-
For series (107–111), average activity percentage was in the range between 88.56631% and 93.282623%, whereas in series (101–106), ranged between 75.895098% and 90.723733%.
-
Their modes of actions suggestions were articulated on two mechanisms. One depends on alkyl chain length (107–111) while the other (101–106) is an electrostatic mechanism. The type of mechanism is related to the nature of the tested tetrazoles.
-
The hypothesis of ET-ROS-OS theory is a subsequent process of the two proposed mechanisms expressing microbial inhibition and cytotoxicity.
Declaration of Competing Interest
The authors declare that they have no known competing financial interests or personal relationships that could have appeared to influence the work reported in this paper.
References
- Methods for in vitro evaluating antimicrobial activity: A review. J. Pharm. Anal.. 2015;6:71-79.
- [Google Scholar]
- Biofouling: lessons from nature. Review. Philos. Trans. R. Soc.. 2011;A 370:2381-2417.
- [Google Scholar]
- Butter, R.N., 1984. Comprehensive heterocyclic chemistry. In: Katritzky, A.R., Rees, C.W. (Eds.), vol. 5: Part 4A, pp. 791–838.
- Synthesis and antimicrobial and antioxidant activities of hybrid molecules containing benzotriazole and 1,2,4-triazole. Turk. J. Chem.. 2018;42:1663-1677.
- [Google Scholar]
- Chemiasoft, 2011. In: “Guide to Preparation of Stock Standard Solutions, First edition. ©Chemiasoft; David Harvey (2000). In ”Modern Analytical Chemistry“. © The McGraw-Hill Companies, Inc. for manufacture and export.
- Biofilm formation in the 96-well Microtiter plate. Methods Mol Biol.. 2014;1149:631-641.
- [Google Scholar]
- Cooper, R.E., 1972. In: Kavanageh, F.W. (Ed.), Analytical Microbiology, Vol. 1&II, Academic Press, New York and London.
- Crisp, D.J., 1974. Factors influencing the settlement of marine invertebrate larvae. In: Grant, P.T., Mackie, A.M. (Eds.), Chemoreception in marine organisms. Academic Press, Inc., New York, pp. 177–265.
- Microbial aspects in wastewater treatment – A technical review. Environ. Pollut. Protect.. 2017;2(2):75-84.
- [Google Scholar]
- Biofilm growth and control in cooling water industrial systems. FEMS Microbiol. Ecol.. 2018;94:fiy044.
- [Google Scholar]
- Biofilm formation by Candida albicans and Streptococcus mutants in the presence of farnesol: a quantitative evaluation. J. Bioadhes. Biofilm Res.. 2016;32(3):329-338.
- [Google Scholar]
- The outer membrane of Gram-negative bacteria inhibits antibacterial activity of brochocin-C. Appl. Environ. Microbiol.. 1999;65:4329-4333.
- [Google Scholar]
- Growth rates, depth preference, and ecological succession of some sessile marine invertebrates in Monterey Harbor. Veliger. 1974;17(suppl.):1-35.
- [Google Scholar]
- Green synthesis of AgNPs stabilized with bio waste and their antimicrobial activities. Braz. J. Microbiol.. 2014;45(4):1325-1332.
- [Google Scholar]
- “Free radicals and oxidative stress in bacteria. Int. J. Curr. Microbiol. Appl. Sci.. 2014;3(9):34-40.
- [Google Scholar]
- Mechanism of antifungal triazoles and related drugs: electron transfer, reactive oxygen species and oxidative stress. SOJ Microbiol. Infect. Dis. 2017 (Symbiosis Online Publishing)
- [Google Scholar]
- Mechanism of antifungal triazoles and related drugs: electron transfer, reactive oxygen species and oxidative stress. Microbiol. Infect. Dis.. 2017;5(5):1-9.
- [Google Scholar]
- Mechanism of conjugated imine and iminium species, including marine alkaloids: electron transfer, reactive oxygen species, therapeutics and toxicity. Curr Bioact. Compd.. 2010;6(1):46-59.
- [Google Scholar]
- Synthesis, Characterization and biological evaluation of tetrazole derivatives. Indo Am. J. Pharm. Res.. 2014;4(11)
- [Google Scholar]
- Action of fentrazamide on protein metabolism and cell division in plants. J. Pestic. Sci.. 2007;32:249-254.
- [Google Scholar]
- Synthesis, characterization, and study of antibacterial activity of homo polymers and copolymers of 4-benzyloxyphenylacrylates for pressure-sensitive adhesive application. J. Appl. Polym. Sci.. 2012;125:3115-3124.
- [Google Scholar]
- Synthesis, characterization, antibacterial & anti-inflammatory effects of substituted tetrazole derivatives based on different types of carbazone and benzaldehyde. Int. J. ChemTech Res. CODEN (USA). 2013;5(4):1982-1990.
- [Google Scholar]
- An effort to understand the molecular basis of hypertension through the study of conformational analysis of losartan and sarmesin using a combination of nuclear magnetic resonance spectroscopy and theoretical calculations. J. Med. Chem.. 1999;42:1714-1722.
- [Google Scholar]
- Antiseptics and disinfectants: Activity, action, and resistance. Clin. Microbiol. Rev. 1999:147-179. 0893-8512/99/$04.0010
- [Google Scholar]
- Synthesis of new 1-substituted 4-perfluoroalkyl tetrazol-5-ones. J. Fluorine Chem.. 2008;129:1073-1075.
- [Google Scholar]
- Synthesis of 5-arylamino-1H(2H)-tetrazoles and 5-amino-1-aryl-1H-tetrazoles from secondary arylcyanamides in glacial acetic acid: A simple and efficient method. Turk. J. Chem.. 2009;33:267-280.
- [Google Scholar]
- A novel approach for synthesis of substituted tetrazoles. Digest J. Nanomater. Biostruct.. 2009;4(4):803-807.
- [Google Scholar]
- MRC Stands for Microbiological Resources Centre, Faculty of Agriculture, Ain Shams University, Cairo, Egypt.
- The role of 5-(4-alkoxybenzylidine)-2-thioxo-4-oxo-1,3-thiazolidine as antioxidants for Egyptian lube oil base stock. Int. J. Curr. Res. Chem. Pharma. Sci.. 2015;2(2):80-93.
- [Google Scholar]
- Synthesis and study antimicrobial activities of some novel tetrazole derivatives. Egypt. J. Chem.. 2018;61(1)
- [Google Scholar]
- Evaluating novel synthetic compounds active against Bacillus subtilis and Bacillus cereus spores using live imaging with Spore Tracker X. Sci. Rep. 2018:8. (Article No: 9128)
- [Google Scholar]
- Antibacterial and phytochemical studies on twelve species of Indian medicinal plants. Afr. J. Biomed. Res.. 2007;10:175-181.
- [Google Scholar]
- Development of rapid assessment method to determine bacterial viability based on ultraviolet and visible (UV-Vis) spectroscopy analysis including application to bioaerosols. Aerosol. Air Qual. Res.. 2012;12:399-408.
- [Google Scholar]
- Sociomicrobiology: the connections between quorum sensing and biofilms. Trends Microbiol.. 2005;13:27-33.
- [Google Scholar]
- Biofouling on polymeric heat exchanger surfaces with E. coli and native biofilms. J. Bioadhes. Biofilm Res.. 2015;31(9–10):699-707.
- [Google Scholar]
- Salton, Milton R.J., Kim, Kwang-Shin, 1996. Chapter 2: structure. In: Baron, S. (Ed.), Medical Microbiology, fourth ed. (ISBN-10: 0-9631172-1-1).
- Microbial transformation of the tetrazolinone herbicide F5231. Appl. Environ. Micobiol.. 1989;55:1220-1222.
- [Google Scholar]
- Investigation of in vitro antioxidant behavior of some 2-phenylindole derivatives: discussion on possible antioxidant mechanisms and comparison with melatonin. J. Enzyme Inh. Med. Chem.. 2006;21(4):405-411.
- [Google Scholar]
- Highly selective and orally active inhibitors type IV collagenase (MMP-9 and MMP-2): N-sulfonylamino acid derivatives. J. Med. Chem.. 1998;41:640-649.
- [Google Scholar]
- Antibiotic sensitization using biphenyl tetrazoles as potent inhibitors of bacteroides fragilis metallo-b-lactamase. Chem. Biol.. 1998;5:185-196.
- [Google Scholar]
- Synthesis and evaluation of a series of 1-substituted tetrazole derivatives as antimicrobial agents. Org. Commun.. 2010;3(3):45-56.
- [Google Scholar]
- Living biofouling-resistant membranes as a model for the beneficial use of engineered biofilms. Proc. Natl. Acad. Sci. U. S. A.. 2016;17113(20):E2802-E2811.
- [Google Scholar]
- A simple 96-well micro plate method for estimation of total polyphenol content in seaweeds. J. Appl. Phycol.. 2006;18:445-450.
- [Google Scholar]