Translate this page into:
Treatment of rheumatoid arthritis with dissymmetric fluorine and pyridyl-substituted 3,5-bis(aryl)-4-piperidone derivatives by inhibition of NF-κB and MAPK activation
⁎Corresponding author. gengzikai@126.com (Zi-Kai Geng)
⁎⁎Corresponding author. maliyingbz@163.com (Li-Ying Ma),
⁎⁎⁎Corresponding author. qinggmeng@163.com (Qing-Guo Meng),
-
Received: ,
Accepted: ,
This article was originally published by Elsevier and was migrated to Scientific Scholar after the change of Publisher.
Abstract
Abstract
Inhibition of inflammation-related NF-κB and MAPK activation could treat rheumatoid arthritis (RA). Previously, some fluorine-substituted 3,5-bis(aryl)-4-piperidone derivatives (BAPs) exhibited promising anti-inflammatory activity. Herein, interesting structural optimization was carried out and generated thirty-eight dissymmetric fluorine and pyridyl-substituted BAPs (4a-s and 5a-s). They were confirmed by NMR and HRMS. A preliminary structure–activity relationship was summarized and discovered 3,5-CF3 and 3-pyridyl-substituted 4k exhibited less toxicity and higher anti-inflammatory activity. Preliminary mechanistic studies revealed that 4k inhibited Lipopolysaccharide-induced nuclear factor kappa-B (NF-κB) and mitogen-activated protein kinase (MAPK) activation by suppressing LPS-induced phosphorylation levels of NF-κB/p65, NF-κB/IκBα, c-Jun N-terminal kinase (JNK), extracellular regulated protein kinases (ERK), and NF-κB/p38 in RAW264.7 cells. In vivo, 4k inhibited the development of inflammation and synovial proliferation in rat joint tissues, significantly decreasing paw swelling and arthritis index in adjuvant-induced arthritis rats. More importantly, 4k did not affect body weight and splenic index values. Overall, 4k showed optimistic anti-RA effects, which may be developed as a potential multifunctional agent for the clinical treatment of RA.
Keywords
Rheumatoid arthritis (RA)
3,5-Bis(arylidene)-4-piperidone derivatives (BAPs)
Anti-inflammation
NF-κB
MAPK
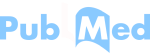
- AIA
-
adjuvant-induced arthritis
- BAPs
-
3,5-bis(arylidene)-4-piperidones
- BAP 4k BAP
-
representative compound 4k
- CCK-8
-
Cell Counting Kit-8
- CFA
-
complete Freund’s adjuvant
- ERK
-
extracellular regulated protein kinases
- IκB
-
inhibitory subunit of nuclear factor-Kappa B
- JNK
-
c-Jun N-terminal kinase
- LPS
-
Lipopolysaccharide
- MAPK
-
mitogen-activated protein kinase
- MTX
-
methotrexate
- NF-κB
-
nuclear factor kappa-B
- RA
-
rheumatoid arthritis
- PDTC
-
Pyrrolidine dithiocarbamate
- TNF-α
-
tumor necrosis factor-α
- IL-6
-
interleukin-6
Abbreviations
1 Introduction
Rheumatoid arthritis (RA) is an autoimmune disease characterized by synovial cell hyperplasia, joint swelling, and joint pain (Bottini and Firestein, 2013; Nugroho and Morita, 2014; Wang et al., 2019). The clinical symptoms of RA are mainly inflammatory lesions in multiple joints throughout the body, characterized by chronic, peripheral, and symmetrical nature in its onset (Littlejohn and Monrad, 2018). As the disease progresses, RA affects multiple other organs in other parts of the body such as the heart, lungs, and kidneys. Both nuclear factor-κB (NF-κB) and mitogen-activated protein kinase (MAPK) signaling pathways are classical inflammatory pathways that promote the proliferation and differentiation of osteoclasts, cause bone erosion and bone destruction, with important roles in the progression of RA (Wei et al., 2022). In studies of synovial cells from RA patients, inhibition of NF-κB pathway activation inhibited the development of arthritis and pathological damage to the joints. The MAPK pathway is divided into three components: Extracellular signal-regulated kinase (ERK), c-Jun N-terminal kinase (JNK), and p38 MAPK. JNK and p38 are activated mainly by stress stimuli or inflammatory cytokines. They can both influence the development of inflammation (Behl et al., 2021; Chen et al., 2023). Elevated expression of NF-κB in synovial tissue of RA patients is associated with overexpression of inflammatory mediators such as TNF-α and IL-6. It has been shown that p38 can regulate the production of cytokines such as TNF-α by macrophages, while JNK inhibitors can effectively reduce bone destruction in adjuvant arthritic rats. The pathogenesis of RA involves chronic inflammation, and various inflammatory stimuli (such as bacterial endotoxin lipopolysaccharide (LPS) and other foreign antigens). Inflammatory stimuli can induce macrophages to migrate to the contact site to produce TNF-α, IL-6 and promote inflammation, so studies using macrophage activation can reflect the inflammatory process in RA.
Curcumin (Fig. 1) is a natural component with anti-inflammatory and anti-cancer activities, including anti-RA (Pourhabibi-Zarandi et al., 2021), but its clinical application is limited by its structural instability, low bioavailability, and false positives. Therefore, the optimization of curcumin analogs has received extensive attention based on the pharmacodynamic structure of curcumin and literature reports. Among them, (3E,5E)-3,5-bis(arylidene)-4-piperidone (BAP) is a typical example, which has good anti-inflammatory and antitumor activities by inhibiting the activation of NF-κB. For example, fluorine-substituted 3,5-bis(2-fluorobenzylidene)-4-piperidone (EF24, Fig. 1) can inhibit tumor growth and metastasis by inhibiting NF-κB signaling pathways (He et al., 2018). An alkylated mono-carbonyl analog of curcumin (MAC 7a, Fig. 1) showed potent anti-inflammatory activities against LPS-induced acute lung injury (ALI) in rats by inhibiting the expression of IL-6, IL-1β, TNF-α, and VCAM-1 in Beas-2B cells (Zhu et al., 2016). In recent years, our group obtained a number of BAPs and evaluated their anti-inflammatory activity, as well as toxicity. We found that some BAPs (e.g. BAP 6d, BAP 32, BAP 67, Fig. 1) also showed good inhibition of MAPK signaling pathway, or were associated with NF-κB signaling pathway (Li et al., 2018a, 2018b; Yao et al., 2019). Structural analysis shows that these active compounds all contain different substituents, such as pyridyl, fluorophenyl, and trifluoromethylphenyl groups on both sides of 4-piperidone. On the one hand, the dissymmetric molecular structure may be more conducive to the interaction between lead compounds and targeted biological macromolecules. On the other hand, it is expected that the optimization of the different substituents will further optimize the water-solubility, toxicity, and bioactivity (Gao et al., 2020; Li et al., 2018a, 2018b). Therefore, the development of novel dissymmetric BAPs with different substituents for anti-inflammatory activity drug screening is of great research value.The structures of curcumin and some BAPs were reported.
Literature reports show that fluorine substitution can influence the potency, conformation, metabolism, membrane permeability, P-gp recognition of a molecule, and temper inhibition of the hERG channel by basic amines (Meanwell, 2018). Meanwhile, the electronegative fluorine substitution can form multiple H-bonds with the target protein (Swallow, 2015). In addition, pyridine substituent, as one of the imperative pharmacophores occurring synthetically as well as naturally in heterocyclic compounds, showed a wide range of therapeutic applications in the area of drug discovery, and many pyridine-based derivatives are reported to inhibit enzymes, receptors, and many other targets for controlling and curing the global serious diseases (Al-Warhi et al., 2023). Previously, our group reported that a group of fluorine or pyridyl-substituted BAPs derivatives could reasonably bind to the active site of Bcl-2, p65, and p38 proteins through multiple hydrogen bonds and halogen (fluorine) bonds (Sun et al., 2020; Sun et al., 2019; Cong et al., 2021). On this basis, our interests lie in the incorporation of different substituents (e.g. 3- or 4-pyridyl substituents, -F or -CF3 substituents) on both sides of BAPs to report some dissymmetric BAPs. The desired and improved anti-inflammatory activity of BAPs for the treatment of RA could be found. According to the above reasons, a series of dissymmetric fluorine and pyridyl-substituted BAPs were synthesized and evaluated as potential anti-inflammatory agents for the treatment of RA by inhibition of MAPK and NF-κB signaling pathways in this study. It is hoped that our study will yield potentially versatile drugs for the treatment of inflammatory diseases such as RA.
2 Experimental
2.1 Materials and methods
Chemical reagents such as fluorobenzene, pyridine benzene, all fragrant aldehydes, and piperidones had been then procured from Leyan Technology Co., Ltd(Beijing, China) and Bide Pharmaceutical Technology Co., Ltd. (Shanghai, China). Referring to the literature synthesis methods (Li et al., 2018a, 2018b), BAP derivatives (4a-s, 5a-s) have been synthesized. Spectral records had been accumulated through the usage of 1H NMR, Bruker Avance four hundred MHz or 600 MHz spectrometer and 13C NMR, Bruker Avance one hundred MHz or a hundred and fifty MHz spectrometer in dimethyl sulfoxide (DMSO‑d6) solvent with tetramethylsilane (TMS) as inner standard. Chemical shifts (δ) are given in ppm and coupling constants (J) are given in Hz. All melting factors had been measured on a digital melting factor meter barring correction.
2.2 General procedure for the preparation of asymmetric BAP derivatives 4a-s
To 5 mL of methanol was once introduced 3-pyridinobenzaldehyde (0.54 g, 5.00 mmol), N-methylpiperidone (0.57 g, 5.00 mmol), and 3-fluorobenzaldehyde (0.62 g, 5.00 mmol). 2 mL of 10 % NaOH answer was brought at 25 °C and stirred for 6.0 h, all through which the response was observed via TLC. After the reaction, the precipitate was withdrawn and filtered, and the used to be subjected to silica gel column chromatography (petroleum ether: ethyl acetate: methanol = 10: 10: 1) to reap compound 4a as a yellow solid. Compound 4b-s was prepared in accordance with the instruction approach of compound 4a.
2.2.1 (E)-3-((E)-3-fluorobenzylidene)-1-methyl-5-(pyridin-3-ylmethylene)piperidin-4-one (4a)
Yellow powder; yield: 67 %; Mp:136.3 ∼ 137.6 °C; 1H NMR (600 MHz, DMSO‑d6) δ 8.72 (d, J = 2.3 Hz, 1H), 8.59 (dd, J = 4.9, 1.6 Hz, 1H), 7.92 (dt, J = 8.0, 2.0 Hz, 1H), 7.60 (dd, J = 7.3 Hz, 2H), 7.52 (dt, J = 13.5, 7.8 Hz, 2H), 7.35 (t, J = 7.9 Hz, 2H), 7.30–7.24 (td, J = 8.6, 2.6 Hz, 1H), 3.75 (s, 2H), 3.75 (s, 2H), 2.40 (s, 3H). 13C NMR (100 MHz, DMSO‑d6) δ 183.31, 162.09 (d, J = 243.3 Hz), 151.17, 149.66, 137.18, 136.89 (d, J = 8.5 Hz), 135.43, 134.69, 133.53 (d, J = 2.4 Hz), 131.37, 130.71 (d, J = 8.6 Hz), 130.53, 126.60, 123.73, 116.84 (d, J = 22.2 Hz), 116.09 (d, J = 22.2 Hz), 56.15, 46.58. HRMS (ESI): m/z calcd. for C19H17FN2O, [M + H]+, 309.1398; found: 309.1400.
2.2.2 (E)-3-((E)-4-fluorobenzylidene)-1-methyl-5-(pyridin-3-ylmethylene)piperidin-4-one (4b)
Yellow powder; yield: 68 %; Mp:135.2 ∼ 136.8 °C; 1H NMR (600 MHz, DMSO‑d6) δ 8.72 (s, 1H), 8.59 (d, J = 4.8 Hz, 1H), 7.92 (dt, J = 8.2, 2.2 Hz, 1H), 7.63–7.56 (m, 4H), 7.50 (dd, J = 7.9, 4.8 Hz, 1H), 7.32 (t, J = 8.8 Hz, 2H), 3.74 (s, 2H), 3.74 (s, 2H), 2.40 (s, 3H). 13C NMR (150 MHz, DMSO‑d6) δ 186.70, 162.88(d, J = 247.5 Hz), 151.59, 150.06, 137.61, 135.98, 134.34, 133.74 (d, J = 2.5 Hz), 133.38 (d, J = 8.5 Hz), 131.60, 131.04, 124.18, 116.28 (d, J = 21.1 Hz), 56.74, 56.61, 45.72. HRMS (ESI): m/z calcd. for C19H17FN2O, [M + H]+, 309.1398; found: 309.1398.
2.2.3 (E)-1-methyl-3-(pyridin-3-ylmethylene)-5-((E)-3-(trifluoromethyl)benzylidene)piperidin-4-one (4c)
Yellow powder; yield: 55 %; Mp:129.7 ∼ 131.5 °C; 1H NMR (600 MHz, DMSO‑d6) δ 8.74 (d, J = 1.8 Hz, 1H), 8.60 (dd, J = 4.7, 1.2 Hz, 1H), 7.94 (d, J = 8.0 Hz, 1H), 7.67 (t, J = 7.3 Hz, 2H), 7.61–7.49 (m, 2H), 7.46 (s, 1H), 7.29–7.19 (m, 2H), 3.78 (s, 2H), 3.36 (s, 2H), 2.32 (s, 3H). 13C NMR (150 MHz, DMSO‑d6) δ 186.75, 151.66, 150.15, 137.66, 136.09, 135.85, 135.61, 134.27, 133.73, 132.01, 129.99 (q, J = 31.5 Hz), 127.44 (q, J = 4.5 Hz), 126.05 (q, J = 4.0 Hz), 124.52(d, J = 271.5 Hz), 124.21, 56.53, 56.53, 45.60. HRMS (ESI): m/z calcd. for C20H17F4N2O, [M + H]+, 377.1272; found: 377.1284.
2.2.4 (E)-3-((E)-2,3-difluorobenzylidene)-1-methyl-5-(pyridin-3-ylmethylene)piperidin-4-one (4d)
Yellow powder; yield: 70 %; Mp:134.6 ∼ 136.2 °C; 1H NMR (600 MHz, DMSO‑d6) δ 8.73 (d, J = 2.3 Hz, 1H), 8.60 (dd, J = 4.8, 1.6 Hz, 1H), 7.93 (dt, J = 7.9, 2.0 Hz, 1H), 7.64 (t, J = 2.2, 2H), 7.56–7.47 (m, 2H), 7.36–7.26 (m, 2H), 3.77 (s, 2H), 3.64 (s, 2H), 2.37 (s, 3H). 13C NMR (150 MHz, DMSO‑d6) δ 186.34, 151.70, 150.31 (dd, J = 244.5, 12.0 Hz), 150.22, 148.48 (dd, J = 250.0, 12.8 Hz), 137.71, 136.89, 135.62, 132.57, 130.92, 126.58, 126.00, 125.52 (d, J = 9.9 Hz), 125.04 (d, J = 9.9 Hz), 124.20, 118.81 (d, J = 16.8 Hz), 56.72, 56.33, 45.56. HRMS (ESI): m/z calcd. for C19H17F2N2O, [M + H]+, 327.1304; found: 377.1305.
2.2.5 (E)-3-((E)-3,4-difluorobenzylidene)-1-methyl-5-(pyridin-3-ylmethylene)piperidin-4-one (4e)
Yellow powder; yield: 59 %; Mp:127.2 ∼ 128.9 °C; 1H NMR (600 MHz, DMSO‑d6) δ 8.71 (d, J = 2.3 Hz, 1H), 8.59 (dd, J = 4.8, 1.5 Hz, 1H), 7.91 (dt, J = 8.1, 2.2 Hz, 1H), 7.66–7.58 (m, 2H), 7.58–7.47 (m, 3H), 7.38 (m, 1H), 3.76 (s, 2H), 3.71(s, 2H), 2.40 (s, 3H). 13C NMR (150 MHz, DMSO‑d6) δ 186.70, 151.64, 150.21(dd, J = 249.0 Hz, 12 Hz), 149.79 (dd, J = 244.5 Hz, 12 Hz), 137.64 (d, J = 9.9 Hz), 135.86, 134.91, 133.21, 132.71 (d, J = 9.9 Hz), 131.85, 128.28, 124.19, 119.82 (d, J = 17.4 Hz), 118.35 (d, J = 17.4 Hz), 56.60, 56.48, 45.67. HRMS (ESI): m/z calcd. for C19H17F2N2O, [M + H]+, 327.1304; found: 377.1303.
2.2.6 (E)-3-((E)-2,4-difluorobenzylidene)-1-methyl-5-(pyridin-3-ylmethylene)piperidin-4-one (4f)
Yellow powder; yield: 56 %; Mp:147.0 ∼ 149.0 °C; 1H NMR (600 MHz, DMSO‑d6) δ 8.62 (d, J = 2.3 Hz, 1H), 8.49 (dd, J = 4.8, 1.6 Hz, 1H), 7.82 (dt, J = 8.0, 2.0 Hz, 1H), 7.52 (d, J = 8.7 Hz, 2H), 7.44 (td, J = 8.8, 6.5 Hz, 1H),7.40 (dd, J = 7.8, 4.9 Hz, 1H),7.30 (ddd, J = 10.9, 9.3, 2.6 Hz, 1H), 7.11 (td, J = 9.8, 2.6 Hz,1H), 3.65 (s, 2H), 3.65 (s, 2H), 2.40 (s, 3H). 13C NMR (150 MHz, DMSO‑d6) δ 186.36, 162.26 (dd, J = 249.0, 12.5 Hz), 161.22 (dd, J = 250.5, 12.4 Hz), 151.67, 150.18, 137.68, 135. 70, 135.66, 132.76 (d, J = 9.9 Hz), 132.34, 130.97, 126.33, 124.20, 119.41 (d, J = 21.9, 3.5 Hz), 112.52 (d, J = 21.9, 3.5 Hz), 105.01 (t, J = 26.4 Hz), 56.72, 56.40, 45.60. HRMS (ESI): m/z calcd. for C19H17F2N2O, [M + H]+, 327.1304; found: 377.1304.
2.2.7 (E)-3-((E)-2,5-difluorobenzylidene)-1-methyl-5-(pyridin-3-ylmethylene)piperidin-4-one (4g)
Yellow powder; yield: 42 %; Mp:156.1 ∼ 157.2 °C; 1H NMR (600 MHz, DMSO‑d6) δ 8.73 (d, J = 2.1 Hz, 1H), 8.60 (dd, J = 4.8, 1.5 Hz, 1H), 7.93 (dt, J = 8.0, 2.0 Hz, 1H), 7.64 (s, 1H), 7.59 (s, 1H), 7.51 (dd, J = 7.9, 4.8 Hz, 1H), 7.43–7.31 (m, 3H), 3.77 (s, 2H), 3.66 (s, 2H), 2.38 (s, 3H). 13C NMR (150 MHz, DMSO‑d6) δ 186.39, 158.32(d, J = 240 Hz), 157.02 (d, J = 244.02 Hz), 151.70, 150.22, 137.70, 136.87, 135.62, 132.52, 130.91, 126.19, 124.20, 124.10 (dd, J = 24.9, 8.6 Hz), 118.33 (dd, J = 24.2, 9.1 Hz), 117.80 (dd, J = 25.1, 9.2 Hz), 117.45 (d, J = 24.9 Hz), 56.72, 56.13, 45.55. HRMS (ESI): m/z calcd. for C19H17F2N2O, [M + H]+, 327.1304; found: 377.1308.
2.2.8 (E)-3-((E)-2,6-difluorobenzylidene)-1-methyl-5-(pyridin-3-ylmethylene)piperidin-4-one (4h)
Yellow powder; yield: 33 %; Mp:136.4 ∼ 138.0 °C; 1H NMR (600 MHz, DMSO‑d6) δ 8.74 (d, J = 1.8 Hz, 1H), 8.60 (dd, J = 4.7, 1.2 Hz, 1H), 7.94 (d, J = 8.0 Hz, 1H), 7.67 (s, 1H), 7.61–7.49 (m, 2H), 7.46 (s, 1H), 7.24 (t, J = 8.2 Hz, 2H), 3.78 (s, 2H), 3.34 (s, 2H), 2.32 (s, 3H). 13C NMR (150 MHz, DMSO‑d6) δ 185.74, 160.08 (d, J = 247.5 Hz), 160.04 (d, J = 247.5 Hz), 155.07, 151.92, 151.73, 150.27, 137.91, 136.51, 135.34, 133.20, 132.34 (t, J = 9.9 Hz), 130.90, 124.84, 124.20, 122.39, 112.51 (dd, J = 21.1 Hz, 4.7 Hz), 112.15 (t, J = 26.4 Hz). 56.90, 56.32, 45.40. HRMS (ESI): m/z calcd. for C19H17F2N2O, [M + H]+, 327.1304; found: 377.1307.
2.2.9 (E)-3-((E)-3,5-difluorobenzylidene)-1-methyl-5-(pyridin-3-ylmethylene)piperidin-4-one (4i)
Yellow powder; yield: 72 %; Mp:145.5 ∼ 147.1 °C; 1H NMR (600 MHz, DMSO‑d6) δ 8.73 (d, J = 2.2 Hz, 1H), 8.60 (dd, J = 4.8, 1.6 Hz, 1H), 7.93 (d, J = 8.0 Hz, 1H), 7.62 (s, 1H), 7.56 (s, 1H), 7.51 (dd, J = 8.0, 4.7 Hz, 1H), 7.33 (tt, J = 9.4, 2.4 Hz, 1H), 7.26 (d, J = 6.4 Hz, 2H), 3.76 (s, 2H), 3.76 (s, 2H), 2.40 (s, 3H). 13C NMR (150 MHz, DMSO‑d6) δ 186.76, 162.76 (d, J = 245.1, 13.6 Hz), 151.67, 150.17, 138.44 (t, J = 9.8 Hz), 137.66, 136.22, 135.79, 132.82, 132.06, 130.92, 124.19, 113.77 (d, J = 25.8 Hz), 105.06 (t, J = 25.8 Hz), 56.59, 56.35, 45.61. HRMS (ESI): m/z calcd. for C19H17F2N2O, [M + H]+, 327.1304; found: 377.1307.
2.2.10 (E)-3-((E)-2,4-bis(trifluoromethyl)benzylidene)-1-methyl-5-(pyridin-3-ylmethylene)piperidin-4-one (4j)
Yellow powder; yield: 45 %; Mp:158.2.5 ∼ 159.7 °C; 1H NMR (600 MHz, DMSO‑d6) δ 8.74 (d, J = 2.2 Hz, 1H), 8.60 (dd, J = 4.8 Hz, 1.6 Hz, 1H), 8.18–8.10 (m, 2H), 7.94 (dt, J = 8.1 Hz, 2.0 Hz, 1H), 7.80–7.73 (m, 2H), 7.67 (d, J = 2.2 Hz, 1H), 7.51 (dd, J = 8.1 Hz, 4.8 Hz, 1H), 3.77 (s, 2H), 3.54 (s, 2H), 2.32 (s, 3H). 13C NMR (150 MHz, DMSO‑d6) δ 186.18, 151.76, 150.33, 137.77, 135.35, 133.24, 132.65, 130.87, 130.14 (d, J = 4.5 Hz), 129.75 (q, J = 33.0 Hz), 129.12 (q, J = 30.0 Hz), 123.76 (q, J = 270.0 Hz), 123.67 (q, J = 271.5 Hz), 129.86, 129.64, 129.12 (dd, J = 61.3, 30.3 Hz), 124.23, 123.65 (q, J = 6 Hz), 56.72, 55.58, 45.35. HRMS (ESI): m/z calcd. for C21H17F6N2O, [M + H]+, 427.1240; found: 427.1247.
2.2.11 (E)-3-((E)-3,5-bis(trifluoromethyl)benzylidene)-1-methyl-5-(pyridin-3-ylmethylene)piperidin-4-one (4k)
Yellow powder; yield: 36 %; Mp:133.0 ∼ 134.7 °C; 1H NMR (600 MHz, DMSO‑d6) δ 8.74 (d, J = 2.3 Hz, 1H), 8.60 (dd, J = 4.8, 1.6 Hz, 1H), 8.15 (d, J = 7.9 Hz, 3H), 7.94 (dt, J = 8.1, 2.1 Hz, 1H), 7.77 (d, J = 2.2 Hz, 1H), 7.65 (d, J = 2.2 Hz, 1H), 7.52 (dd, J = 8.0, 4.8 Hz, 1H), 3.79 (s, 2H), 3.77 (s, 2H), 2.39 (s, 3H). 13C NMR (150 MHz, DMSO‑d6) δ 186.63, 170.78, 151.71, 150.22, 137.73 (d, J = 13.7 Hz), 137.11, 135.70, 132.28 (d, J = 30.0 Hz), 131.04 (q, J = 31.5 Hz), 130.93 (d, J = 3.7 Hz), 124.21, 123.65 (q, J = 271.5 Hz), 56.57, 55.91, 45.40. HRMS (ESI): m/z calcd. for C21H17F6N2O, [M + H]+, 427.1240; found: 427.1242.
2.2.12 (E)-3-((E)-2-fluoro-3-(trifluoromethyl)benzylidene)-1-methyl-5-(pyridin-3-ylmethylene)piperidin-4-one (4l)
Yellow powder; yield: 57 %; Mp:133.3 ∼ 135.1 °C; 1H NMR (600 MHz, DMSO‑d6) δ 8.73 (d, J = 2.3 Hz, 1H), 8.60 (dd, J = 4.8, 1.6 Hz, 1H), 7.99–7.89 (m, 1H), 7.82 (dt, J = 28.8, 7.4 Hz, 2H), 7.73–7.60 (s, 2H), 7.58–7.42 (m, 2H), 3.78 (s, 2H), 3.64 (s, 2H), 2.33 (s, 3H). 13C NMR (150 MHz, DMSO‑d6) δ 186.27, 157.43 (d, J = 249.7 Hz), 151.70, 150.23, 137.72, 137.34, 136.01, 135.56, 132.70, 130.89, 128.38 (d, J = 3 Hz), 125.59 (d, J = 3.9 Hz), 124.46 (d, J = 12.5 Hz), 124.21, 123.00 (q, J = 272.3 Hz), 117.56(dd, J = 30, 12.3 Hz), 56.71, 56.12, 45.50. HRMS (ESI): m/z calcd. for C20H17F4N2O, [M + H]+, 377.1272; found: 377.1279.
2.2.13 (E)-3-((E)-2-fluoro-4-(trifluoromethyl)benzylidene)-1-methyl-5-(pyridin-3-ylmethylene)piperidin-4-one (4m)
Yellow powder; yield: 40 %; Mp:123.8 ∼ 125.3 °C; 1H NMR (600 MHz, DMSO‑d6) δ 8.73 (d, J = 2.5 Hz, 1H), 8.60 (dd, J = 4.8 Hz, 1.6 Hz, 1H), 7.93 (dt, J = 8.0 Hz, 2.1 Hz 1H), 7.82 (dd, J = 10.4 Hz, 1.6 Hz, 1H), 7.74–7.59 (m, 4H), 7.54–7.45 (m, 1H), 3.78 (s, 2H), 3.65 (s, 2H), 2.37 (s, 3H). 13C NMR (150 MHz, DMSO‑d6) δ 186.32, 160.42 (d, J = 249.0 Hz), 151.74, 150.27, 137.73 (q, J = 3.3 Hz), 135.56, 132.68 (d, J = 24.9 Hz), 131.49 (q, J = 32.4, 8.6 Hz), 127.09 (d, J = 13.4 Hz), 123.66 (q, J = 271.5 Hz), 121.96, 113.79 (d, J = 24.9 Hz), 56.74, 56.20, 45.52. HRMS (ESI): m/z calcd. for C20H17F4N2O, [M + H]+, 377.1272; found: 377.1276.
2.2.14 (E)-3-((E)-2-fluoro-5-(trifluoromethyl)benzylidene)-1-methyl-5-(pyridin-3-ylmethylene)piperidin-4-one (4n)
Yellow powder; yield: 41 %; Mp:133.0 ∼ 134.7 °C; 1H NMR (600 MHz, DMSO‑d6) δ 8.74 (d, J = 2.3 Hz, 1H), 8.60 (dd, J = 4.7, 1.6 Hz, 1H), 7.93 (dt, J = 7.9, 2.0 Hz, 1H), 7.89 (m, 1H), 7.81 (dd, J = 6.7, 2.4 Hz, 1H), 7.67–7.63 (m, 2H), 7.59 (t, J = 9.3 Hz, 1H), 7.51 (m, 1H), 3.79 (s, 2H), 3.61 (s, 2H), 2.36 (s, 3H). 13C NMR (150, DMSO‑d6) δ 186.26, 162.43 (d, J = 254.6 Hz), 151.73, 150.25, 137.57, 137.41, 135.55, 132.74, 130.91, 129.09 (q, J = 10.0, 4.1 Hz), 128.66 (q, J = 4.1 Hz), 126.18, 126.01 (d, J = 33.0 Hz), 124.22, 124.12, 123.93 (q, J = 270.0 Hz), 117.81 (dd, J = 24.2, 9.2 Hz), 117.66 (d, J = 23.6 Hz), 56.72, 55.95, 45.44. HRMS (ESI): m/z calcd. for C20H17F4N2O, [M + H]+, 377.1272; found: 377.1275.
2.2.15 (E)-3-((E)-2-fluoro-6-(trifluoromethyl)benzylidene)-1-methyl-5-(pyridin-3-ylmethylene)piperidin-4-one (4o)
Yellow powder; yield: 42 %; Mp:133.0 ∼ 134.7 °C; 1H NMR (600 MHz, DMSO‑d6) δ 8.74 (d, J = 2.3 Hz, 1H), 8.61 (dd, J = 4.8, 1.6 Hz, 1H), 7.93 (dt, J = 8.0, 2.0 Hz, 1H), 7.79–7.65 (m, 4H), 7.62–7.48 (m, 2H), 3.77 (s, 2H), 3.26 (s, 2H), 2.28 (s, 3H). 13C NMR (150 MHz, DMSO‑d6) δ 185.65, 159.14 (d, J = 246.9 Hz), 151.72, 150.30, 138.71, 137.78, 135.24, 133.54, 131.88 (d, J = 9.5 Hz), 130.87, 129.44 (q, J = 33.0 Hz), 124.81, 124.19, 123.71 (q, J = 276.0 Hz), 122.92, 121.48 (dd, J = 23.4, 4.7 Hz), 121.09 (d, J = 23.4 Hz), 56.70, 55.73, 45.20. HRMS (ESI): m/z calcd. for C20H17F4N2O, [M + H]+, 377.1272; found: 377.1278.
2.2.16 (E)-3-((E)-3-fluoro-4-(trifluoromethyl)benzylidene)-1-methyl-5-(pyridin-3-ylmethylene)piperidin-4-one (4p)
Yellow powder; yield: 38 %; Mp:147.0 ∼ 149.0 °C; 1H NMR (600 MHz, DMSO‑d6) δ 8.73 (d, J = 2.3 Hz, 1H), 8.60 (dd, J = 4.8, 1.6 Hz, 1H), 7.93 (dt, J = 8.1, 2.0 Hz, 1H), 7.87 (t, J = 7.9 Hz, 1H), 7.67 (d, J = 11.9 Hz, 1H), 7.63 (q, J = 2.3 Hz, 2H), 7.54 (dt, 2H), 3.77 (s, 2H), 3.77 (s, 2H), 2.40 (s, 3H). 13C NMR (150 MHz, DMSO‑d6) δ 186.72, 160.02, 158.34(d, J = 252.0 Hz), 151.71, 150.23, 142.05 (d, J = 8.5 Hz), 137.44, 135.70, 132.32, 130.91, 127.98 (d, J = 4.7 Hz), 127.16 (d, J = 3.7 Hz), 124.22, 123.91, 122.10 (q, J = 271.5 Hz), 118.85 (d, J = 21.1 Hz), 116.86 (dd, J = 32.2, 12.4 Hz), 56.61, 56.31, 45.56. HRMS (ESI): m/z calcd. for C20H17F4N2O, [M + H]+, 377.1272; found: 377.1275.
2.2.17 (E)-3-((E)-3-fluoro-5-(trifluoromethyl)benzylidene)-1-methyl-5-(pyridin-3-ylmethylene)piperidin-4-one (4q)
Yellow powder; yield: 35 %; Mp:120.4 ∼ 122.1 °C; 1H NMR (600 MHz, DMSO‑d6) δ 8.72 (d, J = 2.3 Hz, 1H), 8.59 (dd, J = 4.9 Hz, 1.6 Hz, 1H), 7.92 (dt, J = 8.1 Hz, 2.0 Hz, 1H), 7.80–7.70 (m, 2H), 7.70–7.59 (m, 3H), 7.50 (dd, J = 7.9 Hz, 4.8 Hz, 1H), 3.83 (s, 2H), 3.69 (s, 2H), 2.39 (s, 3H). 13C NMR (150 MHz, DMSO‑d6) δ 186.09, 161.78(d, J = 246.0 Hz), 151.09, 149.59, 136.06 (d, J = 7.7 Hz), 135.13, 131.84, 131.61 (dd, J = 32.7, 8.9 Hz), 131.01, 125.73, 123.93 (d, J = 4.7 Hz), 123.60, 123.01 (q, J = 271.5 Hz), 122.11, 120.47 (d, J = 21 Hz), 113.11 (dd, J = 24.8, 3.8 Hz), 55.98, 55.55, 44.92. HRMS (ESI): m/z calcd. for C20H17F4N2O, [M + H]+, 377.1272; found: 377.1277.
2.2.18 (E)-3-((E)-4-fluoro-2-(trifluoromethyl)benzylidene)-1-methyl-5-(pyridin-3-ylmethylene)piperidin-4-one (4r)
Yellow powder; yield: 39 %; Mp:164.0 ∼ 166.0 °C; 1H NMR (600 MHz, DMSO‑d6) δ 8.73 (d, J = 2.3 Hz, 1H), 8.60 (dd, J = 4.8, 1.6 Hz, 1H), 7.93 (dt, J = 8.1, 2.0 Hz, 1H), 7.87 (t, J = 7.9 Hz, 1H), 7.67 (d, J = 11.9 Hz, 1H), 7.63 (q, J = 2.3 Hz, 2H), 7.54 (dt, 2H), 3.77 (s, 2H), 3.76 (s, 2H), 2.40 (s, 3H). 13C NMR (150 MHz, DMSO‑d6) δ 186.75, 159.19 (d, J = 253.6 Hz), 151.71, 150.23, 142.06 (d, J = 8.7 Hz), 137.71, 137.21, 135.74, 132.30, 130.92, 128.01 (q, J = 4.9 Hz), 127.15, 124.22, 123.01 (d, J = 271.9 Hz), 122.11, 118.85 (d, J = 21.1 Hz), 116.85 (d, J = 31.5 Hz),116.85 (d, J = 21.1 Hz), 56.63, 56.32, 45.57. HRMS (ESI): m/z calcd. for C20H17F4N2O, [M + H]+, 377.1272; found: 377.1274.
2.2.19 (E)-3-((E)-4-fluoro-3-(trifluoromethyl)benzylidene)-1-methyl-5-(pyridin-3-ylmethylene)piperidin-4-one (4s)
Yellow powder; yield: 43 %; Mp:126.5 ∼ 128.5 °C; 1H NMR (600 MHz, DMSO‑d6) δ 8.73 (d, J = 2.3 Hz, 1H), 8.60 (dd, J = 4.8, 1.6 Hz, 1H), 7.93 (ddt, J = 8.1, 4.0, 2.0 Hz, 2H), 7.90–7.84 (m, 1H), 7.68 (d, J = 2.1 Hz, 1H), 7.66–7.59 (m, 2H), 7.51 (ddd, J = 8.0, 4.8, 0.8 Hz, 1H), 3.77 (s, 2H), 3.76 (s, 2H), 2.39 (s, 3H). 13C NMR (150 MHz, DMSO‑d6) δ 186.66, 159.26(d, J = 255.0 Hz), 151.66, 150.16, 137.66, 137.15 (d, J = 8.6 Hz), 135.83, 135.37, 132.82, 132.25, 132.01, 130.97, 129.96 (q, J = 4.7 Hz), 124.21, 122.88(q, J = 270.0 Hz), 118.17 (d, J = 20.8 Hz), 117.48 (qd, J = 33.0, 12.0 Hz), 56.60, 56.32, 45.59. HRMS (ESI): m/z calcd. for C20H17F4N2O, [M + H]+, 377.1272; found: 377.1275.
2.3 General procedure for the preparation of asymmetric BAP derivatives 5a-s
In a 25 mL beaker, dissolve 4-pyridinobenzaldehyde (0.54 g, 5.00 mmol), N-methylpiperidone (0.57 g, 5.00 mmol), and 3-fluorobenzaldehyde (0.62 g, 5.00 mmol) in 10 mL acetic acid Dry hydrogen chloride gas was consistently injected for forty-five min, and stirred at 25 °C for 10 h, at some point of which TLC tracked the reaction. After the reaction, the precipitate was withdrawn and filtered. After the precipitation is dissolved in distilled water, alter the pH value to 8–9 with 10 % NaOH solution. The resultant precipitation is subjected to silica gel column chromatography (Petroleum ether: Ethyl acetate: methanol = 10: 10: 1) to attain compound 5a as a yellow solid. Compound 5b-s was prepared in accordance with the instruction approach of compound 5a.
2.3.1 (E)-3-((E)-3-fluorobenzylidene)-1-methyl-5-(pyridin-4-ylmethylene)piperidin-4-one (5a)
Yellow powder; yield: 65 %; Mp:107.5 ∼ 109.1 °C; 1H NMR (600 MHz, DMSO‑d6) δ 8.71–8.64 (m, 2H), 7.60 (s, 1H), 7.56–7.49 (m, 2H), 7.49–7.44 (m, 2H), 7.37 (t, J = 8.1 Hz, 2H), 7.29 (td, J = 8.7, 2.6 Hz, 1H), 3.76 (t, J = 2.6 Hz, 4H), 2.40 (s, 3H). 13C NMR (150 MHz, DMSO‑d6) δ 186.92, 162.55 (d, J = 241.7 Hz), 150.51, 142.19, 137.71, 137.26 (d, J = 8.4 Hz), 135.05, 134.28, 132.24, 131.19 (d, J = 7.8 Hz), 127.11 (d, J = 3.2 Hz), 124.66, 117.35 (d, J = 22.3 Hz), 116.64 (d, J = 21.2 Hz), 56.60, 56.48, 45.63. HRMS (ESI): m/z calcd. for C19H17FN2O, [M + H]+, 309.1398; found: 309.1398.
2.3.2 (E)-3-((E)-4-fluorobenzylidene)-1-methyl-5-(pyridin-4-ylmethylene)piperidin-4-one (5b)
Yellow powder; yield: 63 %; Mp:145.4 ∼ 147.4 °C; 1H NMR (600 MHz, DMSO‑d6) δ 8.66 (d, J = 5.3 Hz, 2H), 7.66–7.55 (m, 3H), 7.52 (s, 1H), 7.45 (d, J = 4.9 Hz, 2H), 7.32 (t, J = 8.7 Hz, 2H), 3.74 (s, 2H), 3.74 (s, 2H), 2.39 (s, 3H). 13C NMR (150 MHz, DMSO‑d6) δ 186.85, 162.94 (d, J = 246.5 Hz), 150.51, 142.27, 137.79, 134.66, 133.64, 133.46 (d, J = 8.1 Hz), 132.03, 131.54 (d, J = 3.5 Hz), 124.66, 116.32 (d, J = 21.2 Hz), 56.73, 56.49, 45.68. HRMS (ESI): m/z calcd. for C19H17FN2O, [M + H]+, 309.1398; found: 309.1403.
2.3.3 (E)-1-methyl-3-(pyridin-4-ylmethylene)-5-((E)-3(trifluoromethyl)benzylidene)piperidin-4-one (5c)
Yellow powder; yield: 52 %; Mp:104.7 ∼ 106.4 °C; 1H NMR (600 MHz, DMSO‑d6) δ 8.67 (dd, J = 4.6, 1.4 Hz, 2H), 7.86 (s, 1H), 7.79 (t, J = 7.3 Hz, 2H), 7.76–7.67 (m, 2H), 7.54 (s, 1H), 7.49–7.44 (m, 2H), 3.77 (dd, J = 4.1, 1.4 Hz, 4H), 2.39 (s, 3H). 13C NMR (150 MHz, DMSO‑d6) δ 186.85, 150.52, 142.17, 137.65, 136.00, 135.74, 134.15, 134.01, 132.39, 130.31, 129.99 (q, J = 31.5 Hz), 127.74 (d, J = 3 Hz), 127.15, 126.11 (d, J = 3.4 Hz), 124.45(q, J = 271 Hz), 124.26, 56.45, 45.56. HRMS (ESI): m/z calcd. for C20H17F4N2O, [M + H]+, 377.1272; found: 377.1271.
2.3.4 (E)-3-((E)-2,3-difluorobenzylidene)-1-methyl-5-(pyridin-4-ylmethylene)piperidin-4-one (5d)
Yellow powder; yield: 65 %; Mp:136.2 ∼ 138.1 °C; 1H NMR (600 MHz, DMSO‑d6) δ 8.67 (dd, J = 4.5, 1.5 Hz, 2H), 7.65 (d, J = 8.5 Hz, 1H), 7.58–7.50 (s, 2H), 7.47 (dd, J = 4.8, 1.3 Hz, 2H), 7.32 (ddd, J = 9.1, 7.7, 4.9 Hz, 2H), 3.76 (s, 2H), 3.67 (s, 2H), 2.37 (s, 3H). 13C NMR (150 MHz, DMSO‑d6) δ 186.47, 150.52, 150.36 (dd, J = 244.5, 12.8 Hz), 148.50 (dd, J = 247.5, 13.5 Hz), 142.09, 137.40, 136.75, 132.95, 126.56, 126.27, 125.52 (d, J = 9.9 Hz), 124.96 (d, J = 9.9 Hz), 124.67, 118.89 (d, J = 16.9 Hz), 56.57, 56.31, 45.51. HRMS (ESI): m/z calcd. for C19H17F2N2O, [M + H]+, 327.1304; found: 377.1307.
2.3.5 (E)-3-((E)-3,4-difluorobenzylidene)-1-methyl-5-(pyridin-4-ylmethylene)piperidin-4-one (5e)
Yellow powder; yield: 38 %; Mp:106.4 ∼ 107.6 °C; 1H NMR (600 MHz, DMSO‑d6) δ 8.75–8.61 (m, 2H), 7.68–7.60 (m, 1H), 7.59–7.49 (m, 3H), 7.45 (d, J = 5.1 Hz, 2H), 7.42–7.35 (m, 1H), 3.74 (s, 2H), 3.74 (s, 2H), 2.39 (s, 3H). 13C NMR (150 MHz, DMSO‑d6) δ 186.38, 149.79 (dd, J = 247.5, 12.6 Hz), 149.33(dd, J = 244 0.5, 12.6 Hz), 137.20, 134.32, 133.04, 132.18 (d, J = 4.7 Hz), 127.89 (dd, J = 6.9, 3.1 Hz), 124.20, 119.40 (d, J = 17.4 Hz), 117.91 (d, J = 17.4 Hz), 55.99, 45.15. HRMS (ESI): m/z calcd. for C19H17F2N2O, [M + H]+, 327.1304; found: 377.1310.
2.3.6 (E)-3-((E)-2,4-difluorobenzylidene)-1-methyl-5-(pyridin-4-ylmethylene)piperidin-4-one (5f)
Yellow powder; yield: 52 %; Mp:164.0 ∼ 166.0 °C; 1H NMR (600 MHz, DMSO‑d6) δ 8.69–8.65 (m, 2H), 7.62 (s, 1H), 7.55 (s, 2H), 7.46 (d, J = 4.6 Hz, 2H), 7.45–7.35 (m, 1H), 7.22 (td, J = 8.4, 2.4 Hz, 1H), 3.76 (s, 2H), 3.64 (s, 2H), 2.37 (s, 3H). 13C NMR (150 MHz, DMSO‑d6) δ 186.54, 163.34 (d, J = 249.0, 12.5 Hz), 161.25 (d, J = 250.5, 12.4 Hz), 150.54, 142.16, 137.50, 135.55, 132.77, 132.73, 126.62 (d, J = 9.9 Hz), 124.68, 119.35 (dd, J = 21.4, 3.6 Hz), 112.55 (dd, J = 21.1, 3.7 Hz), 105.04 (t, J = 26.4 Hz), 56.57, 56.38, 45.56. HRMS (ESI): m/z calcd. for C19H17F2N2O, [M + H]+, 327.1304; found: 377.1303.
2.3.7 (E)-3-((E)-2,5-difluorobenzylidene)-1-methyl-5-(pyridin-4-ylmethylene)piperidin-4-one (5g)
Yellow powder; yield: 53 %; Mp:161.0 ∼ 163.0 °C; 1H NMR (600 MHz, DMSO‑d6) δ 8.67 (d, J = 6.1 Hz, 2H), 7.59 (s, 1H), 7.55 (s, 1H), 7.47 (d, J = 6.3 Hz, 2H), 7.44–7.31 (m,3H), 3.76 (s,2H), 3.66 (s, 2H), 2.37 (s, 3H). 13C NMR (150 MHz, DMSO‑d6) δ 186.57, 158.71 (dd, J = 240, 3 Hz), 157.05(dd, J = 244.5, 3 Hz), 150.55, 142.10, 137.43, 136.77, 132.92, 126.47, 124.69, 124.06 (dd, J = 24.9, 8.7 Hz), 118.44 (dd, J = 24.3, 9.1 Hz), 117.83 (dd, J = 24.9, 8.8 Hz), 117.46 (d, J = 25.0 Hz), 56.58, 56.12, 45.51. HRMS (ESI): m/z calcd. for C19H17F2N2O, [M + H]+, 327.1304; found: 377.1305.
2.3.8 (E)-3-((E)-2,6-difluorobenzylidene)-1-methyl-5-(pyridin-4-ylmethylene)piperidin-4-one (5h)
Yellow powder; yield: 30 %; Mp:133.0 ∼ 134.7 °C; 1H NMR (600 MHz, DMSO‑d6) δ 8.68 (d, J = 6.0 Hz, 2H), 7.61–7.52 (m, 2H), 7.49–7.43 (m, 3H), 7.24 (t, J = 8.2 Hz, 2H), 3.77 (s, 2H), 3.40 (s, 2H), 2.31 (s, 3H). 13C NMR (150 MHz, DMSO‑d6) δ 185.97, 160.08 (d, J = 247.5 Hz), 160.03 (d, J = 247.5 Hz), 150.52, 142.09, 138.07, 137.23, 133.49, 132.43 (t, J = 10.5 Hz), 124.69, 122.63, 112.43 (dd, J = 21.2, 4.0 Hz), 112.09 (t, J = 19.7 Hz), 56.79, 56.32, 45.39. HRMS (ESI): m/z calcd. for C19H17F2N2O, [M + H]+, 327.1304; found: 377.1308.
2.3.9 (E)-3-((E)-3,5-difluorobenzylidene)-1-methyl-5-(pyridin-4-ylmethylene)piperidin-4-one (5i)
Yellow powder; yield: 66 %; Mp:139.0 ∼ 140.9 °C; 1H NMR (600 MHz, DMSO‑d6) δ 8.69–8.65 (m, 2H), 7.56 (d, J = 2.2 Hz, 1H), 7.53 (d, J = 2.2 Hz, 1H), 7.48–7.44 (m, 2H), 7.34 (tt, J = 9.3, 2.4 Hz, 1H), 7.27 (h, J = 4.7 Hz, 2H), 3.75 (dd, J = 4.4, 2.2 Hz, 4H), 2.39 (s, 3H). 13C NMR (150 MHz, DMSO‑d6) δ 186.95, 162.79 (d, J = 245.3, 12.7 Hz), 150.54, 142.12, 138.37 (t, J = 9.8 Hz), 137.62, 136.13, 133.12, 132.46, 124.68, 113.83 (dd, J = 19.9, 5.1 Hz), 105.15 (t, J = 25.8 Hz), 56.46, 56.34, 45.57. HRMS (ESI): m/z calcd. for C19H17F2N2O, [M + H]+, 327.1304; found: 377.1306.
2.3.10 (E)-3-((E)-2,4-bis(trifluoromethyl)benzylidene)-1-methyl-5-(pyridin-4-ylmethylene)piperidin-4-one (5j)
Yellow powder; yield: 36 %; Mp:150.8 ∼ 152.2 °C; 1H NMR (600 MHz, DMSO‑d6) δ 8.71–8.61 (m, 2H), 8.23–8.02 (m, 2H), 7.83–7.68 (m, 2H), 7.58 (d, J = 2.2 Hz, 1H), 7.50–7.38 (m, 2H), 3.76 (s, 2H), 3.54 (s, 2H), 2.30 (s, 3H). 13C NMR (150 MHz, DMSO‑d6) δ 186.33, 150.56, 142.03, 137.73 (d, J = 28.4 Hz), 137.13, 133.61, 132.64, 130.15 (d, J = 4.5 Hz), 129.92 (d, J = 34.5 Hz), 128.99 (d, J = 34.5 Hz), 123.75(q, J = 271.5 Hz), 123.66 (q, J = 273.0 Hz), 124.72, 123.67 (q, J = 4.5 Hz), 56.56, 55.55, 45.30. HRMS (ESI): m/z calcd. for C21H17F6N2O, [M + H]+, 427.1240; found: 427.1244.
2.3.11 (E)-3-((E)-3,5-bis(trifluoromethyl)benzylidene)-1-methyl-5-(pyridin-4-ylmethylene)piperidin-4-one (5k)
Yellow powder; yield: 38 %; Mp:131.5 ∼ 132.5 °C; 1H NMR (600 MHz, DMSO‑d6) δ 8.68 (dd, J = 4.5, 1.6 Hz, 2H), 8.16 (s, 3H), 7.77 (s, 1H), 7.56 (s, 1H), 7.47 (dd, J = 4.8, 1.3 Hz, 2H), 3.78 (s, 2H), 3.78 (s, 2H), 2.38 (s, 3H). 13C NMR (150 MHz, DMSO‑d6) δ 186.79, 150.55, 142.06, 137.66, 137.49, 136.96, 137.57 (d, J = 25.1 Hz), 137.22, 132.64, 131.25 (d, J = 32.7 Hz), 131.03(q, J = 33 Hz), 130.98 (d, J = 3.7 Hz), 130.92, 124.68, 123.64 (q, J = 271 Hz), 122.80, 56.14, 45.37. HRMS (ESI): m/z calcd. for C21H17F6N2O, [M + H]+, 427.1240; found: 427.1243.
2.3.12 (E)-3-((E)-2-fluoro-3-(trifluoromethyl)benzylidene)-1-methyl-5-(pyridin-4-ylmethylene)piperidin-4-one (5l)
Yellow powder; yield: 55 %; Mp:133.6 ∼ 134.6 °C; 1H NMR (600 MHz, DMSO‑d6) δ 8.68 (d, J = 5.9 Hz, 2H), 7.83 (dt, J = 29.4, 7.2 Hz, 2H), 7.66 (s, 1H), 7.57 (s, 1H)7.54 (dd, J = 21.2, 13.4 Hz, 1H), 7.47 (d, J = 5.8 Hz, 2H), 3.78 (s, 2H), 3.66 (s, 2H), 2.36 (s, 3H). 13C NMR (150 MHz, DMSO‑d6) δ 186.41, 157.46 (d, J = 257.8 Hz), 150.53, 142.06, 136.00, 133.07, 128.45, 125.87 (d, J = 4.8 Hz), 125.59 (d, J = 3.9 Hz), 123.49 (q, J = 270 Hz), 124.68, 124.39 (d, J = 12.4 Hz), 117.57 (dd, J = 32.3, 12.4 Hz), 56.56, 56.11, 45.46. HRMS (ESI): m/z calcd. for C20H17F4N2O, [M + H]+, 377.1272; found: 377.1270.
2.3.13 (E)-3-((E)-2-fluoro-4-(trifluoromethyl)benzylidene)-1-methyl-5-(pyridin-4-ylmethylene)piperidin-4-one (5m)
Yellow powder; yield: 32 %; Mp:129.7 ∼ 131.1 °C; 1H NMR (600 MHz, DMSO‑d6) δ 8.69–8.65 (m, 2H), 7.82 (dd, J = 10.4 Hz, 1.7 Hz, 1H), 7.69 (td, J = 8.7 Hz, 8.3 Hz, 4.2 Hz, 2H), 7.65 (m, 1H), 7.56 (d, J = 2.3 Hz, 1H), 7.49–7.45 (m, 2H), 3.77 (s, 2H), 3.65 (s, 2H), 2.36 (s, 3H). 13C NMR (150 MHz, DMSO‑d6) δ 186.49, 160.44(d, J = 249.0 Hz), 150.55, 142.07, 137.61, 137.37, 133.13, 132.60 (d, J = 26.0 Hz), 131.55 (q, J = 32.5, 8.6 Hz), 127.01 (d, J = 13.4 Hz), 123.64 (q, J = 271.5 Hz), 126.12 (d, J = 3.8 Hz), 124.70, 124.55, 122.74, 121.97, 120.93, 113.80 (dd, J = 26.0, 3.9 Hz), 56.59, 56.17, 45.47. HRMS (ESI): m/z calcd. for C20H17F4N2O, [M + H]+, 377.1272; found: 377.1274.
2.3.14 (E)-3-((E)-2-fluoro-5-(trifluoromethyl)benzylidene)-1-methyl-5-(pyridin-4-ylmethylene)piperidin-4-one (5n)
Yellow powder; yield: 38 %; Mp:133.0 ∼ 134.7 °C; 1H NMR (600 MHz, DMSO‑d6) δ 8.68–8.67 (m, 2H), 7.90 (ddd, J = 8.6, 4.5, 2.3 Hz, 1H), 7.82 (dd, J = 6.7, 2.4 Hz, 1H), 7.64 (s, 1H), 7.61–7.56 (m, 2H), 7.48–7.46 (m, 2H), 3.78 (s, 2H), 3.62 (s, 2H), 2.35 (s, 3H). 13C NMR (150 MHz, DMSO‑d6) δ 186.44, 162.45 (d, J = 254.5 Hz), 150.56, 142.08, 137.35 (d, J = 10.3 Hz), 133.12, 126.48 (dd, J = 9.8, 4.5 Hz), 126.39–125.64 (m), 124.70 (d, J = 31.1 Hz), 123.85 (d, J = 23.5 Hz), 123.75 (q, J = 270.0 Hz), 117.69 (d, J = 23.5 Hz), 56.56, 55.92, 45.39. HRMS (ESI): m/z calcd. for C20H17F4N2O, [M + H]+, 377.1272; found: 377.1277.
2.3.15 (E)-3-((E)-2-fluoro-6-(trifluoromethyl)benzylidene)-1-methyl-5-(pyridin-4-ylmethylene)piperidin-4-one (5o)
Yellow powder; yield: 41 %; Mp:133.0 ∼ 134.7 °C; 1H NMR (600 MHz, DMSO‑d6) δ 8.70–8.66 (m, 2H), 7.75–7.69 (m, 3H), 7.61 (d, J = 2.3 Hz, 1H), 7.53 (q, J = 2.3 Hz, 1H), 7.49–7.45 (m, 2H), 3.76 (d, J = 2.3 Hz, 2H), 3.27 (s, 2H), 2.27 (s, 3H). 13C NMR (150 MHz, DMSO‑d6) δ 185.81, 159.14 (d, J = 247.0 Hz), 150.53, 142.06, 138.61, 137.00, 133.92, 131.93 (d, J = 9.5 Hz), 129.86 (d, J = 30.3 Hz), 125.16, 124.70, 123.71 (q, J = 273.0 Hz), 122.90, 121.39 (d, J = 19.7 Hz), 121.10 (d, J = 23.4 Hz), 56.54, 55.68, 45.14. HRMS (ESI): m/z calcd. for C20H17F4N2O, [M + H]+, 377.1272; found: 377.1276.
2.3.16 (E)-3-((E)-3-fluoro-4-(trifluoromethyl)benzylidene)-1-methyl-5-(pyridin-4-ylmethylene)piperidin-4-one (5p)
Yellow powder; yield: 38 %; Mp:164.0 ∼ 166.0 °C; 1H NMR (600 MHz, DMSO‑d6) δ 8.67 (d, J = 6.0 Hz, 2H), 7.87 (t, J = 8.0 Hz, 1H), 7.68 (d, J = 11.9 Hz, 1H), 7.63 (s, 1H), 7.54 (d, J = 10.4 Hz, 2H), 7.47 (d, J = 6.2 Hz, 2H), 3.77 (s, 2H), 2.39 (s, 3H). 13C NMR (150 MHz, DMSO‑d6) δ 186.89, 160.05, 158.26 (d, J = 268.5 Hz), 150.55, 142.08, 141.95 (d, J = 8.8 Hz), 137.30, 132.65, 128.05 (d, J = 4.9 Hz), 127.20 (d, J = 3.7 Hz), 124.69, 122.24 (dq, J = 271.5 Hz), 118.89 (d, J = 21.1 Hz), 117.07 (dd, J = 33.1, 11.5 Hz), 56.47, 56.29, 45.52. HRMS (ESI): m/z calcd. for C20H17F4N2O, [M + H]+, 377.1272; found: 377.1279.
2.3.17 (E)-3-((E)-3-fluoro-5-(trifluoromethyl)benzylidene)-1-methyl-5-(pyridin-4-ylmethylene)piperidin-4-one (5q)
Yellow powder; yield: 35 %; Mp:162.8 ∼ 164.2 °C; 1H NMR (600 MHz, DMSO‑d6) δ 8.72–8.63 (m, 2H), 7.80–7.71 (m, 2H), 7.72–7.64 (m, 2H), 7.54 (d, J = 2.2 Hz, 1H), 7.50–7.42 (m, 2H), 3.77 (s, 2H), 3.77 (s, 2H), 2.39 (s, 3H). 13C NMR (150 MHz, DMSO‑d6) δ 186.91, 162.40 (d, J = 246.0 Hz), 150.55, 142.10, 138.67 (d, J = 9.0 Hz), 137.59, 136.59, 132.68, 131.85 (dd, J = 32.3, 8.7 Hz), 124.53, 124.38, 123.81, 123.80 (d, J = 4.2 Hz), 123.60 (q, J = 271.5 Hz),122.73, 121.12 (d, J = 22.3 Hz), 113.73 (d, J = 25.6 Hz), 56.45, 56.14, 45.49. HRMS (ESI): m/z calcd. for C20H17F4N2O, [M + H]+, 377.1272; found: 377.1280.
2.3.18 (E)-3-((E)-4-fluoro-2-(trifluoromethyl)benzylidene)-1-methyl-5-(pyridin-4-ylmethylene)piperidin-4-one (5r)
Yellow powder; yield: 33 %; Mp:133.0 ∼ 134.7 °C; 1H NMR (600 MHz, DMSO‑d6) δ 8.69–8.65 (m, 2H), 7.78 (dd, J = 9.1, 2.7 Hz, 1H), 7.75 (d, J = 4.5 Hz, 1H), 7.65 (td, J = 8.5, 2.7 Hz, 1H), 7.61–7.54 (m, 2H), 7.49–7.45 (m, 2H), 3.76 (d, J = 2.4 Hz, 2H), 3.54 (d, J = 2.0 Hz, 2H), 2.32 (s, 3H). 13C NMR (150 MHz, DMSO‑d6) δ 186.42, 162.02 (d, J = 249.3 Hz), 150.55, 142.09, 137.25, 136.35, 134.00 (d, J = 8.4 Hz), 133.29, 130.37, 130.11 (dq, J = 30.0, J = 9 Hz), 129.66 (d, J = 4.7 Hz), 124.69, 123.11 (q, J = 271.5 Hz), 120.10 (d, J = 20.8 Hz), 115.17 (dd, J = 20.8, 4.7 Hz), 56.59, 55.73, 45.39. HRMS (ESI): m/z calcd. for C20H17F4N2O, [M + H]+, 377.1272; found: 377.1277.
2.3.19 (E)-3-((E)-4-fluoro-3-(trifluoromethyl)benzylidene)-1-methyl-5-(pyridin-4-ylmethylene)piperidin-4-one (5s)
Yellow powder; yield: 52 %; Mp:136.2 ∼ 138.1 °C; 1H NMR (600 MHz, DMSO‑d6) δ 8.66 (d, J = 6.1 Hz, 2H), 7.92 (d, J = 7.0 Hz, 1H), 7.90–7.82 (m, 1H), 7.67 (s, 1H), 7.65–7.57 (m, 1H), 7.53 (s, 1H), 7.45 (d, J = 6.1 Hz, 2H), 3.75 (s, 2H), 3.75 (s, 2H), 2.38 (s, 3H).13C NMR (150 MHz, DMSO‑d6) δ 186.77, 159.28 (d, J = 255.3 Hz), 150.51, 142.14, 137.61, 137.18 (d, J = 8.9 Hz), 135.23, 133.10, 132.40, 132.15 (d, J = 3.7 Hz), 130.00 (q, J = 4.6 Hz), 124.66, 122.85(q, J = 271.5 Hz), 118.17 (dd, J = 20.5, 0.7 Hz), 117.48 (qd, J = 31.5, 12.0 Hz), 56.45, 56.30, 45.54. HRMS (ESI): m/z calcd. for C20H17F4N2O, [M + H]+, 377.1272; found: 377.1275.
2.4 Cell culture and CCK-8 assay for RAW 264.7cell cytotoxicity
Mouse RAW264.7 cells were kindly provided by Procell (Wuhan, China) and cultured in a complete medium consisting of DMEM supplemented with 10 % FBS and 1 % streptomycin/penicillin antibiotics. Cells of the logarithmic growth phase were recruited for the following tests. Detect cytotoxicity using the CCK-8 assay according to the manufacturer's instructions. Cells are grown and treated in 96-well plates and incubated with CCK-8 reagent for 1 h at 37 °C. Absorbance is measured at 450 nm.
2.5 Anti-inflammatory testing of all derivatives
RAW264.7 cells were randomly grouped. Cells were re-exposed for 24 h in the presence of 1.0 µg/mL LPS. After treatment, cells were collected and analyzed. TNF-α and IL-6 were assayed according to the ELISA double antibody sandwich kit assay instructions, and absorbance values at 450 nm were determined on the ELISA instrument after the assay. A standard curve was established for each measurement in pg/mL.
2.6 Western blotting
RAW264.7 cells (2.0 × 106 cells per well in 6-well plates) were exposed to the drug for 2.0 h and then to 1.0 µg/mL LPS for 24 h. Lysis was performed with a potent RIPA lysate containing a phosphatase inhibitor (RIPA lysate: phosphatase inhibitor = 99: 1). After complete sonication lysis on ice, centrifuge. After determining the protein concentration, the protein solution was boiled. Protein samples were added to a 10 % polyacrylamide gel for electrophoresis. The proteins were transferred onto PVDF membranes and incubated with 5 % skim milk for 2.0 h. Subsequently incubated with IκB-α, p-IκB-α, p65, p-p65, JNK2, p-JNK, p-38, p-p38, ERK, p-ERK, β-actin (antibodies purchased from Cell Signaling Technology, USA) antibodies at 4 °C overnight. Incubate with goat anti-rabbit IgG/HRP antibody (Solarbio, Beijing, China) for 1.0 h at room temperature. Testing was performed after using ECL developer (Novland Biopharma, Shanghai, China). Protein intensity was detected using Image Lab software and analyzed using ImageJ software.
2.7 Apoptosis assay
RAW264.7 cells were inoculated at a density of 2 × 105 cells/well in 12-well plates. The plates were then treated with 4 k (0.275, 0.55, and 1.1 μM) for 24 h. Cells were collected in centrifuge tubes and washed twice with pre-cooled PBS. PBS was centrifuged and discarded. Cells were suspended in 200 mL of 1 binding buffer. 5 mL Annexin V-FITC and 5 mL of propidium iodide (BD Biosciences, San Jose, CA, USA) were then sequentially added to the solution. Apoptosis was rapidly detected by flow cytometry (BD FACS Cal ibur). Finally, cells were analyzed by flow cytometry.
2.8 Animals feeding
Male SPF Sprague-Dawley (SD) rats, 6 weeks old, 150–180 g, were purchased from Pengyue Experimental Animal Breeding Co Ltd [Qualification No. SYXK (Lu) 2018–0029, Jinan]. Animals were kept in animal houses at standard temperature (20 ± 1.0)°C, humidity (50 % ± 10 %) and 12 h photoperiod, given a standard diet, fed and watered ad libitum, and acclimatized for experiments 7 days before. All experimental-related operations were approved by the Experimental Animal Ethics Committee of Binzhou Medical University. All efforts were made to minimize the suffering of the animals used for the present experiments.
2.9 AIA induction and treatment
Rats were randomly separated into 6 different experimental groups with which 8 rats per group. Except for the control group, complete Freund's adjuvant (CFA) induced inflammation in rats by injecting 100 μL in the left hind paw after mixing in a vortex shake. To establish a model of adjuvant-induced rheumatoid arthritis (AIA), control rats were injected with an equal volume of saline at the same site. On day 10 after AIA induction, the control and AIA groups received intraperitoneal treatment Saline was used daily; the MTX group was treated orally. weekly with MTX (7.6 mg/kg suspended in 0.5 % CMC-Na) once a week; and the 4k group was treated intraperitoneally.
2.10 Paw swelling and weight measurement
Body weight and paw swelling were measured in rats prior to modeling and every three days thereafter, and the arthritis index was evaluated. Paw swelling = post-inflammatory volume - pre-inflammatory volume. Grade 0: normal, no redness or swelling; Grade 1: redness or swelling of the toe joint; Grade 2: redness or swelling of the toe joint and metatarsal region; Grade 3: redness or swelling below the ankle joint; Grade 4: redness or swelling of the toe and ankle joints and joint dysfunction.
2.11 Arthritis index
On day 24, 2 h after the last dose, the rats were weighed and anesthetized (sodium pentobarbital; 50 mg/kg; i.p). The spleen and thymus of the rat were removed and euthanized. The spleen and thymus were weighed wet on an electronic balance in thousands to calculate the spleen and thymus factor. Immune organ index (%) = immune organ weight (mg) / rat body weight (g).
2.12 Immune index testing and blood processing
On day 24, 1 h after the last dose, the rats were given an intraperitoneal injection of 10 % chloral hydrate (1.15 mL/300 g) and blood was taken from the abdominal aorta after anesthesia, the blood samples were collected and left for two hours before centrifugation (3000 rpm, 4 °C, 10 min) and the supernatant was frozen at −80 °C. The spleen was taken and weighed wet on a one-thousandth of an electronic balance to calculate the spleen factor. Immune organ index (%) = immune organ weight (mg)/rat body weight (g).
2.13 Histopathological examination of joints
Rat ankle sections were entrusted to Rafael Biotechnology Co., Ltd. The left hind paw of rats was peeled, fixed in 4 % paraformaldehyde for 72 h, and decalcified by 12 % EDTA washing for 28 days. The samples were then routinely dehydrated, embedded, and cut into 4-μm paraffin sections, followed by HE staining and visualization by light microscopy. The degree of inflammatory cell infiltration was classified into four grades after pathological evaluation: grade 0: no inflammatory cell infiltration; grade 0: no inflammatory cell infiltration; grade; 1: weak inflammatory cell infiltration; grade; 2: mild infiltration; grade; 3: moderate inflammatory cell infiltration; and grade; 4: massive inflammatory cell infiltration. Synovial cell hyperplasia was classified into three grades, grade 0: normal without hyperplasia; grade 1: essentially normal with a small amount of hyperplasia; grade 2: moderate hyperplasia; and grade 3: significant synovial cell hyperplasia with massive synovial cell attachment.
2.14 Statistics analysis
GraphPad Prism 8 software was used for plotting and bilateral t-tests, and ImageJ software was used to quantify protein expression. ##p < 0.01, ###p < 0.001 and *p < 0.05, **p < 0.01, ***p < 0.001 indicate statistically significant differences.
3 Results and discussion
3.1 Synthesis and structural characterization of target compounds
The synthetic strategy and structures of BAPs are shown in Scheme 1. One of the key raw materials is N-methyl-4-pperidone. According to the previous design, the aromatic aldehyde on one side is chosen as the raw material of 3-pyridine carboxaldehyde (1a) and 4-pyridine carboxaldehyde (1b), while the aromatic aldehyde on the other side was chosen to contain single fluorine, single trifluoromethyl or multiple substituents, such as 4-fluoro benzaldehyde (2b), 3-(trifluoromethyl)benzaldehyde (2c), 2,3-difluorobenzaldehyde (2d), 3,5-bis(trifluoromethyl)benzaldehyde (2k), 2-fluoro-3-(trifluoromethyl)benzaldehyde (2l), and so on. The titled compounds, 4a-s, and 5a-s, were synthesized under Claisen-Schmidt condensation catalyzed by dry HCl gas (Li et al., 2018a, 2018b). In the synthesis process, the ratio of the three raw materials was 1: 1: 1. Because of the formation of symmetric and asymmetric compounds, the target BAPs were purified by column chromatography, and the yield was only about 30 %. These yield rates are also similar to those reported in the literature. Then their chemical structures were confirmed by 1H NMR, 13C NMR and HRMS.Synthetic route of compounds 4a-s and 5a-s. Reagents and conditions: (a) 20 % NaOH/MeOH, T: 25 °C, 3–5 h.
The spectral characteristics of the title compounds BAPs (4a-s and 5a-s) share some common features because of their similar skeleton of (3E,5E)-3,5-bis(arylidene)-4-piperidone. In this study, BAP 4b was taken as an example (Fig. 2). For the 1H NMR spectra, the chemical shifts in 7.61 ppm and 7.62 ppm appear as two single peaks attributed to the two protons of the dissymmetrical α,β-unsaturated ketone pharmacophore on both sides of central piperidone. Meanwhile, due to the dissymmetry of BAP 4b, 1H NMR signals of two methylene groups of piperidone split into 3.75 ppm and 3.73 ppm. Similarly, in 13C NMR spectra, the two methylene carbons split into two carbon signals, such as 56.74 ppm and 56.61 ppm. In addition, the characteristic peak with a chemical shift in 186.70 ppm should correspond to the carbonyl carbon atom of piperidone. BAP 4b contains 4-fluorobenzene substitution, resulting in the presence of four split carbon signals in the carbon spectrum, at 162.87 ppm (d, J = 246.1 Hz), 133.75 ppm (d, J = 2.0 Hz), 133.38 ppm (d, J = 8.6 Hz), and 116.28 (d, J = 21.3 Hz). In conclusion, the above NMR signals prove the structural correctness of BAP 4b. These results further confirm the correctness of their structures.(A) 1HNMR spectrum and proton attribution of 4b; (B) 13CNMR spectrum and carbon attribution of 4b.
3.2 Screening for cytotoxic and anti-inflammatory activity
In this study, the CCK-8 assay was used as a preliminary cytotoxicity assessment in vitro. All experiments were repeated three times in parallel and the mean value of survival was taken. The survival rates of RAW264.7 cells treated with the title compounds (1.1 μM) are shown in Fig. 3. After treatment with 1.1 μM of BAPs, the survival rate of RAW264.7 cells was more than 85 %, which proved the newly synthesized BAPs were not significantly toxic to RAW264.7 cells. Only compounds 4b, 4e, 5k, and 5n were more toxic to RAW264.7 cells, resulting in only 85–90 % cell survival, but this does not affect the screening for the next anti-inflammatory activity.(A) Survival rate (%) of RAW 264.7 cells treated with target compounds (5.0 μM). (B) Expression rate (%) of the inflammatory cytokine TNF-α in LPS-stimulated RAW264.7 macrophages with target compounds (5.0 μM) and LPS (1 μg/mL) by ELISA analysis. PDTC (5.0 μg/mL) was used as a positive control.
In RA, TNF-α and IL-6 are the main pro-inflammatory cytokines that trigger bone damage. TNF-α and IL-6 can cause bone damage by binding to receptors and indirectly inducing the expression of RANKL (Marahleh et al., 2019; Labib et al., 2020). Therefore, blocking TNF-α or IL-6 delays or halts the progression of bone destruction in RA. The inflammation model established by LPS-stimulated RAW 264.7 cell is used in basic research of anti-inflammatory drugs, which can help to screen drug targets and anti-inflammatory active ingredients and contribute to the development of anti-inflammatory drugs. PDTC (Pyrrolidine dithiocarbamate, μM) was used as a positive control. All experiments were performed three parallel times and the mean expression rates (%) are shown in Fig. 3. We see that the TNF-α expression rate in LPS-stimulated RAW 264.7 cell after PDTC (5.0 μM) exposure was 90.3 ± 1.5 %.
For the monofluorinated substituted BAPs 4a, 4b, 5a, and 5b, they had little to no inhibitory effect on the release of TNF-α, which expression rates were all higher than the positive control PDTC. For bis-fluorinated substituted 4d-i and 5d-i, 2,5-F-3-pyridyl-substituted 4g and 2,6-F-3-pyridyl-substituted 4h exhibited better anti-inflammatory activity, and the TNF-α expression rate in LPS-stimulated RAW 264.7 cell after treatment with 4g and 4h were only 67.7 % and 68.5 %, respectively. More interestingly, the 4-pyridyl-substituted 5g also exhibited an expression rate of 81.2 %.
After changing the substituent to 3-CF3-substituted BAP 4c and 4d, this inhibitory effect was significantly improved, leading to a release of TNF-α of 82.2 % and 82.9 %, respectively. There is no difference in inhibitory effect whether 3-pyridyl or 4-pyridyl substituted in BAPs. For 2,4-CF3-substituted 4j and 5j, the inhibitory effect barely improves. When replacing the substituent position with 3,5-CF3, BAPs 4k and 5k were obtained. Surprisingly, they showed a significant improvement in anti-inflammatory activity, whose TNF-α expression rate in LPS-stimulated RAW 264.7 cells can reach 64.8 % and 78.5 %, respectively. Especially for 4k, exhibited the strongest inhibitory effect against TNF-α expression in all title BAPs. This indicates that 3-pyridine substitution is more favorable than 4-pyridine substitution in anti-inflammatory activity. In general, the results indicated that the substituent effect of BAPs has a significant influence on TNF-α expression in LPS-stimulated RAW 264.7 cells.
To further examine the substituent effect of BAPs, we selected a batch of aromatic aldehydes containing -F and -CF3 groups as raw materials to obtain a series of fluorine- and trifluoromethyl-substituted BAPs, 4l–s, and 5l–s. Screening for anti-inflammatory activity showed 2-F-3-CF3-substituted 4l, 2-F-4-CF3-substituted 4m, 2-F-5-CF3-substituted 5n, 2-F-6-CF3-substituted 5o, and 4-F-2-CF3-substituted 4r exhibited moderate inhibitory effect against TNF-α expression. Their expression rate in LPS-stimulated RAW 264.7 cells was 71.9 %, 78.2 %, 79.9 %, 71.4 %, and 78.5 %, respectively. Compared to that of 4k, there is no significant improvement.
Based on the above results, the preliminary structure–activity relationship (SAR) of anti-inflammatory activities could be summarized. Firstly, mono-fluoro-substituted BAPs are less active than monotrifluoromethyl-substituted BAPs. Secondly, the 3,5-CF3 substituent significantly outperformed other substituents. Thirdly, 3-pyridine substituents significantly outperformed 4-pyridine substituents. Combining toxicity and anti-inflammatory activity, 4k (Fig. 4A) was selected to continue the next biological evaluation.(A) The structure of BAP 4k. (B) The expression levels of IL-6 in LPS-induced RAW 264.7 cells through ELISA analysis. PDTC and BAP 32 were used as positive controls. Statistical significance relative to the LPS group is indicated: *: P < 0.1; **: P < 0.01. Compared with Ctrl group: ###: P < 0.001. (C) RAW 264.7 cells were incubated with 4k and analyzed for apoptosis by flow cytometry.
The anti-IL-6 effect is shown in Fig. 4, where IL-6 expression decreased to 72 % after PDTC (5 μM) exposure. Using BAP 32 (1.1 μM), a lead compound with good anti-inflammatory activity, as a control, the expression rate decreased to 65 % after exposure, which was lower than PDTC. BAP 4k expression rate decreased to 59 % after exposure, which was better than PDTC with BAP 32. The present results show that 4k can effectively reduce the concentration of TNF-α and IL-6 and have a good anti-inflammatory effect.
3.3 4k induce RAW264.7 cells apoptosis in a dose-dependent manner
To further corroborate the effect of 4k on apoptosis, the results of 4k on RAW264.7 cells were analyzed by flow cytometry using the Membrane Associated Protein V/PI assay kit. Double staining for membrane-linked protein V and PI allows differentiation between live cells (membrane-linked protein V-/PI-), early apoptotic cells (annexin V+/PI-), late apoptotic cells (annexin V+/PI + ), and necrotic cells (membrane linked protein V-/PI + ). As shown in Fig. 4C, there was almost no apoptosis of Ctrl cells, while apoptosis only was 1.5 %, 4.9 %, and 6.8 %, after treatment with different doses of 4k (0.275, 0.55, 1.1 μM, respectively). Apoptosis increased with increasing dose. The present results further corroborate that 4k (1.1 μM) was not significantly toxic to RAW264.7 cells.
3.4 4k impacts paw swelling, arthritic index values, and body weight in AIA rats
NF-κB and MAPK signaling pathways are both classical inflammatory pathways, which can promote the proliferation and differentiation of osteoclasts, thus causing bone erosion and bone destruction (Jiang et al., 2019; Behl et al., 2022). Therefore, NF-κB and MAPK signaling pathways play an important role in the disease development of RA, which are important targets for the treatment of RA and other chronic immune-mediated inflammatory diseases. Normally, inactivated NF-κB is inactivated in the cytoplasm, while inflammatory stimuli (LPS or other external stimuli) lead to the release and nuclear translocation of NF-κB and phosphorylation of the p65-IκBα complex (Zhang et al., 2018; Liu et al., 2020; Zhou et al., 2022; Yu et al., 2020). MAPK signaling pathway is closely related to NF-κB. So, we endeavored to reveal whether the inhibitory effect of compound 4k on inflammation is related to the activation of NF-κB and MAPK. Therefore, we further detected the expression of NF-κB-related and MAPK-related proteins, including IκB-α, p65, JNK, ERK, and p38. Analysis of IκB-α, p65 in LPS-treated RAW264.7 cells by protein blotting revealed (Fig. 5) that 24 h LPS intervention induced IκB-α, p65 protein phosphorylation and upregulated JNK, ERK, p38 protein phosphorylation compared to control. After different concentrations (0.275, 0.55, and 1.1 μM) of 4k, the LPS-induced protein phosphorylation expression levels decreased significantly as expected. As shown in Fig. 5A, compared with the LPS-induced group, the expression levels of NF-κB-related IκB-α and p65 proteins were significantly decreased and exhibited a clearly dose-dependent (Fig. 5B and 5C). As shown in Fig. 5D, the expression levels of MAPK-related JNK, ERK, and p38 proteins were significantly decreased sequentially with increasing concentrations of 4k. A clearly dose-dependent can be found (Fig. 5E-G). The result indicated that 4k significantly inhibited the LPS-induced phosphorylation of NF-κB-related IκB-α, p65 proteins, and MAPK-related JNK, ERK, and p38 proteins. This also indicates that 4k may inhibit LPS-induced NF-κB and MAPK activation with a dose-dependent in RAW264.7 cells.(A) The expression of NF-κB-related IκBα and p65 phosphorylation in LPS-induced RAW264.7 cells by 4k; (B-C) Relative expression of p-IκBα and IκBα, p-p65 and p65; (D) The expression of MAPK-related JNK, ERK, p38 proteins and their phosphorylation in LPS-induced RAW264.7 cells by 4k; (E-G) Relative expression of p-ERK and ERK, p-JNK and JNK, p-p38 and p38; Compared with LPS alone group: *: P < 0.05; **: P < 0.01; ***: P < 0.001. Compared with Ctrl group: ###: P < 0.001.
3.5 4k impacts paw swelling, arthritic index values, and body weight in AIA rats
The development of RA is characterized by joint erythema, pain, and synovial hyperplasia. The adjuvant-based arthritis model is a classic model of RA in which rats develop swelling and pain, synovial hyperplasia, and bone damage after inflammation is induced by using a complete Freund's adjuvant (Choudhary et al., 2018; Li et al., 2021). Compound 4k showed favorable results in the assay of the LPS-induced anti-inflammatory activity of RAW264.7. To further determine the anti-RA efficacy of 4k, this study was conducted to establish an adjuvant-type arthritis model using complete Fever's adjuvant as an inducer for efficacy studies (Fig. 6A). In this study, the CFA was established with a significant increase in paw swelling and arthritis index (Logashina et al., 2021). Therefore, paw swelling and arthritis indices are important indicators of inflammation measurements. Firstly, paw volumes of all rats were measured every 3 days, starting from day 0 (Fig. 6B). The methotrexate (MTX) and 4k (10 mg/kg) groups inhibited foot swelling in rats from 15 days compared with the AIA. However, by 24 days, the 4k group (10 mg/kg) paw swelling was significantly lower than that of the AIA group. Secondly, to assess the development of inflammation, we performed a simultaneous assessment of the arthritis coefficient. There was no significant difference in the arthritis scores of the AIA group when the scores were started after administration (Fig. 6C) on day 12. With continuous administration, MTX with 4k (10 mg/kg) showed a significant advantage in the inhibition of paw swelling. Finally, body weight is an important indicator of in vivo experimental toxicity of the compounds assessed, with body weight changes able to give an important indication of toxicity to the rats. Body weight measurements were performed every three days starting from day 10 (Fig. 6D). Compared with the AIA and control groups, the MTX group showed a significant decrease in body weight, which may be related to the toxicity of MTX, but there was no significant effect on body weight after injection of 4k.(A) Experimental process of the adjuvant-based arthritis model. (B-D) Degree of paw swelling, arthritis index, and body weight changes in rats. B: Paw swelling; C: Arthritis index; D: Body weight change; Data are represented as mean ± SEM, n = 5. ##p < 0.01, ###p < 0.001 compare with control group.
3.6 4k alters AIA-associated histopathological changes in rat joints
During the development of CFA, ankle synovial hyperplasia and inflammatory cell infiltration are important indicators for evaluating the condition. Therefore, we decided to observe the ankle joint of rats by HE staining. First, Fig. 7A shows no exudate in the joint cavity of the control group and no inflammatory cell infiltration in the connective tissue of the joint capsule. The surface was covered with a single or double layer of synovial cells without hyperplasia. Unlike the control group, the AIA group had a large amount of inflammatory cell infiltration and cellular degeneration in the joint capsule connective tissue (black arrows), and the surface was covered with synovial cell hyperplasia (blue arrows). The MTX group has an insignificant inflammatory cell infiltrate with mild surface synovial cell hyperplasia with inflammatory exudate (red arrow). The 4k group has synovial hyperplasia with a small amount of inflammatory cell infiltration. Secondly, histopathology showed that inflammatory cell infiltration and synovial cell proliferation were significantly reduced in the 4k group in a dose-dependent manner (Fig. 7B). The results indicated that 4k effectively inhibited synovial proliferation and inflammatory cell infiltration in rat ankle joints and suppressed CFA inflammation.Effect of 4k on the histopathology of rat joints. (A) Haematoxylin and eosin-stained sections of joint tissues; (B) inflammatory scores of joint tissues. (C, D) The impact of 4k on immune organ Spleen (C) and thymus (D) index values in AIA Model Rats. Data are represented as mean ± SD, n = 3. ##p < 0.01, ###p < 0.001 compared with control group, *p < 0.1, **p < 0.01, ***p < 0.001 compared with AIA group.
3.7 The impact of 4k on immune organ index values in AIA model rats
Significantly increased splenic index values in AIA relative to control, animals at the end of the experimental period are consistent with the immunological basis of the disease (Zeng et al., 2023). Relative to AIA model animals, MTX-treated rats exhibited a significant decrease in immune organ index values consistent with impaired immune function in treated animals. The dose of 4k (10 mg/kg) slightly reduced thymic index values (Fig. 7D), but did not affect splenic index values (Fig. 7C).
4 Conclusions
Rheumatoid arthritis (RA) is a peripheral immune disease, whereas the usual clinical drugs are limited to NSAIDs and immunosuppressive drugs. Inhibition of inflammation-related NF-κB and MAPK activation could treat RA to some extent. The natural product curcumin and its analogs have been extensively studied for their anti-inflammatory and anti-cancer activity, including anti-RA effects. Recently, mang 3,5-bis(aryl)-4-piperidone derivatives (BAPs) were obtained by design modification of curcumin in our group, and some fluorine- and pyridyl-substituted BAPs exhibited promising potential anti-inflammatory activity. In this paper, thirty-eight dissymmetric fluorine and pyridyl-substituted BAPs were synthesized and evaluated as potential anti-inflammatory agents for the treatment of RA by inhibition of MAPK and NF-κB signaling pathways in this study. It is hoped that our study will yield potentially versatile agents for the treatment of inflammatory diseases such as RA.
A preliminary structure–activity relationship was summarized and discovered 3,5-CF3 and 3-pyridyl-substituted 4k exhibited less toxicity and more potential anti-inflammatory activity. We also found that anti-rheumatoid effects were related to the inhibition of NF-κB and MAPK signaling pathways. Mechanistic studies show that lead compound 4k effectively inhibited LPS-induced NF-κB and MAPK activation by suppressing LPS-induced phosphorylation levels of p65, IκBα, JNK, ERK, and p38 in RAW264.7 cells. Based on the rat AIA model, 4k significantly inhibited the growth of paw swelling and arthritis index in rats. More importantly, 4k did not affect body weight and splenic index values, which indicates that it had a better therapeutic effect and no significant toxicity in CFA rats. Histopathologically, 4k effectively inhibited synovial hyperplasia and inflammatory cell infiltration in rat ankle joints and suppress the development of CFA rats. Overall, this study demonstrated that curcumin analog, BAP 4k, had relatively potential anti-RA effects. It may be developed as a potential multifunctional agent for the clinical treatment of inflammatory diseases such as RA.
Funding
We are grateful for financial support from Shandong Provincial Natural Science Foundation (Nos. ZR2022MH159, ZR2023MH022, ZR2023MH190), College Youth Innovation Science and Technology Support Programme of Shandong province (No. 2020KJK003). This study was financially supported by Key Laboratory of Medical Antibacterial Materials of Shandong Province.
Declaration of competing interest
The authors declare that they have no known competing financial interests or personal relationships that could have appeared to influence the work reported in this paper.
References
- Biological evaluation, docking studies, and in silico ADME prediction of some pyrimidine and pyridine derivatives as potential EGFRWT and EGFRT790M inhibitors. J. Enzyme Inhib. Med. Chem.. 2023;38(1):176-191.
- [CrossRef] [Google Scholar]
- Polyphenols targeting MAPK mediated oxidative stress and inflammation in rheumatoid arthritis. Molecules. 2021;26(21):6570.
- [CrossRef] [Google Scholar]
- Polyphenols inhibiting MAPK signalling pathway mediated oxidative stress and inflammation in depression. Biomed. Pharmacother.. 2022;146:112545
- [CrossRef] [Google Scholar]
- Duality of fibroblast-like synoviocytes in RA: passive responders and imprinted aggressors. J. Nat. Med.. 2013;9(1):24-33.
- [CrossRef] [Google Scholar]
- Phillyrin ameliorated collagen-induced arthritis through inhibition of NF-κB and MAPKs pathways in fibroblast-like synoviocytes. Arab. J. Chem.. 2023;16:104844
- [CrossRef] [Google Scholar]
- Experimental animal models for rheumatoid arthritis. Immunopharmacol. Immunotoxicol.. 2018;40:193-200.
- [CrossRef] [Google Scholar]
- Trifluoromethyl-substituted 3,5-bis(arylidene)-4-piperidones as potential anti-hepatoma and anti-inflammation agents by inhibiting NF-кB activation. J. Enzyme Inhib. Med. Chem.. 2021;36(1):1622-1631.
- [CrossRef] [Google Scholar]
- Synthesis and target identification of benzoxepane derivatives as potential anti-neuroinflammatory agents for ischemic stroke. Angew. Chem. Int. Ed. Engl.. 2020;59(6):2429-2439.
- [CrossRef] [Google Scholar]
- Bioactivities of EF24, a novel curcumin analog: a review. Front. Oncol.. 2018;8:614.
- [CrossRef] [Google Scholar]
- Novel tetrazole-based selective COX-2 inhibitors: design, synthesis, anti-inflammatory activity, evaluation of PGE2, TNF-α, IL-6 and histopathological study. Bioorg. Chem.. 2020;104:104308
- [CrossRef] [Google Scholar]
- Rosmanol and carnosol synergistically alleviate rheumatoid arthritis through inhibiting TLR4/NF-κB/MAPK pathway. Molecules. 2021;27:78.
- [CrossRef] [Google Scholar]
- N-phenylsulfonyl-3,5-bis(arylidene)-4-piperidone derivatives as activation NF-κB inhibitors in hepatic carcinoma cell lines. Eur. J. Med. Chem.. 2018;155:531-544.
- [CrossRef] [Google Scholar]
- Novel dissymmetric 3,5-bis(arylidene)-4-piperidones as potential antitumor agents with biological evaluation in vitro and in vivo. Eur. J. Med. Chem.. 2018;147:21-33.
- [CrossRef] [Google Scholar]
- Early diagnosis and treatment of rheumatoid arthritis. Prim. Care. 2018;45(2):237-255.
- [CrossRef] [Google Scholar]
- Discovery of talmapimod analogues as polypharmacological anti-inflammatory agents. J. Enzyme Inhib. Med. Chem.. 2020;35:187-198.
- [CrossRef] [Google Scholar]
- Anti-Inflammatory and analgesic effects of TRPV1 polypeptide modulator APHC3 in models of osteo- and rheumatoid arthritis. Mar. Drugs. 2021;19:39.
- [CrossRef] [Google Scholar]
- TNF-α directly enhances osteocyte RANKL expression and promotes osteoclast formation. Front. Immunol.. 2019;10:2925.
- [CrossRef] [Google Scholar]
- Fluorine and fluorinated motifs in the design and application of bioisosteres for drug design. J. Med. Chem.. 2018;61(14):5822-5880.
- [CrossRef] [Google Scholar]
- Circular dichroism calculation for natural products. J. Nat. Med.. 2014;68:1-10.
- [CrossRef] [Google Scholar]
- Curcumin and rheumatoid arthritis: A systematic review of literature. Int. J. Clin. Pract.. 2021;75(10):e14280.
- [Google Scholar]
- Synthesis, crystal structures and anti-inflammatory activity of fluorine-substituted 1,4,5,6-tetrahydrobenzo[h]quinazolin-2-amine derivatives. Acta Crystallogr. C Struct. Chem.. 2019;75(Pt 8):1157-1165.
- [CrossRef] [Google Scholar]
- Potential anti-neuroinflammatory NF-кB inhibitors based on 3,4-dihydronaphthalen-1(2H)-one derivatives. J. Enzyme Inhib. Med. Chem.. 2020;35(1):1631-1640.
- [CrossRef] [Google Scholar]
- Curcumin attenuates collagen-induced rat arthritis via anti-inflammatory and apoptotic effects. Int. Immunopharmacol.. 2019;72:292-300.
- [CrossRef] [Google Scholar]
- Systemic pharmacological verification of Baixianfeng decoction regulating TNF-PI3K-Akt-NF-κB pathway in treating rheumatoid arthritis. Bioorg. Chem.. 2022;119:105519
- [CrossRef] [Google Scholar]
- Dissymmetric pyridyl-substituted 3,5-bis(arylidene)-4-piperidones as anti-hepatoma agents by inhibiting NF-κB pathway activation. Eur. J. Med. Chem.. 2019;167:187-199.
- [CrossRef] [Google Scholar]
- Targeting NF-κB pathway for the therapy of diseases: mechanism and clinical study. Signal Transduct. Target. Ther.. 2020;5:209.
- [CrossRef] [Google Scholar]
- The Effective Treatment of Purpurin on Inflammation and Adjuvant-Induced Arthritis. Molecules. 2023;28:366.
- [CrossRef] [Google Scholar]
- Discovery of novel small-molecule inhibitors of NF-κB signaling with antiinflammatory and anticancer properties. J. Med. Chem.. 2018;61(14):5881.
- [CrossRef] [Google Scholar]
- Aflatoxin B1 induces microglia cells apoptosis mediated by oxidative stress through NF-κB signaling pathway in mice spinal cords. Environ. Toxicol. Pharmacol.. 2022;90:103794
- [CrossRef] [Google Scholar]
- Synthesis and optimization of novel allylated mono-carbonyl analogs of curcumin (MACs) act as potent anti-inflammatory agents against LPS-induced acute lung injury (ALI) in rats. Eur. J. Med. Chem.. 2016;121:181-193.
- [CrossRef] [Google Scholar]