Translate this page into:
Binary and ternary approach of solubility of Rivaroxaban for preparation of developed nano drug using supercritical fluid
⁎Corresponding author. esfandiari_n@miau.ac.ir (Nadia Esfandiari)
-
Received: ,
Accepted: ,
This article was originally published by Elsevier and was migrated to Scientific Scholar after the change of Publisher.
Abstract
This study addressed the solubility of Rivaroxaban in supercritical carbon dioxide at a temperature range of 308–338 K and a pressure range of 12–30 MPa with and without a Co-solvent in binary and ternary systems. The impact of ethanol Co-solvent was also examined. Furthermore, the examined systems were modeled using semi-empirical approaches once the tentative solubility data were determined. Rivaroxaban solubility in the binary and ternary systems ranged based on mole fraction from to and to , respectively. Based on the results, the use of a Co-solvent can greatly boost the solubility of Rivaroxaban. The highest Co-solvent effect on the Rivaroxaban-Ethanol-CO2 mixture was observed at 18.73 (338 K and 12 MPa). Furthermore, empirical and semi-empirical models can effectively fit the solubility values of the analyzed materials by AARD% and Radj for binary and ternary approaches. The Jouyban et al. (AARD%=7.40 and Radj = 0.993) model for the binary system and the Garlapati-Madras (AARD%=6.16 and Radj = 0.991) and Sodeifian-Sajadian (AARD%=6.13 and Radj = 0.979) and Soltani-Mazloumi (AARD%=6.89 and Radj = 0.987) models for the ternary system are the most accurate models.
Keywords
Rivaroxaban
Solubility
Supercritical carbon dioxide
Semi-empirical modeling
Co-solvent
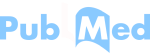
Nomenclature
- a0-a6
-
Adjustable parameters for density-based models
- AARD%
-
Average absolute relative deviation
- Cs
-
Solute concentration in the collection vial
- e
-
Co-solvent enhancement effects
- MCO2
-
CO2 molecular weight (g/mol)
- Ms
-
Solute molecular weight (g/mol)
- MW
-
Molecular weight (g/mol)
- N
-
The number of experimental data, dimensionless
- nCO2
-
Mole of CO2
- nsolute
-
Moles of solute (RXN)
- P
-
Pressure (MPa)
- Pc
-
Critical pressure (MPa)
- Pref
-
Reference pressure (0.1 MPa)
- Q
-
The number of self-determining parameters
- R2
-
Correlation coefficient
- Radj
-
Adjusted correlation coefficient
- S
-
Equilibrium solubility (g/L)
- SSE
-
Sum square error
- SST
-
Total sum of squares
- T
-
Temperature (K)
- Tc
-
Critical temperature (K)
- Tm
-
Melting temperature (K)
- y2
-
Equilibrium mole fraction
-
Mole fraction in ternary system
- y3
-
Mole fraction of Co-solvent
- Vs
-
Volume of the collection vial (L)
- VL
-
Volume of the sampling loop (L)
- Z
-
Number of adjustable parameters
- Cal
-
Calculated
- Exp
-
Experimental
- i, j
-
Component
Superscript
- 2
-
Solute
- i, j
-
Component
Subscripts
- ASES
-
Aerosol solvent extraction system
- BCS
-
Biopharmaceutics Classification System
- cEoS
-
Cubic equations of state
- DMSO
-
Dimethyl sulfoxide
- EC number
-
European Community number
- EoS
-
Equations of State
- GAS
-
Supercritical gas antisolvent
- GRAS
-
Generally Recognized as Safe
- GUM
-
Guide of uncertainty measurement
- HBA
-
Hydrogen-bond acceptor
- HBD
-
Hydrogen-bond donor
- HSP
-
Hansen solubility parameter
- KJ
-
Kumar and Johnston model
- KT
-
Kamlet-Taft solvent parameters
- LFHB
-
Lattice Fluid Hydrogen Bonding
- PGSS
-
Particles from the gas saturated solution
- PC-SAFT
-
Perturbed-chain SAFT
- PCP-SAFT
-
Perturbed-chain polar SAFT
- PR
-
Peng-Robinson
- MST
-
Méndez-Santiago and Teja model
- RESS
-
Rapid expansion of the supercritical solution
- RESOLV
-
Rapid expansion of a supercritical solution into a liquid solvent
- RESSAS
-
Rapid expansion of supercritical solution into aqueous solutions
- RV
-
Retrograde vaporization
- RXN
-
Rivaroxaban
- SA
-
Simulated annealing
- SAS
-
Supercritical antisolvent
- SCF
-
Supercritical fluid
- SC-CO2
-
Supercritical CO2
- SEDS
-
Solution-enhanced dispersion by supercritical fluid
- SRK
-
Soave-Redlich-Kowng
Abbreviations
- α
-
H-bond donor
- β
-
H-bond acceptor
- π*
-
Kamlet-Taft dipolarity/polarizability
- δ
-
Hildebrand solubility parameter
- λmax
-
Maxim wave length (nm)
-
Density of SC-CO2 (kg m−3)
-
Reference density (700 kg m−3)
Greek symbols
1 Introduction
Rivaroxaban (RXN) is the first authorized oral direct factor Xa inhibitor (xabans) and a direct oral anticoagulant. Inhibiting Factor Xa diminishes the activation of coagulation and platelets. RXN can be used to minimize the risk of coronary heart disease and embolism individuals with nonvalvular atrial fibrillation, to prevent and/or treat venous thromboembolism, and to treat bioprosthetic mitral valves. It has emerged as an acceptable alternative to vitamin K antagonists, which are more susceptible to drug-drug interactions and more complicated to administer. However, RXN has an inherent risk of bleeding and can increase the risk of hemorrhage when used with other hemostasis-weakening medications. It is not recommended in pregnant or lactating women, children, or those with severe hepatic (ChildPugh C), renal, antiphospholipid syndrome, or artificial heart valves (Kubitza et al., 2010, Patel et al., 2011, Samama et al., 2013, Thomas et al., 2013, Costa et al., 2020, Duarte et al., 2020, Evans et al., 2020, Fernandez et al., 2021, Galiuto and Patrono, 2021). Capell et al. discovered that RXN improved the incidence of thrombotic events, hospitalizations, and deaths among symptomatic outpatients with COVID-19 (Capell et al., 2021).
RXN is categorized as a high-permeability and low-solubility substance by the Biopharmaceutical Classification System (BCS) (Class II) (Mueck et al., 2014, Kushwah et al., 2021). It exhibits low pH-independent solubility in aqueous solution. Xarelto is the commercial brand of RXN, and 685–132-2 is the and EC number (European Community) of RXN, respectively (Seshamamba and Sekaran, 2017, Kushwah et al., 2021).
The bioavailability of drugs is limited by their solubility in aqueous media, which is governed by their dissolution time. Reducing the particle size of drugs that are normally water-insoluble is a typical strategy for enhancing their solubility and dissolution rate (Esfandiari, 2015, Esfandiari and Ghoreishi, 2015a,b, Sodeifian et al., 2020a, Esfandiari and Sajadian, 2022a). Supercritical carbon dioxide (SC-CO2)-based particle production technology is a cutting-edge method for creating nano-sized pharmaceuticals. The rapid mass transfer rate and superior dissolving capability of supercritical fluids can be assigned attributed to their viscosities that are more similar to those of gases rather than liquids. Additionally, SC-CO2 is harmless, colorless, odorless, and leaves no residue in the finished product, further promoting its extensive application in the paramedical industry (Cheng et al., 2018, Ardestani et al., 2020, MacEachern et al., 2020, Pishnamazi et al., 2020a,b, Sodeifian et al., 2020f, Zabihi et al., 2020a, Pishnamazi et al., 2021a). A supercritical fluid (SCF) is frequently used as a dense solvent or anti-solvent to manufacture therapeutic nanoparticles. The solubility of the medicine in the solvent is one of the prerequisites for employing supercritical technology. In general, SCF can be utilized in particle production processes through three different approaches: (i) SCF as a solvent, such as RESS, RESSAS, and RESOLV; (ii) SCF as an anti-solvent, such as GAS, SAS, SEDS, and ASES; and (iii) SCF as a Co-solvent, such as PGSS and PGSS-drying (Esfandiari and Ghoreishi, 2013, Esfandiari and Ghoreishi, 2014, Esfandiari, 2015, Esfandiari and Ghoreishi, 2015a, Cheng et al., 2018, Sodeifian et al., 2019d, MacEachern et al., 2020, Pishnamazi et al., 2020c,b, Najafi et al., 2021, Pishnamazi et al., 2021a, Esfandiari and Sajadian, 2022a).
Throughout the last few decades, the estimation of the solubility of medications in SCFs has become one of the main subjects. So far, a few studies have addressed the reliability and correlation of the solubility documentation of different sorts of medications in SCFs. Table 1 sorts the solubility (crossover and mole fraction points of these medicinal compounds in SC-CO2) of certain medications examined in the years between 2017 and the present. The solubility of solid components in SCFs offers fundamental facts on the development of small-scale medicinal particles with the ideal size dispersion, to achieve better dissolution rates (Ardestani et al., 2020, Saadati Ardestani et al., 2020, Askarizadeh et al., 2023). Although numerous experimental techniques can evaluate the solubility of a substance, correlations and mathematical models are frequently implemented to estimate the solubility of the substances in SC-CO2 due to the high cost of experimental measurements. In general, semi-empirical models and equation of state (EoS)-based models can be used to correlate the solubility data. EoS-based models require complex computational techniques and data for a variety of physical variables (cubic equations of state (Peng-Robinson (PR) (Peng and Robinson, 1976) and Soave-Redlich-Kowng (SRK) (Soave, 1972)) or perturbation equations (perturbed-chain polar SAFT (PCP-SAFT) (Gross, 2005), PC-SAFT (Gross and Sadowski, 2001))). Semi-empirical equations, like density-based models, only require easily accessible control items i.e., temperature, pressure, and the density of CO2 with no need for thermophysical properties such as molar volume, acentric factor, and critical point, which cannot be estimated (Sodeifian et al., 2019e, Ardestani et al., 2020, Zhan et al., 2020, Zabihi et al., 2021a). Numerous semi-empirical models have been geared towards connecting the solubility data of solids in SC-CO2; among which, Kumar and Johnston (KJ) (Kumar and Johnston, 1988), Bartle et al. (Bartle et al., 1991), Khansary et al. (Khansary et al., 2015), Jouyban et al. (Jouyban et al., 2002b), Chrastil (Chrastil, 1982), Adachi-Lu (Adachi and Lu, 1983), Garlapati − Madras (Garlapati and Madras, 2010), González et al. (González et al., 2001), Mendez − Santiago − Teja (MST) (Sauceau et al., 2003), Li et al. (Li et al., 2003), Soltani − Mazloumi (Soltani and Mazloumi, 2017), Reddy − Madras (Reddy and Madras, 2011), Keshmiri et al. (Keshmiri et al., 2014), Bian et al. (Bian et al., 2011), Sodeifian et al. (Sodeifian et al., 2019), Sparks et al. (Sparks et al., 2008), Del Valle and Aguilera (Del Valle and Aguilera, 1988), Tan (Yeoh et al., 2013), Gordillo (Gordillo et al., 1999), Yu (Yu et al., 1994), Sung and Shim (Sung and Shim, 1999) can be mentioned. NO: In the pressure range, the crossover is not exit. NO MEN: In the article, the crossover is not calculated.
Compound
Pressure range (MPa)
Temperature range (K)
Cross over (MPa)
Mole fraction (y)
MW (g/mol)
Ref
Esomeprazole (C17H19N3O3S)
12–27
308.2–338.2
22
1.11 × 10-5 to 9.10 × 10-4
345.42
(Sodeifian et al., 2019b)
Amiodarone hydrochloride (C25H29I2NO3. HCl)
12–30
313.2–343.2
19
2.510 × 10−5 to 1.012 × 10−3
681.77
(Sodeifian et al., 2017b)
Ketotifen fumarate (C23H23NO5S)
12–30
308.2–338.2
20
2.11 × 10−5 to 1.07 × 10−3
425.5
(Sodeifian et al., 2018a)
Aprepitant (C23H21F7N4O3)
12–33
308.15–338.15
15–18
4.50 × 10−6 to 7.67 × 10−5
534.4
(Sodeifian et al., 2017a)
Imatinib mesylate (C30H35N7O4S)
12–27
308.2–338.2
18–21
1.0 × 10−7 to 4.4 × 10−6
589.71
(Sodeifian et al., 2019e)
Loratadine (C22H23N2O2Cl)
12–27
308.15–338.15
18–21
4.50 × 10−6 to 1.30 × 10−3
382.88
(Sodeifian et al., 2018b)
Loxoprofen (C15H18O3)
12–40
308–338
20
1.04 × 10−5 to 1.28 × 10−3
246.10
(Zabihi et al., 2020a)
Quetiapine hemifumarate (C21H25N3O2S.0.5C4H4O4)
12–27
308–338
13–14
0.30 × 10−6 to 9.03 × 10−6
441.54
(Sodeifian et al., 2021a)
2,4,7-Triamino-6-phenylpteridine (Triamterene) (C13H13N7)
12–27
308–338
19.2–19.5
0.03 × 10−5 to 2.89 × 10−5
253.26
(Sodeifian et al., 2020a)
Tolmetin (C15H15NO3)
12–40
308–338
16
5.00 × 10−5 to 2.59 × 10−3
257.29
(Pishnamazi et al., 2020c)
Amlodipine besylate (C26H31ClN2O8S)
12–27
308–338
NO
4.15 × 10−6 to 23 × 10−6
567.05
(Sodeifian et al., 2021c)
Busulfan (C6H14O6S2)
12–40
308–338
16
3.27 × 10−5 to 8.65 × 10−4
246.30
(Pishnamazi et al., 2020b)
Sunitinib malate (C26H33FN4O7)
12–27
308–338
NO
0.5 × 10−5 to 8.56 × 10−5
532.56
(Sodeifian et al., 2020c)
Fenoprofen (C15H14O3)
12–40
308–338
16
2.01 × 10−5 to 4.20 × 10−3
242.3
(Zabihi et al., 2020b)
Azathioprine (C9H7N7O2S)
12–27
308–338
12–15
0.27 × 10−5 to 1.83 × 10−5
277.26
(Sodeifian et al., 2020b)
Sorafenib tosylate (C28H24ClF3N4O6S)
12–27
308–338
NO
0.68 × 10−6 to 12.57 × 10−6
637.03
(Sodeifian et al., 2020d)
Capecitabine (C15H22FN3O6)
10–––35
308.15–––348.15
19
3.18 × 10−5 to 120.29 × 10−5
359.35
(Ardestani et al., 2020)
Aspirin (C9H8O4)
10–––30
308.15–––328.15
13–14
0.33 × 10−4 to 3.45 × 10−4
180.15
(Ardestani et al., 2020)
Ibuprofen (C13H18O2)
10–––30
308.15–––333.15
10
0.72 × 10−3 to 3.8 × 10−3
206.28
(Ardestani et al., 2020)
Repaglinide (C27H36N2O4)
12–27
308–338
16–18
2.89 × 10−6 to 9.53 × 10−5
452.29
(Sodeifian et al., 2019d)
Sodium Valproate (C8H15NaO2)
12–27
308.15–––338.15
22–24
0.05 × 10−5 to 3.71 × 10−5
166.19
(Sodeifian et al., 2020f)
Chloroquine (C18H26ClN3)
12–40
308–338
16–20
1.64 × 10−5 to 8.92 × 10−4
319.87
(Pishnamazi et al., 2021a)
Decitabine (C8H12N4O4)
12–40
308–338
16
2.84 × 10–5 to 1.07 × 10–3
228.41
(Pishnamazi et al., 2021b)
Oxcarbazepine (C15H12N2O2)
12–27
308–338
17–19
1.10 × 10-7 to 2.675 × 10-5
252.27
(Sodeifian et al., 2019c)
Sulfabenzamide (C13H12N2O3S)
12–27
308–338
NO
1.53 × 10-6 to 22.35 × 10-6
276.3
(Sodeifian et al., 2021d)
Galantamine (C17H21NO3)
12–27
308–338
17–19
0.006 × 10−4 to 0.233 × 10−4
287.35
(Sodeifian et al., 2021e)
Gliclazide (C15H21N3O3S)
10–18.6
308.2–328.2
15–17
1.26 × 10−7 to 5.01 × 10−6
323.41
(Wang et al., 2021)
Captopril (C9H15NO3S)
10–18.6
308.2–328.2
14–16
3.59 × 10−6 to 9.32 × 10−5
217.28
(Wang et al., 2021)
Salsalate (C14H10O5)
12–40
308–338
16
3.77 × 10−5 to 3.88 × 10−3
258.23
(Zabihi et al., 2021a)
Lansoprazole (C16H14F3N3O2S)
12–27
308.2–338.2
21
1.15 × 10−5 to 7.36 × 10−4
369.36
(Sodeifian et al., 2020g)
(Letrozole) (C17H11N5)
12–36
318.2–348.2
16–18
1.6 × 10−6 to 8.51 × 10−5
263.33
(Sodeifian and Sajadian, 2018)
Rivaroxaban (C19H18ClN3O5S)
12–27
308–338
22.5
0.0104 × 10−4 to 0.2062 × 10−2
435.90
(Sodeifian et al., 2023e)
Tamsulosin (C20H28N2O5S)
12–27
308–338
21
0.18 × 10−6 to 1.013 × 10−5
408.05
(Hazaveie et al., 2020)
Gambogic acid (C38H44O8)
10–30
308.15–328.15
20
1.63 × 10−6 to 22.62 × 10−6
628.76
(Xiang et al., 2019)
Tamoxifen (C26H29NO)
12–40
308–338
20
1.88 × 10−5 to 9.89 × 10−4
371.51
(Pishnamazi et al., 2020a)
Losartan potassium, Cozaar (C22H22ClN6O)
12–27
308–338
19
2.03 × 10−6 to 1.88 × 10−5
461
(Sodeifian et al., 2021f)
Gatifloxacin (C38H50F2N6O11)
12–36
313–333
14
0.106 × 10−6 to 1.605 × 10−6
375.4
(Shi et al., 2017, Padrela et al., 2018)
Enrofloxacin (C19H22FN3O3)
17–36
313–333
17
0.022 × 10−6 to 5.605 × 10−6
359.4
(Shi et al., 2017, Padrela et al., 2018)
Ciprofloxacin (C17H19ClFN3O3)
24–36
313–333
NO
0.0265 × 10−6 to 0.1887 × 10−6
331.34
(Shi et al., 2017, Padrela et al., 2018)
Penicillin G (Benzylpenicillin) (C16H18N2O4S)
10–35
313.15–333.35
10
0.420 × 10−5 to 6.330 × 10−5
334.4
(Gordillo et al., 1999, Padrela et al., 2018)
Lenalidomide (C13H13N3O3)
12–30
308–338
18
0.02 × 10−4 to 1.08 × 10−4
259.25
(Sajadian et al., 2022a)
Glibenclamide (C23H28ClN3O5S)
12–30
308–338
21
0.8 × 10−6 to 8.03 × 10−5
494
Esfandiari and Sajadian, 2022b
Montelukast (C35H36ClNO3S)
12–30
308–338
15
0.4 × 10-6 to 6.12 × 10-5
586.18
(Sajadian et al., 2022c)
Minoxidil (C9H15N5O)
12–27
308–338
19
0.24 × 10−6 to 3.39 × 10−6
209.25
(Sodeifian et al., 2020e)
Ketoconazole (C26H28Cl2N4O4)
12–30
308–338
13–15
0.20 × 10–6 to 8.02 × 10–4
531
(Sodeifian et al., 2021g)
Sertraline. HCl (C17H17Cl2N. HCl)
12–30
308–338
17–19
0.61 × 10−4 to 0.89 × 10−3
342.69
(Sodeifian et al., 2019)
Favipiravir (C5H4FN3O2)
12–30
308–338
18
3.0 × 10-6 to 9.05 × 10-4
157.1
(Sajadian et al., 2022b)
Dasatinib Monohydrate (C22H28ClN7O3S)
12–27
308–338
NO
0.45 × 10−6 to 9.08 × 10−6
505.16
(Sodeifian et al., 2022h)
Clemastine Fumarate (C21H26ClNO·C4H4O4)
12–27
308–338
NO
1.61 × 10–6 to 9.41 × 10–6
460
(Sodeifian et al., 2021b)
Teriflunomide (C12H9F3N2O2)
12–27
308–338
19.5
8.84 × 10–5 to 5.43 × 10–4
270.21
(Sodeifian et al., 2022g)
Metoclopramide hydrochloride (C14H23Cl2N3O2)
12–27
308–338
22
0.15 × 10–5 to 5.56 × 10–5
336.26
(Sodeifian et al., 2022f)
Pholcodine (C23H30N2O4)
12–27
308–338
16–16.5
2.06 × 10–4 to 5.93 × 10−4
398.55
(Sodeifian et al., 2022a)
Lacosamide (C13H18N2O3)
12–30
308–338
12–18
1 × 10−6 to 2.29 × 10−4
250.3
(Esfandiari and Ali Sajadian, 2022)
Febuxostat (C16H16N2O3S)
12–27
308–338
21
0.05 × 10-4 to 7.42 × 10-4
316.37
Abourehab et al., 2022b; Zabihi et al., 2021b
Paracetamol (C8H9NO2)
9.5–26.5
311–358
11
0.305 × 10-6 to 16.358 × 10-6
151.16
(Bagheri et al., 2022)
Methylparaben (C8H8O3)
12–35.5
308–348
15.2
1.13 × 10-5 to 1.213 × 10-3
152.16
(Mahesh and Garlapati, 2022)
Ethylparaben (C9H10O3)
8–21
308–328
8
1.64 × 10-6 to 1.755 × 10-5
166.17
(Mahesh and Garlapati, 2022)
Propylparaben (C10H12O3)
9.41–22.02
308.15––328.15
14
4.4 × 10-6 to 6.12 × 10-5
180.2
(Mahesh and Garlapati, 2022)
Empagliflozin (C23H27ClO7)
12–27
308–338
16.5
5.14 × 10–6 to 25.9 × 10–6
450.91
(Sodeifian et al., 2022c)
Pantoprazole sodium sesquihydrate (C16H14F2N3NaO4S × 1.5 H2O)
12–27
308–338
16
0.0301 × 10–4 to 0.463 × 10–4
432.4
(Sodeifian et al., 2022d)
Prazosin hydrochloride (C19H22ClN5O4)
12–27
308–338
NO
1.59 × 10−5 to 7.2 × 10−5
419.9
(Sodeifian et al., 2022i)
Temozolomide (C6H6N6O2)
12–40
308–338
20
4.30 × 10−4 to 5.28 × 10−3
194.1
(Zabihi et al., 2021b)
Cefuroxime axetil (C20H22N4O10S)
8–25
308–328
Higher than 25 MPa
2.2 × 10−7 to 11.24 × 10-6
510.47
(Ongkasin et al., 2019)
Ethosuximide (C7H11NO2)
9–15
313.15–328.15
NO
3.45 × 10−3 to 8.71 × 10−3
141.168
(Zha et al., 2019)
(Octatrimethylsiloxy) Polyhedral oligomeric silsesquioxanes (POSS) C24H72OO20Si16
1–30
308–328
10.6
0.0083 to 2 × 10-3
1146.18
(Demirtas and Dilek, 2019)
Chlorothiazide (C7H6ClN3O4S2)
13–29
308–338
17
0.417 × 10−5 to 1.012 × 10−5
295.73
(Majrashi et al., 2023)
Pazopanib hydrochloride (C21H24ClN7O2S)
12–27
308–338
NO
1.87 × 10−6 to 14.25 × 10−6
474
(Sodeifian et al., 2022b)
Crizotinib (C21H22Cl2FN5O)
12–27
308–338
14.5
0.156 × 10−5 to 1.219 × 10−5
450.3
(Sodeifian et al., 2022e)
Alendronate (C4H13NO7P2)
12–30
308–338
18
0.01 × 10−4 to 1.5 × 10−4
271.08
Abourehab et al., 2022a
Sildenafil citrate (C22H30N6O4S)
12–30
308–338
15–18
2.40 × 10−7 to 6.48 × 10−6
474.6
(Honarvar et al., 2023)
Riluzole (C8H5F3N2OS)
12–27
308–338
22
4.95 × 10−5 to 1.49 × 10−4
234.2
(Abadian et al., 2022)
Fludrocortisone acetate (C23H31FO6)
12–30
308–338
18–21
0.211 × 10-6 to 0.653 × 10-5
422.5
(Amani et al., 2022)
Metformin (C4H11N5)
14–29
308–328
NO
0.39 × 10-6 to 1.23 × 10-6
129.16
(Venkatesan et al., 2022)
Haloperidol (C21H23ClFNO2)
12–22
313.2–323.2
17–19
3.4 × 10-7 to 1.4 × 10-5
375.9
(Khudaida et al., 2023a)
Retinol Vitamin A (C20H3O)
9–23.3
303–323
11
2.18 × 10-5 to 1.964 × 10-4
286.45
(Naikoo et al., 2021)
Famotidine (FAM) (C8H15N7O2S3)
12–30
308–338
18
1.4 × 10-6 to 1.11 × 10-4
337.43
(Saadati Ardestani et al., 2023)
Erlotinib hydrochloride (C22H24N3O4Cl)
12–30
308–338
19–22
1.2 × 10-6 to 2.12 × 10-5
429.9
(Bazaei et al., 2023)
Phemytoin (C15H12N2O2)
9.5–25
313–345
11
0.68 × 10-6 to 15.7 × 10-6
252.268
(Notej et al., 2023)
Raloxifene (C28H27NO4S)
9.5–25
313–345
12
0.79 × 10-5 to 8.09 × 10-5
473.59
(Notej et al., 2023)
Clonazepam (C15H10ClN3O3)
12–30
308–338
20
3.9 × 10-6 to 7.26 × 10-5
315.71
(Alwi et al., 2023)
Curcumin (C21H20O6)
8–20
308.15–328.15
13
1.82 × 10-8 to 1.97 × 10-6
368.38
(Zhan et al., 2017)
Dibutylbutyl phosphonate (C12H27O3P)
10–25
313–333
11
0.087 to 0.117
250.31
(Pitchaiah et al., 2017)
Diamylamyl phosphonate (C15H33O3P)
10–25
313–333
12
0.065 to 0.09
292.4
(Pitchaiah et al., 2017)
Ipriflavone (C18H16O3)
10–20
308.2–328.2
15
1.4 × 10−4 to 2.2 × 10−4
280.3
(Wang and Su, 2020)
Tolbutamide (C12H18N2O3S)
10–30
313.15–353.15
17–20
1.66 × 10–5 to 40.5
270.35
(Manna and Banchero, 2018)
Chlorpropamide (C10H13N2O3S)
10–30
313.15–353.15
17–20
2.29 × 10–6 to 72.2 × 10–6
276.74
(Manna and Banchero, 2018)
1-aminoanthraquinone (C14H9NO2)
12.5–25
323.15–383.15
17
5.5 × 10–7 to 351 × 10–7
223.23
(Tamura et al., 2017)
1-nitroanthraquinone (C14H7NO)
12.5–25
323.15–383.15
18–20
9.8 × 10–7 to 252.3 × 10–7
253.21
(Tamura et al., 2017)
Phthalocyanines green (Pc-G)
10–35
308.15–338.15
22
0.01 × 10-5 to 12.12 × 10-5
1127.154
(Sodeifian et al., 2019f)
Fampridine (pyridin-4-amine, 4-aminopyridine) (C5H6N2)
10–22
308.2–328.2
10.5–12.5
2 × 10-5 to 2 × 10-4
94.11
(Chen et al., 2017)
Vitamin E acetate (α-tocopheryl acetate) (VEA) (C31H52O3)
8–15
308.15–328.15
NO
2.76 × 10−4 to 7.26 × 10−4
472.76
(Han et al., 2017)
Anthraquinone violet 3RN (AV3RN) (C28H20N2Na2O8S2)
10–34
308–338
10
0.047 × 10−5 to 0.546 × 10−5
622.58
(Saadati Ardestani et al., 2020)
Phosphatidylcholine (PC) (C42H80NO8P)
12.4–17.2
313–353
NO
5.082 × 10−6 to 11.758 × 10−6
758.1
(Jash et al., 2020)
Coumarin-7 (C20H19N3O2)
9–33
308–338
13–16
0.415 × 10−5 to 1.009 × 10−5
333.38
(Sodeifian et al., 2019a)
Vanillin (C8H8O3)
8–28
313–353
16
0.14 × 10−3 to 13 × 10−3
152.15
(Maqbool et al., 2017)
Phenol (C6H6O)
10–35
333–363
28
1.14 × 10−3 to 9.064 × 10−2
94.11
(Maqbool et al., 2017)
Flufenamic acid (FFA (C14H10F3NO2)
8–21
313.2–333.2
14
0.8 × 10−6 to 2.13 × 10−4
281.23
(Tsai et al., 2017)
Nystatin (C47H75NO17)
12–30
308–338
22
0.40 × 10−6 to 1.20 × 10−5
926.1
(Sajadian et al., 2023)
Aripiprazole (C23H27CL2N3O2)
12–30
308–338
18
1.83 × 10−6 to 1.036 × 10−5
448.39
(Ansari et al., 2023)
Nifedipine (C17H18N2O6)
12.5–27.5
333.15–353.15
18
7.9 × 10−6 to 53.6 × 10−6
346.3
(Li et al., 2017)
Quinine (C20H24N2O2)
12.5–27.5
323.15–343.15
15–19
12 × 10−6 to 50.4 × 10−6
324.4
(Li et al., 2017)
Nilotinib hydrochloride monohydrate (C28H25ClF3N7O2)
12–27
308–338
12–15
0.1 × 10–5 to 0.59 × 10–5
584
(Nateghi et al., 2023)
Palbociclib (C24H29N7O2)
12–27
308–338
12–15
0.081 × 10–5 to 2.027 × 10–5
447.533
(Sodeifian et al., 2023c)
Oxaprozin (C18H15NO3)
12–40
308–338
NO MEN
3.31 × 10–5 to 1.24 × 10–3
293.317
(Alshehri et al., 2022)
lutein (β,ε-carotene-3,3′-diol) (C40H56O2)
18.7–33.55
313–333
NO
0.82 × 10–6 to 2.45 × 10–6
568.89
(Araus et al., 2019)
Metoprolol (C15H25NO3)
12–30
308–338
18
0.02 × 10−5to 8.11 × 10−5
267.36
(Alshahrani et al., 2023)
Chlorpromazine (C17H19ClN2S)
17–41
308–348
20
3.21 × 10-5to 5.25 × 10-5
318.9
(Alharby et al., 2023)
Hyoscine (C17H21NO4)
17–40
308–348
20
0.79 × 10-4to 2.83 × 10-4
303.3
(Hani et al., 2023)
Verapamil (C27H38N2O4)
12–30
308–338
12–15
3.6 × 10–6to 7.14 × 10–5
(Esfandiari et al., 2023)
Buprenorphine hydrochloride (C29H42ClNO4)
12–27
308–338
15–18
0.131 × 10-4to 4.752 × 10-4
504.1
(Sodeifian et al., 2023a)
Hydroxychloroquine sulfate (C18H28ClN3O5S)
12–27
308–338
NO
0.0304 × 10–5 to 0.5515 × 10–5
434
(Sodeifian et al., 2023b)
Probenecid (C13H19NO4S)
15–21
313.2–353.2
15–19
0.13 × 10−5to 1.45 × 10−5
285.36
(Khudaida et al., 2023b)
Warfarin (C19H16O4)
10–18
308.2–328.2
18
1.48 × 10−6 to 4.32 × 10−6
434
(Ciou et al., 2018)
Ibrutinib (C25H24N6O2)
12–27
308–338
17
3.90 × 10–6 to 1.30 × 10–5
440.51
(Sodeifian et al., 2023d)
Sitagliptin phosphate (C16H18F6N5O5P)
12–30
308–338
15–16.5
3.02 × 10–5 to 6.98 × 10−5
407.31
(Ardestani et al., 2023)
One of the biggest challenges in the development of the SCF process is the limited solubility of polar solutes in SC-CO2. As the majority of pharmaceuticals are polar molecules, the interaction of carbon dioxide (a nonpolar structure) with medications is limited. Therefore, supercritical CO2 is employed in combination with other solvents, known as “Co-solvent”, to enhance the solubility. Co-solvents can alter the polarity of the solvent. Consequently, the use of Co-solvents can enhance the solubility in SCFs (polar or non-polar). Moreover, the incorporation of small amounts (less than 10 %) of polar solvents, such as acetone, dimethyl sulfoxide (DMSO), ethanol, menthol, and methanol can significantly increase the solute solubility in SC-CO2 (Hosseini et al., 2018, Bitencourt et al., 2019, Ardestani et al., 2020, Saadati Ardestani et al., 2020, Sodeifian et al., 2021g). These Co-solvents can participate in hydrogen bonding with solute molecules and increase the solvation power of a specific supercritical fluid in solvents with lower solvation ability like water. Additionally, the impact of the Co-solvent is related to an improvement in solubility by a rise in solvent density or by intermolecular cooperation between the Co-solvent and the solute. Furthermore, an increase in the specific intermolecular interactions between the Co-solvent and one or more components of the mixed components can enhance the separation selectivity (Knez et al., 2017, Bitencourt et al., 2019, Saadati Ardestani et al., 2020, Zhan et al., 2020).
The Co-solvent effect is primarily influenced by heightened intermolecular interactions and solvent density. In systems with multiple components, solubility can be significantly increased, but selectivity remains unaffected if the increase is solely due to higher solvent mixture density. The density input to the Co-solvent impact is affected by pressure, temperature, and the addition of a Co-solvent, which can lead to the formation of clusters of SCF molecules around it, boosting overall density. The greatest density increase is observed near the critical point of the solvent mixture. An increase in pressure decreases clustering, increases SCF density, and decreases density differences between the SCF and SCF mixture, ultimately crossing density isotherms. The addition of a Co-solvent can strengthen the SCF solvent while decreasing the molar density of the solvent. Key factors influencing the Co-solvent effect include various physical interactions like dipole-induced dipole, dipole–dipole, and induced dipole-induced dipole (dispersion), as well as specific interactions like charge transfer and H-bonding complexes. A comprehensive understanding of the Co-solvent effect requires a thorough comprehension of intermolecular interactions between solvents and solutes (Prausnitz et al., 1999, Güçlü-Üstündağ and Temelli, 2005, Cui et al., 2018, Li et al., 2018, Pitchaiah et al., 2018, Peyrovedin and Shariati, 2020, Matin et al., 2022, Sajadian et al., 2023).
The Hildebrand solubility parameter (δ) and the Hansen solubility parameter (HSP) are commonly used to assess suitable solvents for specific applications based on similar solubility parameters. HSP categorizes molecular interactions into dispersion, hydrogen-bond, and polar contributions, making it applicable to both polar and non-polar mixtures. Kamlet-Taft solvent parameters (KT) like α (H-bond donor) (HBD), β (H-bond acceptor) (HBA), and solvent dipolarity/polarizability (π^*) help evaluate total solvent polarity. The entertainer effect enhances selectivity and solubility through specific intermolecular interactions like H-bonding between Co-solvent and solutes. When selecting a binary mixed-solvent, the one with higher KT-acidity is the HBD solvent, and the local composition of the HBD-HBA pair influences KT-parameters of complex molecules. In SC-CO2, non-aqueous and aqueous HBD-HBA solvent pairs act as Co-solvents, interacting with polar solutes and CO2. The HBD-HBA complex molecule impacts selectivity by specific interactions with solutes and CO2 affinity (CO2 philicity). Adjusting the HBD-HBA Co-solvent composition can enhance basicity, CO2 philicity, and specific interactions for solute dissolution in the SC-CO2 phase (Güçlü-Üstündağ and Temelli, 2005, Cui et al., 2018, Duereh and Smith, 2018, Li et al., 2018, Pitchaiah et al., 2018).
This research is aimed at understanding the solubility of RXN in SC-CO2 with or without ethanol as a Co-solvent. The static equilibrium test conditions involve the pressure range of 12, 15, 17, 21, 24, 27 and 30 and temperatures of 308, 318, 328, and 338 K. The solubility of RXN in SC-CO2 was experimentally studied to investigate the impacts of the operational factors such as temperature, pressure, and the presence of a Co-solvent. The density models of Jouyban et al., Soltani-Mazloumi, Méndez-Santiago-Teja (MST), Sodeifian-Sajadian, González et al., Garlapati–Madras, Chrastil, Kumar and Johnston (KJ), Bian et al. and Bartle et al. were used to correlate the solubility data of RXN in binary and ternary procedures. The model parameters were established, and the average absolute relative deviation (AARD (%)) was also utilized to evaluate the prediction effectiveness of the method.
2 Experiments
2.1 Materials
Rivaroxaban was acquired from Tofigh Darou drug company (Tehran, Iran) with a purity of 99 %. Additionally, further information regarding other components including carbon dioxide and ethanol can be found in Table 2. The structure of Rivaroxaban is provided in Table 3.
Material
Source
Initial mass fraction (Purity)
Final mass fraction Purity
Analysis method
Rivaroxaban
Tofigh Daru Research & Engineering Co.
0.99
0.99
HPLCa
Ethanol
Merck Co.
0.999
0.999
GCb
Carbon dioxide
Novin Oxygen Co.
0.9999
0.9999
GC
Component
Formula
Mw (g/mol)
Tm (K)
λmax [nm]
Structure
CAS number
Rivaroxaban
C19H18ClN3O5S
435.88
503.15
249
366789–02-8
2.2 Experimental apparatus
Based on Fig. 1, the experimental pilot plant was equipped with a spectrophotometer and included a CO2 tank, an air compressor (Finac, China), a high-pressure pump (Haskel pump, Burbank CA 91502, USA), a refrigeration machine, a magnetic stirrer with 100 rpm, a filter, flow control valves like a needle valve, a back-pressure valve, a metering valve, an equilibrium cell, and an oven (Memert, Germany). All the components of this high-pressure unit, including the pipeline and fittings, have a diameter of 1/8 in. and are made of 316 stainless steel. The impurities in the CO2 flow from the tank were eliminated by passing through a molecular filter with a pore size of 1 µm. The flow then reached the cooling unit where the CO2 flow liquefied due to the low interior temperature of the refrigerator (∼-15 °C). From the pressure of the CO2 tank, the liquid CO2 enters the high-pressure pump at a pressure of about 6 MPa. A manometer and transmitter were used to evaluate the pressure with an accuracy of 0.1 MPa.Schematic diagram of experimental apparatus used for measuring solubility.
The drug was homogenized in SC-CO2 using a magnetic stirrer in a 300-mL cell to achieve a lab balance cell (binary system). In the case of a ternary system or a Co-solvent approach, 3000 mg of the RXN was added to the cell with a certain amount (3 mol %) of ethanol as a Co-solvent. The temperature control was achieved using an oven. A sintered filter (1 ) was placed to keep the RXN in place on either side of the cell. Before being fed to the cell, CO2 was compressed to an appropriate pressure. Based on the preliminary test, the static time was 120 min. Saturated SC-CO2 (600 ± 0.6 % ) was inserted into the injection loop using a three-valve two-position device (shown by V1-V3) after 120 min. Upon rerouting the injection valve, the loop can be depressurized into the collecting vial to keep a particular amount of ethanol (solvent). This mechanism is summarized in Fig. 1 in four cases. After the static time, the loop should be filled. So, the valve (V1) has been opened. After filling the loop, V1 is closed. Next, V2 is opened. The loop goes to the collection vial, then V3 is opened and all the lines and the loop are rinsed with ethanol (1 mL). Also, in the picture, the green and black colors show the open and closed positions of the valves, respectively. This process was repeated three times for every data point and each system.
The solution was collected in a container with a final volume of 5 mL (±0.2 %). Each test was repeated multiple times. The absorbance was measured spectrophotometrically using a Jenway UV-V equipped with a quartz cell, at a maximum wavelength (
) of 249 nm, to monitor the solubility. By utilizing the alignment bend (with a correlation coefficient of 0.989) and the UV absorbance, the solubility was calculated based on the solute concentration. The calibration curve and the linear relationship of the regression data over a wide concentration range confirmed the suitability of the method. Additionally, the melting point was estimated using the DSC test, as shown in Fig. 2. The calculation method for pressure fluctuation during sampling was also presented in the supplementary information, Table (S1).DSC analysis of Rivaroxaban.
The equilibrium mole fraction y2 and solubility,
of RXN in SC-CO2 were calculated at various pressure and temperature levels as follows (Sodeifian et al., 2023e):
2.3 Empirical and semi-empirical density-based models
Several empirical and semi empirical models can be used to determine the solubility of solids (drugs) in SC-CO2. For the binary system in this study, the density-based models of Chrastil, Bian et al., Jouyban et al., Bartle et al., Mendez − Santiago − Teja (MST), and Kumar-Johnston (KJ) were adopted. Concerning the ternary approach with ethanol (Co-solvent), the Garlapati–Madras, Sodeifian-Sajadian, MST, González et al., Soltani-Mazloumi, and Jouyban et al. models were used. Empirical and semi-empirical models were employed to establish the connection between RXN solubility. Tables 4.a and 4.b provide a summary of the equations applied in binary and ternary approaches respectively and Table 5 outlines the parameters employed in the models.
Model
Formula/ explain
constant
ref
Chrastil
Semi-empirical
3
(Chrastil, 1982)
An equation describes the formation of a solvate complex ABk in a system where one unit of solute A combines with k units of solvent B. It highlights a correlation between solubility and density in a supercritical fluid, as well as a relationship between solubility and temperature. However, Chrastil's equation has limitations, such as being unsuitable for solubility levels above 100–200 kg m−3 and lacking validity across a wide range of temperatures. This model is designed for pure fluids and can be applied in mixtures with consistent Co-solvent mole fractions, assuming these mixtures behave like pure fluids at constant concentrations. Overall, the model provides a macroscopic view of the molecular environment in the fluid phase without requiring knowledge of the solute's properties.
(Sauceau et al., 2003, Hojjati et al., 2007, Sparks et al., 2008, Kostrzewa et al., 2019)
Bartle et al.
Semi-empirical
3
(Bartle et al., 1991)
This model illustrates the relationship between solubility and solvent density. The correlation is expressed in a linear manner using the enhancement factor of the solute with respect to the density of the solvent. By fitting the correlation to experimental data, the coefficients a0, a1, and a2 can be determined. The parameter a2 is particularly useful in estimating the heat of vaporization of the solute, Hvap (Hvap = − a2R). By utilizing the values of Htotal and Hvap, the heat of solvation can be estimated for each solute-CO2 system. The Bartle model, which includes an individual pressure term, is expected to provide more reliable correlated results for solubility data at different pressures.
(Hojjati et al., 2007, Sparks et al., 2008)
Mendez − Santiago − Teja (MST)
Semi-empirical
3
(Sodeifian et al., 2018a)
This model utilizes the principles of dilute solutions and employs the algorithm of the Henry constant of solute in a supercritical fluid. Within this theoretical framework, the enhancement factor is ascertained by the solvent's density, leading to straightforward equations for a range of thermodynamic properties of dilute near-critical binary mixtures. Additionally, this model allows for the normalization of data across varying temperatures.
(Sauceau et al., 2003, Hojjati et al., 2007, Sparks et al., 2008)
Jouyban et al.
Empirical
6
(Jouyban et al., 2002a, 2002b)
The solubilities of organic solids in SC-CO2 can be accurately predicted using the empirical model developed by Jouyban et al. This model takes into account the interplay between solute mole fraction, linear pressure, and temperature, allowing for the estimation of solubility data that has not been measured. Additionally, it can be used to identify any outliers in experimental solubility data, providing valuable insights into the behavior of these systems.
(Jouyban et al., 2002a, Sridar et al., 2013)
Bian et al.
Empirical
5
(Bian et al., 2011)
The density-based empirical model proposed by Bian et al. offers a comprehensive understanding of the solubility of compounds in SC-CO2. It accounts for the intricate interplay between solubility and density of the supercritical fluid at varying temperatures and pressures. Additionally, it considers the correlation between solubility and temperature under isopycnic conditions, as well as the impact of temperature and pressure on the association number. This model is derived from Chrastil's equation.
(Sridar et al., 2013)
Kumar and Johnston (KJ)
Semi-empirical
3
(Kumar and Johnston, 1988)
In 1988, a thermodynamic formalism was introduced to explain the connection between the solubility of a nonvolatile solute in a SCF and the density of the fluid phase. This model suggests that the logarithm of the solute's mole fraction in the fluid phase shows a nearly linear relationship with either the logarithm or the density of the SCF phase in the vicinity of the critical point, depending on the specific system. The slope of this linear correlation is determined by both the partial molar volume of the solute in the SCF phase and the isothermal compressibility of the fluid. Through the analysis of solubility data from existing literature, scientists have been able to calculate partial molar volumes using this framework, and these calculated values are in good agreement with independently measured data.
(Kumar and Johnston, 1988, Yan et al., 2022)
Model
Formula
constant
Ref
Mendez−Santiago−Teja (MST)
semi-empirical
4
(Méndez-Santiago and Teja, 1999)
A correlation with four adjustable parameters was derived by combining the Mendez-Santiago and Teja equation with a Clausius-Clapeyron-type equation and including sublimation pressure. This correlation is used to assess the impact of density, temperature, and Co-solvent composition on the solubility of the ternary system.
(Sauceau et al., 2003)
Sodeifian-Sajadian
semi-empirical
4
(Sodeifian et al., 2019c)
Four experimental data points were selected as the minimum requirement from the collected data sets to train the proposed model for determining the solubilities of organic solids in SC-CO2 when a Co-solvent is present. The development of this model is based on the works of González et al. and Chrastil models.
(Rojas et al., 2023)
González et al.
semi-empirical
4
(González et al., 2001)
González and colleagues introduced a thermodynamic model based on the Chrastil model, utilizing the mass-action law to predict solute solubility in non-entrained supercritical fluids. This model has shown effectiveness, especially in systems where the presence of an entrainer boosts solute solubility significantly, particularly in cases with strong solute-entrainer interaction. The model incorporates the logarithmic dependence of solubility on fluid density along with an exponential relationship between solubility and Co-solvent concentration. It is built on the assumption of cluster or solvate complex formation involving the solute, entrainer, and solvent, which is consistent with the observed decrease in solute solubility with temperature. Therefore, the model may not accurately forecast solubility in systems where the Co-solvent only serves as a Co-solvent for CO2, lacking the entrainer effect that enhances both solubility and extraction selectivity.
(González et al., 2001)
Soltani-Mazloumi
Empirical
5
(Soltani and Mazloumi, 2017)
Soltani Mazloumi is an innovative experimental framework that incorporates five parameters to forecast solid solubility in supercritical carbon dioxide with the presence of a Co-solvent. This model considers various input data, including temperature, pressure, and density correlations. It is important to highlight that this model is derived from Hozhabr et al.'s model, showcasing a linear relationship between ln
and ln P, a nonlinear association between ln
' and temperature as well as density, a linear correlation between ln
and a linear correlation between ln
' and ln
(Co-solvent mole fraction).
(Soltani and Mazloumi, 2017)
Garlapati–Madras
semi-empirical
7
(Saadati Ardestani et al., 2020)
In 2010, the Garlapati–Madras equation was developed with seven constants, inspired by the model introduced by Jouyban et al. This equation is used to establish a relationship between the solubilities of high molecular weight solids in SC-CO2, with or without Co-solvents, considering temperature, the density of SC-CO2, and the mole fraction of Co-solvent.
(Garlapati and Madras, 2010)
Jouyban et al.
Empirical
7
(Jouyban et al., 2002b)
The training of the proposed model to predict the solubilities of organic solids in SC-CO2, considering the presence of a Co-solvent, utilizes a minimum of six experimental data points from the collected data sets. To estimate solubility at various temperatures and pressures, an interpolation technique was employed. This correlation provides numerous benefits, such as a simple calculation procedure and higher accuracy in comparison to other empirical equations and equations of state.
(Jouyban et al., 2002b)
Parameter
Description
System
Mole fraction (RXN + SC-CO2)
Binary system
Mole fraction (RXN + SC-CO2 + Ethanol)
Ternary system
The mole fraction of Co-solvent
Ternary system
Adjustable parameters
Binary system
Ternary system
Density of SC-CO2 (kg m−3)
Binary & Ternary system
Reference density (700 kg m−3)
Binary system
Pref
Reference Pressure (0.1 MPa)
Binary & Ternary system
P
System Pressure (MPa)
Binary & Ternary system
T
System Temperature (K)
Empirical and semi-empirical models' constants were assessed using experimental information. Variable parameters were also fine-tuned using the simulated annealing (SA) algorithm in MATLAB software. The AARD% was also applied to evaluate the accuracy of the model.
parameters of each equation. The R2 correlation coefficient was also used to compare different models:
3 Results and discussion
3.1 Solubility data systems- role of Co-solvent
The experimental solubilities of RXN in SC-CO2 with and without ethanol Co-solvent (ternary and binary) were explored experimentally at 308–338 K and 12–30 MPa, as reported in Tables 6 and 7. The Span-Wanger EoS was used to determine the SC-CO2 density (Span and Wagner, 1996). Additionally, each test was reassessed three times to enhance the precision, and the relative standard uncertainties fell below 5 %. The uncertainty of solubility was determined according to the guide of uncertainty measurement (GUM) proposed by the joint committee for guides in metrology (2008). Figs. 3 and 4 present the RXN mole fraction solubility vs. pressure and density at various temperatures for binary and ternary systems, respectively. In 2022, Sodeifian et al. studied RXN in binary mode. According to Table 6, the data difference is lower than 9 % (Sodeifian et al., 2023e). The supplementary information data consists of Tables S2 and S3, containing the tabulated information necessary for the calculation and analysis of ethanol (Co-solvent) content, mixture density, and CO2 mass within the mixture. b The experimental standard deviation and the experimental standard deviation of the mean (SD) were calculated by
and
respectively.
Temperaturea (K)
Pressurea (MPa)
Densityb (kg/m3)
Binary
y2 × 105 (Mole Fraction)
Experimental standard deviation, S(y) × (1 0 5)c
S × 10 (Solubility (g/l))
Expanded uncertainty of mole fraction (1 0 5), Ucd
308
12
768.42
0.405
0.007
0.031
0.011
15
816.06
0.564
0.011
0.046
0.014
18
848.87
0.745
0.018
0.063
0.020
21
874.4
1.102
0.031
0.095
0.032
24
895.54
1.248
0.041
0.111
0.043
27
913.69
1.677
0.063
0.152
0.064
30
929.68
1.924
0.080
0.177
0.081
318
12
659.73
0.256
0.006
0.017
0.011
15
743.17
0.458
0.013
0.034
0.016
18
790.18
0.676
0.022
0.053
0.023
21
823.7
1.042
0.038
0.085
0.039
24
850.1
1.389
0.059
0.117
0.060
27
872.04
1.81
0.065
0.156
0.066
30
890.92
2.163
0.087
0.191
0.088
328
12
506.85
0.171
0.003
0.009
0.012
15
654.94
0.359
0.007
0.023
0.012
18
724.13
0.593
0.014
0.043
0.017
21
768.74
0.971
0.027
0.074
0.029
24
801.92
1.57
0.054
0.125
0.056
27
828.51
1.991
0.048
0.163
0.050
30
850.83
2.396
0.069
0.202
0.071
338
12
384.17
0.102
0.003
0.004
0.016
15
555.23
0.239
0.009
0.013
0.014
18
651.18
0.48
0.019
0.031
0.021
21
709.69
0.836
0.023
0.059
0.025
24
751.17
1.71
0.055
0.127
0.056
27
783.29
2.164
0.078
0.168
0.079
30
809.58
2.572
0.007
0.206
0.019
Temperaturea (K)
Pressurea (MPa)
Density (kg/m3)
Ternary
y'2 × 104
(Mole Fraction)
Experimental standard deviation, S (y ') × 105b
Expanded uncertainty of mole fraction (1 0 5), Uc c
Mass ethanol (gr)
e
(Co-solvent effect)
308
12
769.05
0.439
0.0755
0.080
7.463
10.84
15
815.18
0.582
0.1164
0.122
7.926
10.32
18
846.85
0.753
0.1807
0.187
8.244
10.11
21
871.44
0.999
0.2797
0.286
8.492
9.07
24
891.764
1.087
0.3594
0.365
8.698
8.71
27
909.18
1.208
0.451
0.457
8.874
7.20
30
924.51
1.34
0.5539
0.56
9.029
6.96
318
12
663.14
0.328
0.0787
0.082
6.407
12.81
15
744.53
0.528
0.1542
0.158
7.218
11.53
18
790.14
0.763
0.2442
0.249
7.674
11.29
21
822.57
1.031
0.3712
0.377
8.000
9.89
24
848.04
1.208
0.5154
0.251
8.256
8.70
27
869.17
1.441
0.5188
0.527
8.469
7.96
30
887.32
1.603
0.6412
0.649
8.653
7.41
328
12
512.60
0.26
0.0458
0.050
4.923
15.20
15
658.45
0.442
0.0884
0.093
6.361
12.31
18
726.00
0.708
0.1699
0.176
7.033
11.94
21
769.36
1.004
0.2811
0.288
7.466
10.34
24
801.51
1.45
0.5027
0.510
7.788
9.24
27
827.21
1.566
0.3758
0.388
8.047
7.87
30
848.74
1.794
0.5191
0.531
8.263
7.49
338
12
390.45
0.191
0.0611
0.065
3.731
18.73
15
560.44
0.387
0.1393
0.142
5.393
16.19
18
654.77
0.652
0.2608
0.264
6.324
13.58
21
711.93
1.079
0.3021
0.310
6.893
12.91
24
752.30
1.635
0.5232
0.533
7.296
9.56
27
783.47
1.846
0.6646
0.674
7.607
8.53
30
808.92
2.022
0.8088
0.819
7.863
7.86
The RXN solubility in the binary system.
The RXN solubility in the ternary system.
In general, an increase in pressure enhances the density of SC-CO2 and its solvation power. Consequently, the solubility of RXN in SC-CO2 increases with pressure increment at constant temperature in both systems. Analysis of RXN solubility in binary and ternary systems indicates a significant increase in the solubility of RXN in the presence of a Co-solvent (ethanol). According to the generally recognized as safe (GRAS) designation, ethanol is an ideal Co-solvent for food applications (Güçlü-Üstündağ and Temelli, 2005). The maximum and minimum effects of the Co-solvent are 18.73 (338 K and 12 MPa) and 6.96 (308 K and 30 MPa), respectively, as determined by comparing the data and calculating the solubility enhancement (e) due to the efficacy of ethanol Co-solvent on the RXN solubility in SC-CO2 (Eq. (9) (Araus et al., 2019, Sodeifian et al., 2021).The influence of Co-solvent (ethanol) on solubility of RXN in SC-CO2. a. pressure, b. density.
CO2 cannot be employed as a suitable solvent for most medicinal chemicals due to its low polarity. Moreover, hydrophobic and polar molecules are insoluble in SC-CO2. Therefore,
variant Co-solvents have been introduced to enhance the solubility of drugs in SC-CO2. Ethanol is miscible with SC-CO2 and has shown a high dissolving capacity for numerous chemicals. Thus, ethanol can be employed as a Co-solvent in SC-CO2 systems to improve the dissolving capability (Knez et al., 2017, Cheng et al., 2018).
Small concentrations of Co-solvents can be used to increase the solvation power of SC-CO2. The effect of the Co-solvent was determined based on its concentration in the supercritical phase, which can be influenced by the phase and the treatment of the combination. To determine the influence of the Co-solvent, solvent-co-solvent combinations in a supercritical state (completely miscible) should be used (Güçlü-Üstündağ and Temelli, 2005, Pitchaiah et al., 2018). The key factor in the effect of the Co-solvent involves an increase in solvent density and intermolecular interactions. In multi-component systems, solubility can be improved either selectively or non-selectively. Selectivity does not increase in situations where the rise in insolubility is the result of an increment in the density of the solvent mixture. An increase is expected in both solubility and selectivity in the case of a specific intermolecular interaction between the Co-solvent and one of the solutes (such as H-bonding) (Güçlü-Üstündağ and Temelli, 2005, Cui et al., 2018, Li et al., 2018).
The impact of Co-solvent on solvent density, and therefore its contribution to the Co-solvent effect, varies depending on temperature and the specific Co-solvent. The inclusion of a Co-solvent increases the overall density of the supercritical fluid (SCF) by raising the density of the Co-solvent and causing SCF molecules to cluster around it. These density variations are particularly noticeable near the critical point of the solvent mixture, where the densities of both the Co-solvent and the SCF fluctuate the most, resulting in the highest degree of clustering. As the pressure increases, the density of the SCF mixture increases while the clustering decreases, causing the density isotherms to intersect. It should be noted that the addition of a Co-solvent, such as a hydrocarbon with a large molar volume, can enhance the solvation power of the SCF while reducing its molar density (Güçlü-Üstündağ and Temelli, 2005, Li et al., 2018).
A proper understanding of the Co-solvent outcome demands sufficient cognition of the intermolecular interactions between the solutes and solvents. The Co-solvent effect is mostly influenced by solute-co-solvent physical interactions such as dipole–dipole, dipole-induced dipole, and induced dipole-induced dipole (dispersion) interactions, as well as more specialized interactions such as H-bonding and charge transfer complexes (Prausnitz et al., 1999, Güçlü-Üstündağ and Temelli, 2005, Cui et al., 2018, Peyrovedin and Shariati, 2020, Matin et al., 2022).
H-bonding could be a donor–acceptor action and reaction, including H-bond- donating and accepting atoms. H-bonds are formed when the electronegativity of the H-bond donor is sufficiently high to draw electrons, partially exposing the protons. The acceptor atom has to possess lone-pairs or polarizable electrons to form a bond with the donor species. Functional groups could serve as acceptors (e.g., C = O), donors, or both (e.g., OH). As the most prevalent cases in essence and chemistry, moderate H-bonds are generated between neutral donors and acceptors like –OH and O = C (Jeffrey and Jeffrey, 1997). In the mixture of SC-CO2 and ethanol, ethanol forms H-bonds for weak binding to CO2 as a result of quadrupole-dipole interaction. Furthermore, the hydrogen bonding between RXN and this Co-solvent declined chemical potential, offering additional solute molecules to the supercritical phase (Güçlü-Üstündağ and Temelli, 2005, Araus et al., 2019, Ardestani et al., 2020).
Several articles have investigated crossover pressure and proposed some methods to predict the crossover pressure region (Adachi and Lu, 1983, Chimowitz et al., 1988, Del Valle and Aguilera, 1988, de Melo et al., 2009, Tabernero et al., 2010, Budkov et al., 2019, Kalikin et al., 2020, Kalikin et al., 2021). Correlation of the crossover pressure for the ternary system has been presented by Johnston et al.(Adachi and Lu, 1983) and Chimowitz et al.(Chimowitz et al., 1988). The crossover pressure was related to the enthalpy of sublimation and the partial molar enthalpy of the solute in the supercritical phase. The locations of the lower and upper crossover pressures were determined at the point where the partial molar enthalpy equals the negative of the enthalpy of sublimation. Johnston et al. (1987) applied the Peng-Robinson EoS with a binary interaction parameter regressed from a single experimental point to evaluate the partial molar enthalpy of the solute for determining the crossover points. Chimowitz et al. (1988) used a perturbed hard-sphere model EoS to correlate the crossover pressure for binary and ternary systems. Both of these methods require the P-y-T data to allow the prediction of the crossover point. Kalikin et al. (Kalikin et al., 2021) investigated the solubility of a set of poorly soluble drugs, which have been computed in a wide area of the phase diagram, based on the classical density functional theory. They found that the wider the temperature region of the experimental study, the more pronounced the effect of the crossover points drift. They also estimated solubility values using in situ IR spectroscopy and molecular dynamics simulations along the mentioned isochores and isotherms, respectively. Furthermore, they believed that the critical parameters, sublimation pressure, and molar volume of the compound play a crucial role in the determination of the crossover pressure (Tabernero et al., 2010). De Melo et al. investigated the Peng-Robinson-LCVM-UNIFAC equation and the effect of any uncertainty of some solid pure component properties on the upper crossover pressure. It is shown that the slope of the sublimation pressure curve plays a major role in the accuracy of the upper crossover pressure. To sum up, the crossover region depends on the critical properties of solutes, sublimation pressure, enthalpy of sublimation, partial molar enthalpy, and molar volume of the solute.
As suggested by the chemical structure of RXN (Table 3), its OH, NH, and C = O groups increase its polarity. Accordingly, the solubility of RXN in a high-density solvent will be higher than in a low-density solvent for both binary and ternary systems. At high pressures, SCF behaves like a liquid. Regarding the higher solvation power of liquids compared to gases, RXN is more soluble in solvents with higher density (see Fig. 4). Temperature is another key factor in the solubility of RXN. Based on Figs. 3 and 4, the solubility of RXN in both binary and ternary systems increased with constant pressure, due to temperature elevation. The binary and ternary systems showed crossover pressures. Concerning the solubility of solid materials in supercritical fluids, there is a well-known phenomenon called “retrograde vaporization” (RV), in which a rise in temperature at steady pressure causes a decline in solubility (Kalikin et al., 2021). The limits of this area are illustrated by two positions where all isotherms intersect and the plot of solubility vs. temperature shows extrema; the pressure values corresponding to these extrema are known as the lower and upper crossover pressures. In the case of binary and ternary systems, the crossover pressures roughly reside at 24 MPa and 21 MPa, respectively. The temperature of the system shows different impacts on RXN solubility at pressures higher and lower than the crossover pressure. The effects of temperature enhancement on solubility may vary due to the temperature dependence of the density of the solvent and the vapor pressure of the solute.
3.2 Analyzed solubility data correlation of two system
RXN solubility data were compared in two systems using ten empirical density-based models (Bian et al., Jouyban et al., Chrastil, Bartle et al., Mendez − Santiago − Teja (MST), González et al., Kumar, and Johnston (KJ), Sodeifian–Sajadian, Soltani-Mazloumi and Garlapati-Madras). The RXN solubility in SCF was correctly correlated by all correlations, as shown by the AARD% and Radj values. Using the calculated customizable parameters, the offered models may be employed to predict RXN solubility in binary and ternary modes at definite pressures and temperatures. The solubility data were correlated with high precision using the acquired adjustable parameters. Tables 8 and 9 list the parameters of the empirical and semi empirical model in the binary RXN-CO2 system and the ternary RXN-Ethanol-CO2 system, respectively. The correlation outcomes of each approach are displayed in Figs. 6 and 7. The AARD% of each model in both systems is shown in Fig. 8. Additionally, Jouyban et al. (AARD%=7.40 and Radj = 0.993) is the most accurate model in the binary system. As seen, all models provide proper accuracy in ternary models, while the Garlapati-Madras (AARD%=6.16 and Radj = 0.991) and Sodeifian-Sajadian (AARD%=6.13 and Radj = 0.979) and Soltani-Mazloumi (AARD%=6.89 and Radj = 0.987) models for the ternary system are the most accurate models.
Model
AARD%
Radj
Chrastil
8.794
−5323.289
−43.942
–
–
–
16.75
0.989
KJ
−2.449
0.009
−5352.72
–
–
–
12.42
0.989
Bian et al.
−4.736
0.003
−2324.664
−3.713
17.185
–
8.05
0.986
Bartle et al.
16.928
−7878.695
0.014
–
–
–
16.79
0.986
MST
4.797
−1.182
10-4
18.672
–
–
–
15.93
0.990
Jouyban et al.
–33.695
0.002
−0.004
9.689
10-5
0.100
2.062
7.40
0.993
Model
AARD%
Radj
MST
−1920.282
3.510
16.855
−2.517
10-5
–
–
–
12.37
0.986
González et al.
5.450
−2.867
−4.498
104
−41.694
–
–
–
12.17
0.985
Sodeifian-Sajadian
−2.360
−0.482
0.018
0.695
–
–
–
6.13
0.979
Soltani-Mazloumi
4.608
−5194.273
1.885
−0.386
−0.495
–
–
6.89
0.987
Garlapati–Madras
−93.651
−6.345
0.011
−26.658
10.571
−8.462
3.027
6.16
0.991
Jouyban et al.
−50.732
−1.899
−0.007
−0.002
5.083
10-4
0.008
6.70
9.39
0.989
Comparison of experimental (points) and calculated (line) solubilities of RXN in the binary system: (a) Chrastil, (b) KJ., (c) Bian et al (d) Bartle et al, (e) MST (f) Jouyban et al. models at various temperatures.
Comparison of experimental (points) and calculated (line) solubilities of RXN in the binary system: (a) Chrastil, (b) KJ., (c) Bian et al (d) Bartle et al, (e) MST (f) Jouyban et al. models at various temperatures.
Comparison of experimental (points) and calculated (line) solubilities of RXN in the ternary system (3 mol % ethanol): (a) MST, (b) González et al., (c) Sodeifian-Sajadian, (d) Soltani-Mazloumi. (e) Garlapati–Madras and (f) Jouyban et al. models at various temperatures.
Comparison of experimental (points) and calculated (line) solubilities of RXN in the ternary system (3 mol % ethanol): (a) MST, (b) González et al., (c) Sodeifian-Sajadian, (d) Soltani-Mazloumi. (e) Garlapati–Madras and (f) Jouyban et al. models at various temperatures.
Comparing ARRD percentages between Binary and Ternary models.
4 Conclusion
The current research explored the RXN solubility in two systems (binary: SC-CO2 and ternary: SC-CO2 with Co-solvent (ethanol) at different pressures (12–30 MPa) temperatures (308–338 K). The RXN solubility in the binary and ternary systems ranged based on mole fraction from to and from to , respectively. The solubility values of RXN were correlated by various semi-empirical equations based on the corresponding equilibrium. Accordingly, the incorporation of ethanol Co-solvent considerably improved the RXN solubility due to dipole–dipole and dipole-induced dipole interactions between the Co-solvent and RXN. The highest Co-solvent effect (18.73) was identified at 12 MPa and 338 K, further confirming this hypothesis. The highest RXN solubility (y'2 = 2.02 10-4) at 30 MPa and 338 K, was recorded in a system with ethanol Co-solvent. Furthermore, according to the AARD% and Radj values of the empirical and semi-empirical approaches, Jouyban et al. model for binary system and Garlapati-Madras, Sodeifian-Sajadian and Soltani-Mazloumi models can properly correlate the ternary system at examined temperatures and pressures.
CRediT authorship contribution statement
M.A.: Methodology, Writing- Original draft preparation, Data curation and Software, Reviewing and Editing. N.E.: Methodology, Writing- Original draft preparation, Conceptualization, Investigation, Validation, funding acquisition, Reviewing and Editing. B.H.: Validation, Methodology, Investigation, Reviewing. S.A.S.: Conceptualization, Project administration, Software, Supervision, Reviewing and Editing. A.A.: Investigation, Writing, Reviewing and Editing.
Declaration of Competing Interest
The authors declare that they have no known competing financial interests or personal relationships that could have appeared to influence the work reported in this paper.
References
- Experimental measurement and thermodynamic modeling of solubility of Riluzole drug (neuroprotective agent) in supercritical carbon dioxide. Fluid Phase Equilibria.. 2022;113711
- [CrossRef] [Google Scholar]
- Experimental evaluation and thermodynamic analysis of Febuxostat solubility in supercritical solvent. Journal of Molecular Liquids.. 2022;364:120040.
- [CrossRef] [Google Scholar]
- Laboratory determination and thermodynamic analysis of alendronate solubility in supercritical carbon dioxide. Journal of Molecular Liquids.. 2022;367:120242.
- [CrossRef] [Google Scholar]
- Supercritical fluid extraction with carbon dioxide and ethylene. Fluid Phase Equilibria.. 1983;14:147-156.
- [CrossRef] [Google Scholar]
- Advancing nanomedicine production via green thermal supercritical processing: Laboratory measurement and thermodynamic modeling. Journal of Molecular Liquids.. 2023;383:122042.
- [CrossRef] [Google Scholar]
- Measurement of metoprolol solubility in supercritical carbon dioxide; experimental and modeling study. Case Studies in Thermal Engineering.. 2023;42:102764.
- [CrossRef] [Google Scholar]
- Design of predictive model to optimize the solubility of Oxaprozin as nonsteroidal anti-inflammatory drug. Scientific Reports.. 2022;12:13106.
- [CrossRef] [Google Scholar]
- Experimental study and thermodynamic modeling of clonazepam solubility in supercritical carbon dioxide. Fluid Phase Equilibria.. 2023;113880
- [CrossRef] [Google Scholar]
- Solubility measurement of the fludrocortisone acetate in supercritical carbon dioxide: Experimental and modeling assessments. The Journal of Supercritical Fluids.. 2022;190:105752.
- [CrossRef] [Google Scholar]
- Solubility of Aripiprazole in Supercritical Carbon Dioxide: Experimental and Modeling Evaluations. 2023
- [CrossRef]
- Cosolvent effect of ethanol on the solubility of lutein in supercritical carbon dioxide. The Journal of Supercritical Fluids.. 2019;143:205-210.
- [CrossRef] [Google Scholar]
- Experimental Measurement and Thermodynamic Modeling of Capecitabine (an Anticancer Drug) Solubility in Supercritical Carbon Dioxide in a Ternary System: Effect of Different Cosolvents. Journal of Chemical & Engineering Data.. 2020;65:4762-4779.
- [CrossRef] [Google Scholar]
- Experimental and modeling of solubility of sitagliptin phosphate, in supercritical carbon dioxide: proposing a new association model. Scientific Reports.. 2023;13:17506.
- [CrossRef] [Google Scholar]
- Kinetic modeling to explain the release of medicine from drug delivery systems. ChemBioEng Reviews.. 2023;10:1006-1049.
- [CrossRef] [Google Scholar]
- Supercritical carbon dioxide utilization in drug delivery: Experimental study and modeling of paracetamol solubility. European Journal of Pharmaceutical Sciences.. 2022;177:106273.
- [CrossRef] [Google Scholar]
- Solubilities of solids and liquids of low volatility in supercritical carbon dioxide. Journal of Physical and Chemical Reference Data.. 1991;20:713-756.
- [CrossRef] [Google Scholar]
- Measurement and thermodynamic modeling of solubility of Erlotinib hydrochloride, as an anti-cancer drug, in supercritical carbon dioxide. Fluid Phase Equilibria.. 2023;113877
- [CrossRef] [Google Scholar]
- An improved density-based model for the solubility of some compounds in supercritical carbon dioxide. Thermochimica Acta.. 2011;519:16-21.
- [CrossRef] [Google Scholar]
- Prediction of solid solute solubility in supercritical CO2 with cosolvents using the CPA EoS. Fluid Phase Equilibria.. 2019;482:1-10.
- [CrossRef] [Google Scholar]
- Possibility of pressure crossover prediction by classical dft for sparingly dissolved compounds in scco2. Journal of Molecular Liquids.. 2019;276:801-805.
- [CrossRef] [Google Scholar]
- Rationale and design for the study of rivaroxaban to reduce thrombotic events, hospitalization and death in outpatients with COVID-19: The PREVENT-HD study. American Heart Journal.. 2021;235:12-23.
- [CrossRef] [Google Scholar]
- Experimental investigation for the solubility and micronization of pyridin-4-amine in supercritical carbon dioxide. Journal of CO2 Utilization.. 2017;18:173-180.
- [CrossRef] [Google Scholar]
- Solubility of vitamin E acetate in supercritical carbon dioxide with ethanol as cosolvent. Journal of Chemical & Engineering Data.. 2018;63:4248-4255.
- [CrossRef] [Google Scholar]
- Analysis of retrograde behavior and the cross-over effect in supercritical fluids. Fluid Phase Equilibria.. 1988;44:23-52.
- [CrossRef] [Google Scholar]
- Solubility of solids and liquids in supercritical gases. The Journal of Physical Chemistry.. 1982;86:3016-3021.
- [CrossRef] [Google Scholar]
- Measurement of solid solubility of warfarin in supercritical carbon dioxide and recrystallization study using supercritical antisolvent process. Advanced Powder Technology.. 2018;29:479-487.
- [CrossRef] [Google Scholar]
- Rivaroxaban versus warfarin for treatment and prevention of recurrence of venous thromboembolism in African American patients: a retrospective cohort analysis. Thrombosis Journal.. 2020;18:1-9.
- [CrossRef] [Google Scholar]
- Solubility and data correlation of a reactive disperse dye in a quaternary system of supercritical carbon dioxide with mixed cosolvents. Journal of the Taiwan Institute of Chemical Engineers.. 2018;91:213-223.
- [CrossRef] [Google Scholar]
- Solid pure component property effects on modeling upper crossover pressure for supercritical fluid process synthesis: A case study for the separation of Annatto pigments using SC-CO2. The Journal of Supercritical Fluids.. 2009;49:1-8.
- [CrossRef] [Google Scholar]
- An improved equation for predicting the solubility of vegetable oils in supercritical carbon dioxide. Industrial & Engineering Chemistry Research.. 1988;27:1551-1553.
- [CrossRef] [Google Scholar]
- Enhanced solubility of siloxy-modified polyhedral oligomeric silsesquioxanes in supercritical carbon dioxide. The Journal of Supercritical Fluids.. 2019;143:358-364.
- [CrossRef] [Google Scholar]
- Atrial Fibrillation and use of rivaroxaban: performance of the prothrombin time/INR as a function of time after blood collection. International Journal of Cardiovascular Sciences.. 2020;34:116-121.
- [CrossRef] [Google Scholar]
- Strategies for using hydrogen-bond donor/acceptor solvent pairs in developing green chemical processes with supercritical fluids. The Journal of Supercritical Fluids.. 2018;141:182-197.
- [CrossRef] [Google Scholar]
- Production of micro and nano particles of pharmaceutical by supercritical carbon dioxide. The Journal of Supercritical Fluids.. 2015;100:129-141.
- [CrossRef] [Google Scholar]
- Solubility of Lacosamide in supercritical carbon Dioxide: An experimental analysis and thermodynamic modeling. Journal of Molecular Liquids.. 2022;360:119467.
- [CrossRef] [Google Scholar]
- Synthesis of 5-fluorouracil nanoparticles via supercritical gas antisolvent process. The Journal of Supercritical Fluids.. 2013;84:205-210.
- [CrossRef] [Google Scholar]
- Kinetic Modeling of the Gas Antisolvent Process for Synthesis of 5-Fluorouracil Nanoparticles. Chemical Engineering & Technology.. 2014;37:73-80.
- [CrossRef] [Google Scholar]
- Ampicillin nanoparticles production via supercritical CO2 gas antisolvent process. Aaps Pharmscitech.. 2015;16:1263-1269.
- [CrossRef] [Google Scholar]
- Optimal thermodynamic conditions for ternary system (CO2, DMSO, ampicillin) in supercritical CO2 antisolvent process. Journal of the Taiwan Institute of Chemical Engineers.. 2015;50:31-36.
- [CrossRef] [Google Scholar]
- CO2 Utilization as Gas Antisolvent for the Pharmaceutical Micro and Nanoparticle Production: A Review. Arabian Journal of Chemistry.. 2022;104164
- [CrossRef] [Google Scholar]
- Solubility measurement of verapamil for the preparation of developed nanomedicines using supercritical fluid. Scientific Reports.. 2023;13:17089.
- [CrossRef] [Google Scholar]
- Experimental and modeling investigation of Glibenclamide solubility in supercritical carbon dioxide. Fluid Phase Equilibria.. 2022;556:113408.
- [CrossRef] [Google Scholar]
- Evaluation of the incidence of bleeding in patients prescribed rivaroxaban for the treatment and prevention of deep vein thrombosis and pulmonary embolism in UK secondary care: an observational cohort study. BMJ Open.. 2020;10:e038102.
- [Google Scholar]
- Drug-Drug Interactions Leading to Adverse Drug Reactions with Rivaroxaban: A Systematic Review of the Literature and Analysis of VigiBase. Journal of Personalized Medicine.. 2021;11:250.
- [CrossRef] [Google Scholar]
- Rivaroxaban, a novel option for patients with atrial fibrillation and a bioprosthetic mitral valve. European Heart Journal 2021
- [CrossRef] [Google Scholar]
- Garlapati, C. and G. Madras, 2010. New empirical expressions to correlate solubilities of solids in supercritical carbon dioxide. Thermochimica Acta. 500, 123-127. ttps://doi.org/10.1016/j.tca.2009.12.004.
- Modified mass action law-based model to correlate the solubility of solids and liquids in entrained supercritical carbon dioxide. Journal of Chromatography a.. 2001;910:119-125.
- [CrossRef] [Google Scholar]
- Solubility of the antibiotic Penicillin G in supercritical carbon dioxide. The Journal of Supercritical Fluids.. 1999;15:183-190.
- [CrossRef] [Google Scholar]
- An equation-of-state contribution for polar components: Quadrupolar molecules. AIChE Journal.. 2005;51:2556-2568.
- [CrossRef] [Google Scholar]
- Perturbed-chain SAFT: An equation of state based on a perturbation theory for chain molecules. Industrial & Engineering Chemistry Research.. 2001;40:1244-1260.
- [CrossRef] [Google Scholar]
- Solubility behavior of ternary systems of lipids, cosolvents and supercritical carbon dioxide and processing aspects. The Journal of Supercritical Fluids.. 2005;36:1-15.
- [CrossRef] [Google Scholar]
- Solubility of vitamin E acetate in supercritical carbon dioxide: measurement and correlation. Journal of Chemical & Engineering Data.. 2017;62:3854-3860.
- [CrossRef] [Google Scholar]
- Study of hyoscine solubility in scCO2: Experimental measurement and thermodynamic modeling. Journal of Molecular Liquids.. 2023;381:121821.
- [CrossRef] [Google Scholar]
- Measurement and thermodynamic modeling of solubility of Tamsulosin drug (anti cancer and anti-prostatic tumor activity) in supercritical carbon dioxide. The Journal of Supercritical Fluids.. 2020;163:104875.
- [CrossRef] [Google Scholar]
- Solubility of some statin drugs in supercritical carbon dioxide and representing the solute solubility data with several density-based correlations. The Journal of Supercritical Fluids.. 2007;41:187-194.
- [CrossRef] [Google Scholar]
- Solubility and thermodynamic modeling of sildenafil citrate in supercritical carbon dioxide. Fluid Phase Equilibria.. 2023;566:113677.
- [CrossRef] [Google Scholar]
- Study of the effects of methanol, ethanol and propanol alcohols as co-solvents on the interaction of methimazole, propranolol and phenazopyridine with carbon dioxide in supercritical conditions by molecular dynamics. The Journal of Supercritical Fluids.. 2018;140:91-100.
- [CrossRef] [Google Scholar]
- Phosphatidylcholine solubility in supercritical carbon dioxide: Experimental data, thermodynamic modeling, and application in bioactive-encapsulated liposome synthesis. The Journal of Supercritical Fluids.. 2020;158:104720.
- [CrossRef] [Google Scholar]
- An introduction to hydrogen bonding. New York: Oxford University Press; 1997.
- Mathematical representation of solute solubility in supercritical carbon dioxide using empirical expressions. The Journal of Supercritical Fluids.. 2002;24:19-35.
- [CrossRef] [Google Scholar]
- Solubility prediction in supercritical CO2 using minimum number of experiments. Journal of Pharmaceutical Sciences.. 2002;91:1287-1295.
- [CrossRef] [Google Scholar]
- Carbamazepine solubility in supercritical CO2: A comprehensive study. Journal of Molecular Liquids.. 2020;311:113104.
- [CrossRef] [Google Scholar]
- A crossover of the solid substances solubility in supercritical fluids: What is it in fact? Journal of Molecular Liquids.. 2021;334:115997.
- [CrossRef] [Google Scholar]
- Development and evaluation of a new semi-empirical model for correlation of drug solubility in supercritical CO2. Fluid Phase Equilibria.. 2014;363:18-26.
- [CrossRef] [Google Scholar]
- Representing solute solubility in supercritical carbon dioxide: A novel empirical model. Chemical Engineering Research and Design.. 2015;93:355-365.
- [CrossRef] [Google Scholar]
- Solid solubility measurement of haloperidol in supercritical carbon dioxide and nanonization using the rapid expansion of supercritical solutions process. The Journal of Supercritical Fluids.. 2023;192:105785.
- [CrossRef] [Google Scholar]
- Solubility of probenecid in supercritical carbon dioxide and composite particles prepared using supercritical antisolvent process. The Journal of Supercritical Fluids.. 2023;194:105851.
- [CrossRef] [Google Scholar]
- Solubility of solids in sub-and supercritical fluids: a review 2010–2017. Journal of Chemical & Engineering Data.. 2017;63:860-884.
- [CrossRef] [Google Scholar]
- Experimental data and modelling of the solubility of high-carotenoid paprika extract in supercritical carbon dioxide. Molecules.. 2019;24:4174.
- [CrossRef] [Google Scholar]
- Effects of renal impairment on the pharmacokinetics, pharmacodynamics and safety of rivaroxaban, an oral, direct Factor Xa inhibitor. British Journal of Clinical Pharmacology.. 2010;70:703-712.
- [CrossRef] [Google Scholar]
- Modelling the solubility of solids in supercritical fluids with density as the independent variable. The Journal of Supercritical Fluids.. 1988;1:15-22.
- [CrossRef] [Google Scholar]
- On Absorption Modeling and Food Effect Prediction of Rivaroxaban, a BCS II Drug Orally Administered as an Immediate-Release Tablet. Pharmaceutics.. 2021;13:283.
- [CrossRef] [Google Scholar]
- Determining the solubility of nifedipine and quinine in supercritical fluid carbon dioxide using continuously stirred static solubility apparatus interfaced with online supercritical fluid chromatography. Journal of Chemical & Engineering Data.. 2017;62:1530-1537.
- [CrossRef] [Google Scholar]
- Solubility of solid solutes in supercritical carbon dioxide with and without cosolvents. Fluid Phase Equilibria.. 2003;207:183-192.
- [CrossRef] [Google Scholar]
- Solubility of ionic liquid [Bmim] Ac in supercritical CO2 containing different cosolvents. Journal of Chemical & Engineering Data.. 2018;63:1596-1602.
- [CrossRef] [Google Scholar]
- Supercritical carbon dioxide for pharmaceutical co-crystal production. Crystal Growth & Design.. 2020;20:6226-6244.
- [CrossRef] [Google Scholar]
- Modelling of Solubility of Some Parabens in Supercritical Carbon Dioxide and New Correlations. Arabian Journal for Science and Engineering.. 2022;47:5533-5545.
- [CrossRef] [Google Scholar]
- Experimental measurement and thermodynamic modeling of Chlorothiazide solubility in supercritical carbon dioxide. Case Studies in Thermal Engineering.. 2023;41:102621.
- [CrossRef] [Google Scholar]
- Solubility of tolbutamide and chlorpropamide in supercritical carbon dioxide. Journal of Chemical & Engineering Data.. 2018;63:1745-1751.
- [CrossRef] [Google Scholar]
- Supercritical carbon dioxide separation of carboxylic acids and phenolics from bio-oil of lignocellulosic origin: understanding bio-oil compositions, compound solubilities, and their fractionation. Industrial & Engineering Chemistry Research.. 2017;56:3129-3144.
- [CrossRef] [Google Scholar]
- In vitro antimicrobial, physicochemical, pharmacokinetics and molecular docking studies of benzoyl uridine esters against SARS-CoV-2 main protease. Journal of Biomolecular Structure and Dynamics.. 2022;40:3668-3680.
- [CrossRef] [Google Scholar]
- The solubility of solids in supercritical fluids. Fluid Phase Equilibria.. 1999;158:501-510.
- [CrossRef] [Google Scholar]
- Clinical pharmacokinetic and pharmacodynamic profile of rivaroxaban. Clinical Pharmacokinetics.. 2014;53:1-16.
- [CrossRef] [Google Scholar]
- Solubility of vitamin A in supercritical CO2: experimental study and thermodynamic modeling. Scientific Reports.. 2021;11:1-13.
- [CrossRef] [Google Scholar]
- Production of Rosuvastatin Calcium Nanoparticles Using Gas Antisolvent Technique: Experimental and Optimization. Periodica Polytechnica. Chemical Engineering.. 2021;65:442-453.
- [CrossRef] [Google Scholar]
- A machine learning approach for thermodynamic modeling of the statically measured solubility of nilotinib hydrochloride monohydrate (anti-cancer drug) in supercritical CO2. Scientific Reports.. 2023;13:12906.
- [CrossRef] [Google Scholar]
- Increasing solubility of phenytoin and raloxifene drugs: Application of supercritical CO2 technology. Journal of Molecular Liquids.. 2023;373:121246.
- [CrossRef] [Google Scholar]
- Solubility of cefuroxime axetil in supercritical CO2: Measurement and modeling. The Journal of Supercritical Fluids.. 2019;152:104498.
- [CrossRef] [Google Scholar]
- Supercritical carbon dioxide-based technologies for the production of drug nanoparticles/nanocrystals–a comprehensive review. Advanced Drug Delivery Reviews.. 2018;131:22-78.
- [CrossRef] [Google Scholar]
- Rivaroxaban versus warfarin in nonvalvular atrial fibrillation. New England Journal of Medicine.. 2011;365:883-891.
- [CrossRef] [Google Scholar]
- A new two-constant equation of state. Industrial & Engineering Chemistry Fundamentals.. 1976;15:59-64.
- [CrossRef] [Google Scholar]
- Polar Hard-Core Exponential-6 Intermolecular Potential Function for Determining the Thermodynamic Properties of Polar Gases. Industrial & Engineering Chemistry Research.. 2020;59:14106-14114.
- [CrossRef] [Google Scholar]
- Thermodynamic modelling and experimental validation of pharmaceutical solubility in supercritical solvent. Journal of Molecular Liquids.. 2020;319:114120.
- [CrossRef] [Google Scholar]
- Measuring solubility of a chemotherapy-anti cancer drug (busulfan) in supercritical carbon dioxide. Journal of Molecular Liquids.. 2020;317:113954.
- [CrossRef] [Google Scholar]
- Using static method to measure tolmetin solubility at different pressures and temperatures in supercritical carbon dioxide. Scientific Reports.. 2020;10:1-7.
- [CrossRef] [Google Scholar]
- Chloroquine (antimalaria medication with anti SARS-CoV activity) solubility in supercritical carbon dioxide. Journal of Molecular Liquids.. 2021;322:114539.
- [CrossRef] [Google Scholar]
- Experimental and thermodynamic modeling decitabine anti cancer drug solubility in supercritical carbon dioxide. Scientific Reports.. 2021;11:1-8.
- [CrossRef] [Google Scholar]
- Solubility of dialkylalkyl phosphonates in supercritical carbon dioxide: Experimental and modeling approach. Fluid Phase Equilibria.. 2017;435:88-97.
- [CrossRef] [Google Scholar]
- Solubility of trioctylmethylammonium chloride in supercritical carbon dioxide and the influence of co-solvents on the solubility behavior. The Journal of Supercritical Fluids.. 2018;138:102-114.
- [CrossRef] [Google Scholar]
- Molecular Thermodynamics of Fluid Phase Equilibria (3rd edn). Englewood Cliffs: Prentice Hall PTR; 1999.
- A new semi-empirical model for correlating the solubilities of solids in supercritical carbon dioxide with cosolvents. Fluid Phase Equilibria.. 2011;310:207-212.
- [CrossRef] [Google Scholar]
- Improving and measuring the solubility of favipiravir and montelukast in SC-CO 2 with ethanol projecting their nanonization. RSC Advances.. 2023;13:34210-34223.
- [CrossRef] [Google Scholar]
- Determination of Anthraquinone Violet 3RN solubility in supercritical carbon dioxide with/without co-solvent: Experimental data and modeling (empirical and thermodynamic models) Chemical Engineering Research and Design.. 2020;159:529-542.
- [CrossRef] [Google Scholar]
- Solubility of famotidine in supercritical carbon dioxide: Experimental measurement and thermodynamic modeling. The Journal of Supercritical Fluids.. 2023;201:106031.
- [CrossRef] [Google Scholar]
- Experimental analysis and thermodynamic modelling of lenalidomide solubility in supercritical carbon dioxide. Arabian Journal of Chemistry.. 2022;15:103821.
- [CrossRef] [Google Scholar]
- Solubility of favipiravir (as an anti-COVID-19) in supercritical carbon dioxide: An experimental analysis and thermodynamic modeling. The Journal of Supercritical Fluids.. 2022;183:105539.
- [CrossRef] [Google Scholar]
- Solubility of montelukast (as a potential treatment of COVID -19) in supercritical carbon dioxide: Experimental data and modelling. Journal of Molecular Liquids.. 2022;349:118219.
- [CrossRef] [Google Scholar]
- Using the supercritical carbon dioxide as the solvent of Nystatin: Studying the effect of co-solvent, experimental and correlating. The Journal of Supercritical Fluids.. 2023;194:105858.
- [CrossRef] [Google Scholar]
- Laboratory assessment of rivaroxaban: a review. Thrombosis Journal.. 2013;11:1-7.
- [CrossRef] [Google Scholar]
- Enhanced density-based models for solid compound solubilities in supercritical carbon dioxide with cosolvents. Fluid Phase Equilibria.. 2003;208:99-113.
- [CrossRef] [Google Scholar]
- Spectrophotometric quantification of direct factor xa inhibitor, rivaroxaban, in raw and tablet dosage form. Glob Drugs and Therap.. 2017;2:1-8.
- [CrossRef] [Google Scholar]
- Solubility determination and correlation of gatifloxacin, enrofloxacin, and ciprofloxacin in supercritical CO2. Journal of Chemical & Engineering Data.. 2017;62:4235-4243.
- [CrossRef] [Google Scholar]
- Equilibrium constants from a modified Redlich-Kwong equation of state. Chemical Engineering Science.. 1972;27:1197-1203.
- [CrossRef] [Google Scholar]
- Solubility measurement of a chemotherapeutic agent (Imatinib mesylate) in supercritical carbon dioxide: Assessment of new empirical model. The Journal of Supercritical Fluids.. 2019;146:89-99.
- [CrossRef] [Google Scholar]
- Solubility measurement of a pigment (Phthalocyanine green) in supercritical carbon dioxide: Experimental correlations and thermodynamic modeling. Fluid Phase Equilibria.. 2019;494:61-73.
- [CrossRef] [Google Scholar]
- Solubility of 2, 4, 7-Triamino-6-phenylpteridine (Triamterene, Diuretic Drug) in supercritical carbon dioxide: experimental data and modeling. Journal of Chemical & Engineering Data.. 2020;65:4406-4416.
- [CrossRef] [Google Scholar]
- Experimental data and thermodynamic modeling of solubility of Azathioprine, as an immunosuppressive and anti-cancer drug, in supercritical carbon dioxide. Journal of Molecular Liquids.. 2020;299:112179.
- [CrossRef] [Google Scholar]
- Prediction of solubility of sunitinib malate (an anti-cancer drug) in supercritical carbon dioxide (SC–CO2): experimental correlations and thermodynamic modeling. Journal of Molecular Liquids.. 2020;297:111740.
- [CrossRef] [Google Scholar]
- Experimental data and thermodynamic modeling of solubility of Sorafenib tosylate, as an anti-cancer drug, in supercritical carbon dioxide: Evaluation of Wong-Sandler mixing rule. The Journal of Chemical Thermodynamics.. 2020;142:105998.
- [CrossRef] [Google Scholar]
- Experimental and thermodynamic analyses of supercritical CO2-Solubility of minoxidil as an antihypertensive drug. Fluid Phase Equilibria.. 2020;522:112745.
- [CrossRef] [Google Scholar]
- Solubility measurement and preparation of nanoparticles of an anticancer drug (Letrozole) using rapid expansion of supercritical solutions with solid cosolvent (RESS-SC) The Journal of Supercritical Fluids.. 2018;133:239-252.
- [CrossRef] [Google Scholar]
- Determination of solubility of Aprepitant (an antiemetic drug for chemotherapy) in supercritical carbon dioxide: empirical and thermodynamic models. The Journal of Supercritical Fluids.. 2017;128:102-111.
- [CrossRef] [Google Scholar]
- Solubility of an antiarrhythmic drug (amiodarone hydrochloride) in supercritical carbon dioxide: experimental and modeling. Fluid Phase Equilibria.. 2017;450:149-159.
- [CrossRef] [Google Scholar]
- Measurement, correlation and thermodynamic modeling of the solubility of Ketotifen fumarate (KTF) in supercritical carbon dioxide: evaluation of PCP-SAFT equation of state. Fluid Phase Equilibria.. 2018;458:102-114.
- [CrossRef] [Google Scholar]
- Solubility measurement of an antihistamine drug (Loratadine) in supercritical carbon dioxide: Assessment of qCPA and PCP-SAFT equations of state. Fluid Phase Equilibria.. 2018;472:147-159.
- [CrossRef] [Google Scholar]
- Experimental measurements and thermodynamic modeling of Coumarin-7 solid solubility in supercritical carbon dioxide: Production of nanoparticles via RESS method. Fluid Phase Equilibria.. 2019;483:122-143.
- [CrossRef] [Google Scholar]
- Experimental study and thermodynamic modeling of Esomeprazole (proton-pump inhibitor drug for stomach acid reduction) solubility in supercritical carbon dioxide. The Journal of Supercritical Fluids.. 2019;154:104606.
- [CrossRef] [Google Scholar]
- Experimental investigation and modeling of the solubility of oxcarbazepine (an anticonvulsant agent) in supercritical carbon dioxide. Fluid Phase Equilibria.. 2019;493:160-173.
- [CrossRef] [Google Scholar]
- Determination of the solubility of the repaglinide drug in supercritical carbon dioxide: Experimental data and thermodynamic modeling. Journal of Chemical & Engineering Data.. 2019;64:5338-5348.
- [CrossRef] [Google Scholar]
- Prediction of solubility of sodium valproate in supercritical carbon dioxide: experimental study and thermodynamic modeling. Journal of Chemical & Engineering Data.. 2020;65:1747-1760.
- [CrossRef] [Google Scholar]
- Experimental measurement and thermodynamic modeling of Lansoprazole solubility in supercritical carbon dioxide: Application of SAFT-VR EoS. Fluid Phase Equilibria.. 2020;507:112422.
- [CrossRef] [Google Scholar]
- Solubility of Quetiapine hemifumarate (antipsychotic drug) in supercritical carbon dioxide: Experimental, modeling and Hansen solubility parameter application. Fluid Phase Equilibria.. 2021;537:113003.
- [CrossRef] [Google Scholar]
- Measurement and modeling of clemastine fumarate (antihistamine drug) solubility in supercritical carbon dioxide. Scientific Reports.. 2021;11:24344.
- [CrossRef] [Google Scholar]
- Solubility of amlodipine besylate (calcium channel blocker drug) in supercritical carbon dioxide: Measurement and correlations. Journal of Chemical & Engineering Data.. 2021;66:1119-1131.
- [CrossRef] [Google Scholar]
- The solubility of Sulfabenzamide (an antibacterial drug) in supercritical carbon dioxide: Evaluation of a new thermodynamic model. Journal of Molecular Liquids.. 2021;335:116446.
- [CrossRef] [Google Scholar]
- Determination of Galantamine solubility (an anti-alzheimer drug) in supercritical carbon dioxide (CO2): Experimental correlation and thermodynamic modeling. Journal of Molecular Liquids.. 2021;330:115695.
- [CrossRef] [Google Scholar]
- Measuring and modeling the solubility of an antihypertensive drug (losartan potassium, Cozaar) in supercritical carbon dioxide. Journal of Molecular Liquids.. 2021;331:115745.
- [CrossRef] [Google Scholar]
- Solubility of Ketoconazole (antifungal drug) in SC-CO2 for binary and ternary systems: Measurements and empirical correlations. Scientific Reports.. 2021;11:1-13.
- [CrossRef] [Google Scholar]
- Solubility of Pholcodine (antitussive drug) in supercritical carbon dioxide: Experimental data and thermodynamic modeling. Fluid Phase Equilibria.. 2022;556:113396.
- [CrossRef] [Google Scholar]
- Solubility of pazopanib hydrochloride (PZH, anticancer drug) in supercritical CO2: Experimental and thermodynamic modeling. The Journal of Supercritical Fluids.. 2022;190:105759.
- [CrossRef] [Google Scholar]
- Experimental solubility and thermodynamic modeling of empagliflozin in supercritical carbon dioxide. Scientific Reports.. 2022;12:9008.
- [CrossRef] [Google Scholar]
- Solubility measurement and thermodynamic modeling of pantoprazole sodium sesquihydrate in supercritical carbon dioxide. Scientific Reports.. 2022;12:7758.
- [CrossRef] [Google Scholar]
- Experimental solubility and modeling of Crizotinib (anti-cancer medication) in supercritical carbon dioxide. Scientific Reports.. 2022;12:1-16.
- [CrossRef] [Google Scholar]
- Measurement and modeling of metoclopramide hydrochloride (anti-emetic drug) solubility in supercritical carbon dioxide. Arabian Journal of Chemistry.. 2022;103876
- [CrossRef] [Google Scholar]
- CO2 utilization for determining solubility of teriflunomide (immunomodulatory agent) in supercritical carbon dioxide: Experimental investigation and thermodynamic modeling. Journal of CO2 Utilization.. 2022;58:101931.
- [CrossRef] [Google Scholar]
- Solubility of Dasatinib monohydrate (anticancer drug) in supercritical CO2: Experimental and thermodynamic modeling. Journal of Molecular Liquids.. 2022;346:117899.
- [CrossRef] [Google Scholar]
- Solubility of prazosin hydrochloride (alpha blocker antihypertensive drug) in supercritical CO2: Experimental and thermodynamic modelling. Journal of Molecular Liquids.. 2022;362:119689.
- [CrossRef] [Google Scholar]
- Solubility of buprenorphine hydrochloride in supercritical carbon dioxide: Study on experimental measuring and thermodynamic modeling. Arabian Journal of Chemistry.. 2023;16:105196.
- [CrossRef] [Google Scholar]
- Solubility measurement and modeling of hydroxychloroquine sulfate (antimalarial medication) in supercritical carbon dioxide. Scientific Reports.. 2023;13:8112.
- [CrossRef] [Google Scholar]
- Solubility of palbociclib in supercritical carbon dioxide from experimental measurement and Peng-Robinson equation of state. Scientific Reports.. 2023;13:2172.
- [CrossRef] [Google Scholar]
- Solubility of ibrutinib in supercritical carbon dioxide (Sc-CO2): Data correlation and thermodynamic analysis. The Journal of Chemical Thermodynamics.. 2023;182:107050.
- [CrossRef] [Google Scholar]
- Determination of the solubility of rivaroxaban (anticoagulant drug, for the treatment and prevention of blood clotting) in supercritical carbon dioxide: Experimental data and correlations. Arabian Journal of Chemistry.. 2023;16:104421.
- [CrossRef] [Google Scholar]
- A new empirical model to correlate solute solubility in supercritical carbon dioxide in presence of co-solvent. Chemical Engineering Research and Design.. 2017;125:79-87.
- [CrossRef] [Google Scholar]
- A new equation of state for carbon dioxide covering the fluid region from the triple-point temperature to 1100 K at pressures up to 800 MPa. Journal of Physical and Chemical Reference Data.. 1996;25:1509-1596.
- [CrossRef] [Google Scholar]
- Evaluation of density-based models for the solubility of solids in supercritical carbon dioxide and formulation of a new model. Chemical Engineering Science.. 2008;63:4292-4301.
- [CrossRef] [Google Scholar]
- A new model for the solubility of dye compounds in supercritical carbon dioxide. Thermochimica Acta.. 2013;561:91-97.
- [CrossRef] [Google Scholar]
- Solubility of CI disperse red 60 and CI disperse blue 60 in supercritical carbon dioxide. Journal of Chemical & Engineering Data.. 1999;44:985-989.
- [CrossRef] [Google Scholar]
- A comparison between semiempirical equations to predict the solubility of pharmaceutical compounds in supercritical carbon dioxide. The Journal of Supercritical Fluids.. 2010;52:161-174.
- [CrossRef] [Google Scholar]
- Solubility of 1-aminoanthraquinone and 1-nitroanthraquinone in supercritical carbon dioxide. The Journal of Chemical Thermodynamics.. 2017;104:162-168.
- [CrossRef] [Google Scholar]
- Rivaroxaban: an oral factor Xa inhibitor. Clinical Therapeutics.. 2013;35:4-27.
- [CrossRef] [Google Scholar]
- Phase equilibrium and micronization for flufenamic acid with supercritical carbon dioxide. Journal of the Taiwan Institute of Chemical Engineers.. 2017;72:19-28.
- [CrossRef] [Google Scholar]
- Experimental-Theoretical approach for determination of Metformin solubility in supercritical carbon dioxide: Thermodynamic modeling. Case Studies in Thermal Engineering.. 2022;102649
- [CrossRef] [Google Scholar]
- Measurement and modeling of solubility of gliclazide (hypoglycemic drug) and captopril (antihypertension drug) in supercritical carbon dioxide. The Journal of Supercritical Fluids.. 2021;174:105244.
- [CrossRef] [Google Scholar]
- Solid solubility measurement of ipriflavone in supercritical carbon dioxide and microparticle production through the rapid expansion of supercritical solutions process. Journal of CO2 Utilization.. 2020;37:285-294.
- [CrossRef] [Google Scholar]
- Solubility measurement and RESOLV-assisted nanonization of gambogic acid in supercritical carbon dioxide for cancer therapy. The Journal of Supercritical Fluids.. 2019;150:147-155.
- [CrossRef] [Google Scholar]
- Comparison of Four Density-Based Semi-Empirical Models for the Solubility of Azo Disperse Dyes in Supercritical Carbon Dioxide. Processes.. 2022;10:1960.
- [CrossRef] [Google Scholar]
- Solubility measurement method and mathematical modeling in supercritical fluids. Engineering Journal.. 2013;17:67-78.
- [CrossRef] [Google Scholar]
- Solubilities of fatty acids, fatty acid esters, triglycerides, and fats and oils in supercritical carbon dioxide. The Journal of Supercritical Fluids.. 1994;7:51-59.
- [CrossRef] [Google Scholar]
- Loxoprofen solubility in supercritical carbon dioxide: experimental and modeling approaches. Journal of Chemical & Engineering Data.. 2020;65:4613-4620.
- [CrossRef] [Google Scholar]
- Experimental solubility measurements of fenoprofen in supercritical carbon dioxide. Journal of Chemical & Engineering Data.. 2020;65:1425-1434.
- [CrossRef] [Google Scholar]
- Measuring salsalate solubility in supercritical carbon dioxide: experimental and thermodynamic modelling. The Journal of Chemical Thermodynamics.. 2021;152:106271.
- [CrossRef] [Google Scholar]
- Thermodynamic study on solubility of brain tumor drug in supercritical solvent: Temozolomide case study. Journal of Molecular Liquids.. 2021;321:114926.
- [CrossRef] [Google Scholar]
- Experimental measurement and correlation of solubility of ethosuximide in supercritical carbon dioxide. The Journal of Chemical Thermodynamics.. 2019;131:104-110.
- [CrossRef] [Google Scholar]
- Measurement and correlation of curcumin solubility in supercritical carbon dioxide. Journal of Chemical & Engineering Data.. 2017;62:1257-1263.
- [CrossRef] [Google Scholar]
- Experimental Determination and Association Model for the Solubility of Laminarin in Supercritical Carbon Dioxide. Journal of Chemical & Engineering Data.. 2020;65:1814-1823.
- [CrossRef] [Google Scholar]
Appendix A
Supplementary data
Supplementary data to this article can be found online at https://doi.org/10.1016/j.arabjc.2024.105707.
Appendix A
Supplementary data
The following are the Supplementary data to this article:Supplementary Data 1
Supplementary Data 1