Biosorption of lead (II) from aqueous solution using Cellulose-based Bio-adsorbents prepared from unripe papaya (Carica papaya) peel waste: Removal Efficiency, Thermodynamics, kinetics and isotherm analysis
⁎Corresponding authors. ura.p@chula.ac.th (Ura Pancharoen), kasidit.n@chula.ac.th (Kasidit Nootong)
-
Received: ,
Accepted: ,
This article was originally published by Elsevier and was migrated to Scientific Scholar after the change of Publisher.
Peer review under responsibility of King Saud University.
Abstract
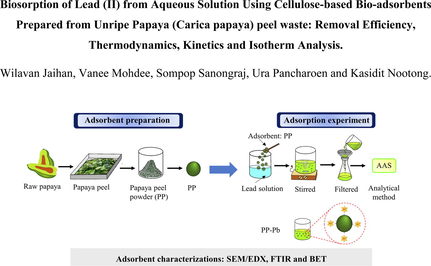
Abstract
This work focuses on the removal of lead from contaminated aqueous solutions using unripe papaya peel based bio-adsorbents (PP). Response surface methodology (RSM) based on Box-Behnken design (BBD) is employed to determine the independent variables. Optimum conditions proved to be 96.5 mg/L of initial lead concentration in solution, at pH 4 of aqueous solution, having adsorbent dosage of 14.6 g/L and contact time (3 h) which subsequently yielded the predicted and actual lead removal efficiencies of 100% and 97.54%, respectively. Adsorption isotherms and kinetics of lead adsorption using unripe papaya peel followed the Freundlich and pseudo-second-order models, indicating that the process of chemisorption occurred. The magnitude of the adsorption capacity of the pseudo-second-order model (qe,cal = 6.25 mg/g) was found to be comparable to the value obtained experimentally (qe,cal = 6.45 mg/g). Thermodynamic parameters were calculated in order to identify the phenomena of adsorption. The values of ΔH° and ΔS° are found to be 13.61 J/mol and 54.30 J/mol⋅K, respectively. The characteristics of unripe papaya peel bio-adsorbents, analyzed via SEM/EDX, FTIR and BET, are also presented. Thus, the O-H and C-O functional groups contained in the unripe papaya peel waste were found to effectively adsorb lead from the aqueous medium. The average pore diameters, average pore volumes and average surface area of bio-adsorbents prepared from unripe papaya peel waste proved to be 9.046 nm, 0.0012 cm3/g and 0.755 m2/g, respectively.
Keywords
Lead
Papaya
Adsorption
Wastewater
RSM
1 Introduction
Lead (Pb), a soft blue-gray metallic element, has been widely used in diverse industrial areas such as paints, batteries, leaded glasses, fuels, pigments, photographic materials, petrochemicals and mining etc. (Suren et al., 2014). Lead is considered to be a cumulative poison and has been found to be hazardous at high levels. Due to its toxicity, lead can cause damage to the digestive tract, immune system, human circulatory system, liver and kidney (Ghaedi et al., 2018; Yu et al., 2020). In Thailand, lead contamination in aquatic systems has been observed in many places (Mingkhwan and Worakhunpiset, 2018; Kladsomboon et al., 2020; Kosanlavit, 2021). Thus, its contamination has been become a serious problem. Various methods, such as coagulation-flocculation, liquid–liquid extraction, ion exchange and electrochemical treatment, have been employed to separate lead from aqueous solutions (Naeem et al., 2009; Pang et al., 2011; Liu et al., 2013). However, the drawbacks of these techniques include high operating costs, time consuming, difficulty in scaling up and the generation of a large volume of toxic sludge (Abbaszadeh et al., 2016; Ariffin et al., 2017; Wu et al., 2011a,b).
Adsorption has been reported as a green and economical method for lead removal in aqueous systems (Li et al., 2019; Fu et al., 2021). It is considered to be a suitable process for the selective binding of metal at low concentration (Li et al., 2019). Recently, bio-based adsorbents from agricultural wastes e.g. potato peel, cucumber peel and banana peel have received heightened interest with respect to the removal of heavy metals from contaminated wastewater (Ali et al., 2016; Ramakul et al., 2012; Basu et al., 2017; Amin et al., 2018; Arampatzidou and Deliyanni, 2016). Such attention is owing to the presence of carboxyl and hydroxyl functional groups in lignocellulose, which play an important role in improving adsorption efficiency (Basu et al., 2017; Amin et al., 2018; Dawn and Vishwakarma, 2021; Arampatzidou and Deliyanni, 2016). Thus, bio-based adsorbents prepared from agricultural wastes are considered to be promising alternative materials and efficient adsorbents for target analysis on account of its high adsorption capacity, renewability, low-cost and eco-friendly properties. (Li et al., 2017; Wang et al., 2016).
Papaya (Carica papaya) is a tropical fruit cultivated widely in Southeast Asia, Central America, and tropical islands. Papaya is popular both as a food and juice. Papaya production in Thailand is about 1.3 to 2.0 ktons annually; approximately 90% of the total production is consumed domestically. Market surveys also indicate that roughly 85% to 90% of the domestic papaya is consumed as green (unripen) papaya for the popular dish called “somtum” (Srisompun et al., 2018). Such consumption leads to a massive amount of papaya peel waste to be managed. Many researchers have been investigated the removal of heavy metals via biosorption process using different parts of papaya waste e.g. seeds, leaves and peel as an adsorbent (Shooto and Naidoo, 2019; Raju et al., 2013; Abbaszadeh et al., 2015). It is noted that the use of papaya peel as bio-adsorbent for lead removal has been, to date, limited only to ripe papaya which results of these experiments have proved unsatisfactory. The percentages of lead removal using ripe papaya have been found to be in the range of 80–93%, such values are low in comparison to those obtained when using other parts of papaya (Abbaszadeh et al., 2015; Adie Gilbert et al., 2011; Raju et al., 2012). To the best of our knowledge, an investigation as regards the use of unripe papaya peel for lead removal in aqueous systems has not been carried out before.
Response surface methodology (RSM) is an effective statistical technique for evaluating optimum conditions of diverse processes, including adsorption (Singh and Bhateria, 2020; Davarnejad et al., 2018). Box-Behnken design (BBD) has also been found to be suitable for three or more variables. Herein, it has been applied to achieve the most accurate prediction results (Singh and Bhateria, 2020; Chowdhury et al., 2016; Uçurum et al., 2018). Singh and Bhateria (2020) applied BBD for evaluating the interactive effect of five variables on lead ion adsorption using Fe3O4NPs as adsorbents. Many works have successfully evaluated optimized conditions having four variables using BBD e.g. copper adsorption (Chowdhury et al., 2016) and zinc adsorption (Uçurum et al., 2018). RSM based on BBD has proved to be a feasible and competent method for optimization of adsorption parameters and minimizing the number of experiments.
This work intends to evaluate the performance of unripe papaya peel as the bio-based adsorbents (PP) to remove lead from aqueous solution. RSM based on BBD is therefore, applied to determine the optimal adsorption conditions. Moreover, independent variables are evaluated viz. (i) initial lead concentration, (ii) pH of feed solution, (iii) adsorbent dosage and (iv) contact time. Various techniques are carried out in order to examine the as prepared bio-adsorbents characteristics i.e. SEM/EDX, BET and FTIR. Isotherms, kinetics and thermodynamics of lead adsorption are analyzed to explain the mechanisms involved.
2 Materials and methods
2.1 Chemicals
Lead (II) nitrate solution (Pb(NO3)2) was used to prepare the aqueous lead solution. Nitric acid (HNO3) and sodium hydroxide (NaOH) were used to adjust the acidity-basicity of solutions. All solutions were prepared using distilled water (Lee Cier Huad Ltd., Part.). All chemicals are of analytical grade and used without further purification. Detailed descriptions of chemicals used are presented in Table 1.
Chemical name | Molecular formular |
Molecular weight (g/mol) | Purity (wt%) |
Source |
---|---|---|---|---|
Lead (II) nitrate solution | Pb(NO3)2 | 331.20 | 1000.0a | PanReac Applichem |
Nitric acid | HNO3 | 63.013 | 65.0 | Merck |
Sodium hydroxide | NaOH | 39.997 | 98.0 | Ajax Finechem |
Note: a is the concentration of lead ion in a unit of mg/L.
*The physical properties data are from chemical bottle labels.
2.2 Adsorbent preparation
First, green (unripe) papayas were obtained from the local market in Bangkok. Then, the papaya peel was removed from the fruit and washed with distilled water to remove dirt. Next, the peel was chopped into small pieces and dried at 343 K in an oven until the weight was constant. The dried papaya peel was subsequently crushed into powder using a mortar and pestle and then sieved with a 40-mesh screen to obtain the papaya peel based bio-adsorbents (PP). In Fig. 1, a schematic diagram of unripe papaya peel based bio-adsorbents preparation is depicted.

- Schema of papaya based bio-adsorbent (PP) preparation.
2.3 Adsorbent characterization
2.3.1 SEM/EDx
Scanning electron microscope (SEM-JEOL, JSM-IT300) coupled with energy-dispersive x-ray spectrum (EDX-Oxford, X-MaxN20) were carried out to study the morphology and analyze the elemental composition of the PP, before and after adsorption of lead. Before scanning, all samples were dried and coated with gold to enhance electron conductivity. The micrographs were examined at 150X magnification.
2.3.2 BETa
The Brunauer-Emmett-Teller (BET- Micromeritics, 3Flex) method was used to determine the specific surface area and pore volume of PP. The relative pressure (p/p0) was 0.004–0.99 bar and 0.99–0.10 bar for adsorption and desorption, respectively. Temperature of the bath was kept at 77.376 K, in liquid N2. Degassing temperature was carried out at 60 ⁰C for 1,440 min.
2.3.3 FTIRa
Fourier transform infrared spectroscopy (FTIR- Bruker, INVENIO-S) was employed to characterize the functional groups of PP, before and after lead adsorption. Samples were analyzed via the ATR diamond method. The measurement wavelength was in the range of 400–4000 cm−1.
2.4 Lead adsorption using PP
The lead adsorption experiment was conducted using unripe papaya peel as adsorbent. Synthetic wastewater was prepared by dissolving analytical-graded of Pb(NO3)2 in distilled water; the solution contained lead from 10 to 100 mg/L. The conditions of pH (4 to 6), adsorbent dosage (0.1 to 1.0 g per 50 mL of solution) and contact time (1 to 3 h) were used during the experiment. Firstly, lead solution (50 mL) and adsorbents were mixed in 100 mL flasks using a magnetic stirrer bar at 200 rpm, for the given contact time, at room temperature (303 K). Then, the solution was filtered using Whatman filtered paper with an average pore size of 2.5 μm. The amount of lead in the solution was measured via atomic absorption spectrometry (AAS-AGILENT 280FS AA). In Fig. 2, a schematic diagram of lead adsorption procedures is depicted. Both adsorption capacity (qe, mg/g) and lead removal efficiency (E, %) were calculated, according to Eqs. (1) and (2), respectively (Abbaszadeh et al., 2016):
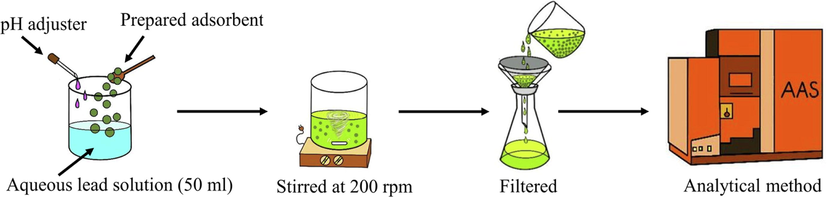
- Schema of lead adsorption procedure for PP.
2.5 Optimization of process parameters
RSM based BBD by Minitab 17 software was applied to optimize the process of lead adsorption using unripe papaya peel as bio-adsorbents. Four independent variables were investigated, including the initial lead concentration in the solution (X1), pH of feed solution (X2), adsorbent dosage (X3) and contact time (X4). As illustrated in Table 2, three levels were examined (-1, 0 + 1). In Table 3, the experimental conditions, including 3 repetitions at the center points, are demonstrated. The lead removal efficiency as the response variable (Y) was fitted via a second-order polynomial model, as in Eq.(3):
Parameter | Code | Level | ||
---|---|---|---|---|
−1 | 0 | 1 | ||
Initial lead concentration (mg/L) | X1 | 10 | 55 | 100 |
pH of feed solution | X2 | 4 | 5 | 6 |
Adsorbent dosage (g/50 mL) | X3 | 0.1 | 0.55 | 1 |
Contact time (h) | X4 | 1 | 2 | 3 |
Run | Experimental Condition | Experimental Condition | |||||||
---|---|---|---|---|---|---|---|---|---|
X1 | X2 | X3 | X4 | Run | X1 | X2 | X3 | X4 | |
1 | 10 | 4 | 0.55 | 2 | 15 | 55 | 4 | 1 | 2 |
2 | 100 | 4 | 0.55 | 2 | 16 | 55 | 6 | 1 | 2 |
3 | 10 | 6 | 0.55 | 2 | 17 | 10 | 5 | 0.1 | 2 |
4 | 100 | 6 | 0.55 | 2 | 18 | 100 | 5 | 0.1 | 2 |
5 | 55 | 5 | 0.1 | 1 | 19 | 10 | 5 | 1 | 2 |
6 | 55 | 5 | 1 | 1 | 20 | 100 | 5 | 1 | 2 |
7 | 55 | 5 | 0.1 | 3 | 21 | 55 | 4 | 0.55 | 1 |
8 | 55 | 5 | 1 | 3 | 22 | 55 | 6 | 0.55 | 1 |
9 | 10 | 5 | 0.55 | 1 | 23 | 55 | 4 | 0.55 | 3 |
10 | 100 | 5 | 0.55 | 1 | 24 | 55 | 6 | 0.55 | 3 |
11 | 10 | 5 | 0.55 | 3 | 25 | 55 | 5 | 0.55 | 2 |
12 | 100 | 5 | 0.55 | 3 | 26 | 55 | 5 | 0.55 | 2 |
13 | 55 | 4 | 0.1 | 2 | 27 | 55 | 5 | 0.55 | 2 |
14 | 55 | 6 | 0.1 | 2 |
2.6 Isotherm study
The isotherm study was conducted by varying the initial concentration of lead in synthetic wastewater from 10 to 110 mg/L, under optimized conditions obtained from RSM. The experiment was performed by adding PP into 100 mL flasks containing lead solution (50 mL). Then, the contents were mechanically stirred at 200 rpm for a given time at room temperature (303 K). Next, the solutions were filtered using Whatman filtered paper having an average pore size of 2.5 μm. The amount of lead in solution, before and after adsorption, was measured using AAS.
The data obtained from the experiment were linearly fitted for the Langmuir, Freundlich, Temkin, and Dubinin-Radushkevich (D-R) isotherms as described as follows:
2.6.1 Langmuir isotherm
The Langmuir isotherm quantitatively describes the monolayer adsorption on the outer surface of the adsorbent. No further adsorption takes place after the site was occupied; the adsorbate cannot permeate through the surface. The Langmuir isotherm can be expressed as follows (Dada et al., 2012):
2.6.2 Freundlich isotherm
The Freundlich isotherm is an empirical adsorption model, which can be applied to nonideal biosorption on heterogeneous surfaces as well as multilayer sorption. The isotherm is displayed as shown in Eq.(5) (Varank et al., 2012; Nandiyanto et al., 2020):
2.6.3 Temkin isotherm
The Temkin isotherm contains a factor that explicitly takes into account adsorbent-adsorbate interactions. As implied in the equation, its derivation is characterized by uniform distribution of binding energies, and is expressed in Eq. (6) (Dada et al., 2012):
2.6.4 Dubinin-Radushkevich (D-R) isotherm
The last isotherm is the D-R model, which describes the adsorption energy between adsorbate and adsorbent in terms of the adsorption potential (ε). The D-R isotherm model can be described by Eqs. (7) to (9) (Piccin et al., 2011):
2.7 Kinetics study
In order to clarify the reaction order and rate controlling mechanism, the following linear kinetic models were employed.
2.7.1 Pseudo-first-order
The pseudo-first-order kinetic model assumes that the number of sites that solutes can occupy is proportional to the rate of adsorption. The model is written as in Eq.(10) (Abbaszadeh et al., 2016):
2.7.2 Pseudo-second-order
The pseudo-second-order kinetic model involves chemisorption, where the removal of adsorbate from bulk liquid is due to the physiochemical interaction between adsorbent and adsorbate (El-Naas and Alhaija, 2013). The adsorption rate is also related to the availability of active sites on the adsorbent (Ali et al., 2016). The pseudo-second-order kinetic model is shown as in Eq. (11) (Varank et al., 2012):
2.7.3 Intraparticle diffusion
As for the intraparticle diffusion model, it is noted that intraparticle diffusion transport is the slowest step of adsorption process between adsorbate and adsorbent, as compared to film diffusion and pore diffusion (Ghasemi et al., 2014). The intraparticle diffusion model can be written as in Eq. (12) (Varank et al., 2012):
2.8 Thermodynamic study
The thermodynamic study of the lead adsorption process was carried out at 303, 323 and 343 K; the initial concentration of lead in the solution, pH of solution, dosage of adsorbent and contact time were maintained according to the optimal conditions via RSM. The change in the standard Gibbs free energy (ΔG°) for the adsorption process can be calculated, as in Eq. (13) (Abbaszadeh et al., 2016):
Eq. (14) is the Van’t Hoff equation in linear form. Thus, plotting ln kc versus 1/T yielded a straight line, whose slope and y-intercept yielded ΔS° and ΔH°, respectively.
2.9 Desorption experiments
The process of desorption for the unripe papaya peel bio-adsorbents was accomplished by varying the concentration of HNO3 at 0.1, 0.5, 1, 3 and 5 M (Chatterjee and Abraham, 2019). After the adsorption process, the adsorbed sample was mixed with HNO3 for 3 h and then washed with distilled water five times. Thereafter, the sample was dried at 343 K in an oven until the weight was constant. The amount of desorbed lead can be calculated as in Eq.(15) (Abbaszadeh et al., 2016):
3 Results and discussion
3.1 Characterization of adsorbent
3.1.1 SEM/ EDx
Fig. 3 displays SEM images of unripe papaya peel bio-adsorbents before (PP) and after lead adsorption (PP-Pb). Before adsorption, PP was a bulky structure with large thickness and had a smooth surface. A significantly rougher surface with a porous structure was observed after the adsorption of lead (Saleh et al., 2017). The presence of lead on the surface of PP was observed after adsorption, as indicated by the red dots (Fig. 3(b)). The results of EDX analysis (Table 4), showing the presence of lead after adsorption, confirmed that lead can be adsorbed on the surface of PP.
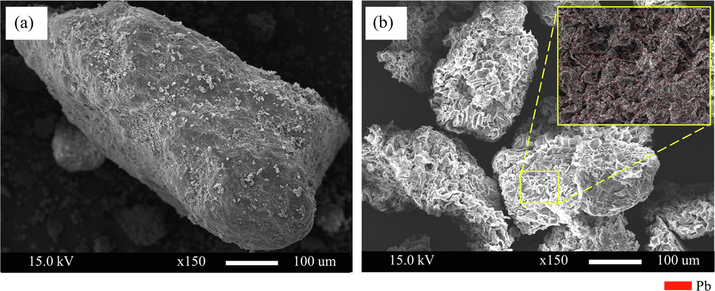
- SEM coupled with EDX of PP surface morphology: (a) before lead adsorption and (b) after lead adsorption.
Elements | Mass (%wt) | |
---|---|---|
Before adsorption | After adsorption | |
C | 51.50 | 43.67 |
O | 43.69 | 49.49 |
K | 3.54 | 1.04 |
Pb | – | 1.56 |
Cl | 1.27 | – |
Na | – | 3.44 |
Ca | – | 0.80 |
The elemental composition obtained from EDX analysis showed that generally elements were notices including C, O, K, Cl, Na and Ca which are frequently found in plant cells (Zvinowanda et al., 2010; Oladipo et al., 2020). The disappearance of Cl after adsorption might due to the detection limit refer to biological samples is quite low (Scimeca et al., 2018). Thus, the presence of heavy elements in the biological samples results in higher detection limits owing to the higher background ‘noise’ (Scimeca et al., 2018). Moreover, the concentration of Na and Ca increased slightly might be attributed to the uncertainty of EDX characterization, small amount of impurities within the distilled water, small amount of impurities to adjust the acidity-basicity of solutions and any impurities on the surface of laboratory glassware (Darvanjooghi et al., 2018).
3.1.2 BET
In Fig. 4, the N2 adsorption–desorption isotherms of PP are depicted. According to the IUPAC system, the results were found to be type IV isotherm that exhibited an H3 hysteresis loop at 0.5 < p/p0 < 1.0. The N2 adsorption on PP exhibited a slit like pore structure (Fu and Huang, 2018). Further, the Barrett-Joyner-Halenda (BJH) average pore diameters of PP proved to be 9.046 nm. The values of the average pore diameters determined that PP had a mesoporous structure with respect to IUPAC classification (Fatombi et al, 2019). In addition, the average pore volume of PP was 0.0012 cm3/g. The BET analysis yielded an average surface area of 0.755 m2/g for PP, which is significantly lower than the absorbents prepared from ripe papaya peel (9.63 m2/g) or activated carbon based papaya peel (15.28 m2/g) (Abbaszadeh et al., 2015; Abbaszadeh et al., 2016). It is significant that the lower surface area was linked to the surface morphology of PP (bulky smooth structure) observed before adsorption. This is in contrast to previous works, which reported that the surface morphology of ripe papaya peel and papaya peel activated carbon adsorbents were highly porous and had uneven surfaces (Abbaszadeh et al., 2015; Abbaszadeh et al., 2016). Thus, the differences in adsorbent preparation resulted in different characteristic of the prepared adsorbent. For instance, higher surface areas of 9.63 and 15.28 m2/g were obtained from previous works due to the raw material (ripened papaya peel) has been pretreated before the drying step i.e. boiled and then heated at high temperature followed by the surface modification with chemical solution (Abbaszadeh et al., 2015; Abbaszadeh et al., 2016).
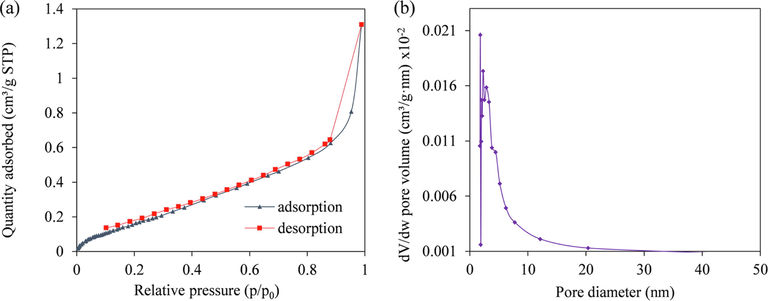
- BET analysis of N2 adsorption–desorption isotherm (a) and pore-size distribution curves of PP (b).
Hence, the adsorption behavior is affected by various factors such as functional groups contained in the material, characteristic of adsorbent and operating conditions in the process. Darvanjooghi et al., (2018) stated that the pH of aqueous solution is one of the most important variables affecting the adsorption process. Moreover, the unripe papaya peel contains an enzyme namely; papain which exhibits the potential to bind with metals ions in the solution (He et al., 2010; Cahyaningrum et al., 2013). Previous studied also reported high performance of adsorption percentages using quite low surface area of prepared adsorbents (Amarasinghe and Williams, 2007; Feng and Guo, 2012).
3.1.3 FTIR
The FTIR spectra of PP and PP-Pb are illustrated in Fig. 5. It is acknowledged that the hydroxyl (O-H) functional groups contained in the agricultural wastes were able to adsorb heavy metals from the aqueous medium (Basu et al., 2017; Dawn and Vishwakarma, 2021). It is noted that the peak of the hydroxyl groups (O-H) of primary alcohol shifted from 1,030 to 1,025 cm−1 (Iqbal et al., 2009; Oikonomopoulos et al., 2010). As for the C-O of polysaccharide, the band shifted from 1,197 to 1,145 cm−1 (Gómez-Ordóñez and Rupérez, 2011; Fanta and Salch, 1991). The shifts of these peaks signify that both O-H and C-O were the main functional groups contributing to the binding of lead on the surface of the prepared adsorbent during the process of biosorption (Abbaszadeh et al., 2015; Abbaszadeh et al., 2016; Iqbal et al., 2009). The changes in intensity of the peaks demonstrated the interaction between the adsorbent surface of the functional groups and the metal ions in the aqueous solution (Abbaszadeh et al., 2015; Iqbal et al., 2009). The broad band around 3,239 cm−1 is assigned to O-H stretching, indicating a high content of alcohol and phenolic-OH functional groups in PP (Chen et al, 2015). The bands at 2,850 and 2,918 cm−1 correspond to the C-H stretching, indicating the presence of polysaccharide compounds (Fatombi et al, 2019). The band observed around 1,729 cm−1 is attributed to the C = O stretching (Yargıç et al, 2015). Moreover, the band at 1,238 cm−1 is characteristic of the C = O stretching vibration in tertiary amides, suggesting that PP contains protein (Fatombi et al, 2019). The band around 1,197 cm−1 is associated with the C-O stretching vibration from polysaccharide (Gómez-Ordóñez and Rupérez, 2011; Fanta and Salch, 1991). The bands at 1,318 and 1,030 cm−1 are assigned to C-N stretching and the C-O stretching of alcohol and lignin (Li et al., 2019; Fatombi et al, 2019; Iqbal et al., 2009). The wave number which ranged from 950 to 1,200 cm−1 is considered to be the finger printed region of the carbohydrates (Chen et al, 2015).
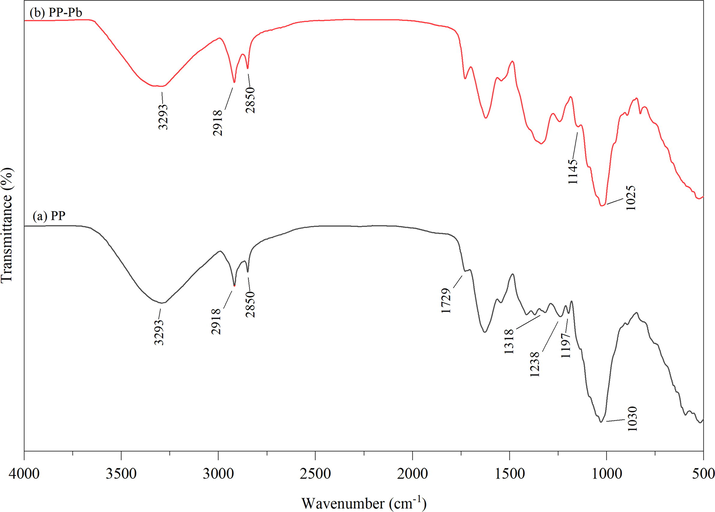
- Illustrate the results of FTIR before and after lead adsorption.
3.2 Statistical analysis of biosorption of lead
3.2.1 RSM based on BBD approach
A regression equation as a function of the response (%E) and independent variables can be expressed as follows in Eq.(16):
In Table 5, the experimental and predicted values of removal percentages are reported. In Fig. 6, the predicted values of lead adsorption are plotted against the actual experimental data from run numbers 1 to 27, yielding the R2 value of 0.9805, which verified the accuracy of the models and can be applied in the experiment. The high lead removal efficiencies from 83.56% to 99.87% were also obtained from the experimental run numbers: 1 to 27, which were subject to different operating conditions. Results obtained were slightly higher than the values from the ripe papaya peel adsorbents but were comparable to the results using papaya peel activated carbon or papaya peel adsorbent loaded with ferrous nanoparticles (Abbaszadeh et al., 2015; Abbaszadeh et al., 2016; Abbaszadeh et al., 2017).
Run | % Removal | Run | % Removal | ||
---|---|---|---|---|---|
Experimental | Predicted | Experimental | Predicted | ||
1 | 94.72 | 95.02 | 15 | 96.09 | 96.69 |
2 | 96.75 | 96.10 | 16 | 96.23 | 96.08 |
3 | 96.36 | 97.22 | 17 | 96.51 | 95.59 |
4 | 94.40 | 94.31 | 18 | 83.56 | 83.94 |
5 | 87.83 | 88.76 | 19 | 91.36 | 90.90 |
6 | 96.05 | 96.01 | 20 | 99.87 | 100.00 |
7 | 91.81 | 92.06 | 21 | 95.59 | 95.43 |
8 | 97.60 | 96.89 | 22 | 95.06 | 95.09 |
9 | 96.02 | 95.85 | 23 | 97.10 | 96.98 |
10 | 94.01 | 93.51 | 24 | 97.66 | 97.73 |
11 | 96.04 | 96.51 | 25 | 96.48 | 96.69 |
12 | 96.90 | 97.03 | 26 | 96.99 | 96.69 |
13 | 89.72 | 89.84 | 27 | 96.54 | 96.69 |
14 | 91.49 | 90.86 |
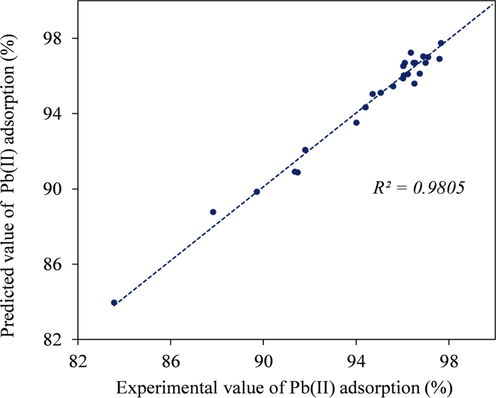
- Fitting qualities between experimental and predicted values.
In Table 6, ANOVA analyses are presented. The significance of the coefficient terms depends on the P-value and the F-value. A P-value < 0.05 is considered to be statistically significant (Singh and Bhateria, 2020; Alkarkhi, 2021). Further, previous studies have reported that when the P-value = 0.00, it is also significant (Ekpenyong et al, 2017). A high value of F indicates that most of the variables in the response can be explained by a regression equation. In Table 6, a high magnitude of F-value (42.99) is observed. Such a magnitude is seen to verify the fact that the independent variables in the model equation contributed to the removal of lead (Singh and Bhateria, 2020; Uçurum et al., 2018). Further, a lack-of-fit test is used to analyze whether the model fit is appropriate (Aliyu, 2019). The lack-of-fit of the model was found to be insignificant (P-value = 0.12, > 0.05), implying that 12% lack-of-fit was possibly due to the noise (Rai et al., 2019; Sharma et al., 2009). Herein, the following significant terms: X1 (initial lead concentration), X3 (adsorbent dosage), X4 (contact time), X1X1 (self-interaction of initial lead concentration), X3X3 (self-interaction of adsorbent dosage), X1X2 (interaction between initial lead concentration and pH of feed solution) and X1X3 (interaction between initial lead concentration and adsorbent dosage) cannot be ignored.
Source | Degree of freedom | Sum of squares | Mean square | F-value | P-value |
---|---|---|---|---|---|
Model | 14 | 307.13 | 21.94 | 42.99 | 0.00 |
Linear | 4 | 125.35 | 31.34 | 61.42 | 0.00 |
X1 | 1 | 2.53 | 2.53 | 4.96 | 0.05 |
X2 | 1 | 0.13 | 0.13 | 0.25 | 0.63 |
X3 | 1 | 109.65 | 109.65 | 214.90 | 0.00 |
X4 | 1 | 13.04 | 13.04 | 25.56 | 0.00 |
Square | 4 | 58.20 | 14.55 | 28.51 | 0.00 |
X1X1 | 1 | 3.46 | 3.46 | 6.77 | 0.02 |
X2X2 | 1 | 0.26 | 0.26 | 0.50 | 0.49 |
X3X3 | 1 | 51.26 | 51.26 | 100.45 | 0.00 |
X4X4 | 1 | 0.13 | 0.13 | 0.26 | 0.62 |
2-Way interaction | 6 | 123.57 | 20.60 | 40.36 | 0.00 |
X1X2 | 1 | 3.97 | 3.97 | 7.77 | 0.02 |
X1X3 | 1 | 115.12 | 115.12 | 225.61 | 0.00 |
X1X4 | 1 | 2.06 | 2.06 | 4.03 | 0.07 |
X2X3 | 1 | 0.66 | 0.66 | 1.29 | 0.28 |
X2X4 | 1 | 0.30 | 0.30 | 0.58 | 0.46 |
X3X4 | 1 | 1.48 | 1.48 | 2.89 | 0.12 |
Error | 12 | 6.12 | 0.51 | N/A | N/A |
Lack-of-fit | 10 | 5.97 | 0.60 | 7.70 | 0.12 |
Pure error | 2 | 0.16 | 0.08 | N/A | N/A |
Total | 26 | 313.25 | N/A | N/A | N/A |
3.2.2 Optimization of adsorption process
In Fig. 7, the optimization plots for lead adsorption using PP as an adsorbent are presented. By varying the concentration of lead in the range of 10 to 100 mg/L, the removal of lead decreased from 97.50 to 85.00%. Such a decrease in lead removal is attributed to the specific sites, which are saturated and to the exchange sites that are filled (Raju et al., 2012).
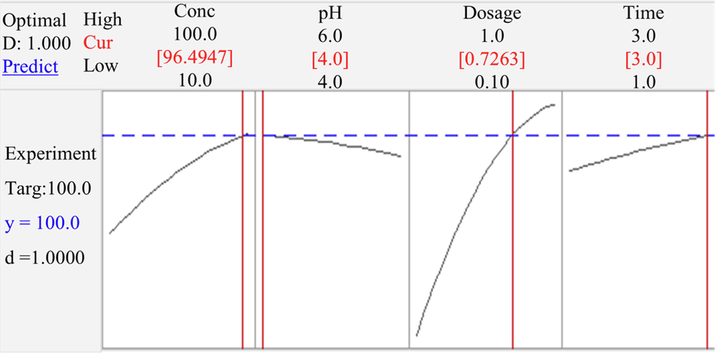
- Optimization plots for lead adsorption.
Consequently, the influence of pH of feed solution was also determined. Owing to the competition between lead ions and H+ ions for appropriate sites on the adsorbent surfaces, maximum percentage for lead removal was seen to yield at pH 4, which was in agreement with previous studies (Raju et al., 2012; Darvanjooghi et al., 2018). Preliminary studies were performed in the pH range of 2–10; results are given in the supplementary material, Fig. S1. As shown, the maximum percentages of lead removal were observed at pH 4–6. In an acidic medium, the lower amount of lead adsorption on the adsorbents was due to the competition between lead and H+ (Darvanjooghi et al., 2018). Thus, at the optimum pH of 4, lead ions were able to replace the H+ bond, forming part of the surface functional groups such as –OH and –COOH (Raju et al., 2012). A downward trend in the removal percentages is noticed with further increased in pH value due to the formation of lead hydroxide resulting in the precipitation of lead in the solution (Abbaszadeh et al., 2015; Raju et al., 2012; Darvanjooghi et al., 2018; Kampalanonwat and Supaphol, 2014). Darvanjooghi et al., (2018) also reported that it is recommended to carry out experiments at pH 4 in order to avoid the precipitation of lead into the solution.
Besides, when the dosage of adsorbent was increased from 0.1 to 1.0 g/50 mL, the removal of lead was found to increase from 85.00 to 97.50%. Hence, as the amount of dosage increased, the metal uptake of the adsorbent increased. It is evident that the removal of lead increased as the number of active sites increased, generated via the increase in dosage of the adsorbent (Raju et al., 2012).
Finally, the optimum time for lead adsorption was found to be 3 h. Removal percentages increased from 90% at 1 h of operation to 97.50% at 3 h of operation. At the initial stages, it is observed that removal percentages increased because more unsaturated surfaces and active sites were available on the surface areas of the adsorbents. As the process of adsorption proceeded, it is seen that more lead ions were adsorbed onto the surfaces (Abbaszadeh et al., 2016).
Applying Minitab software, the optimal conditions for the independent variables were identified: namely, initial lead concentration in wastewater at 96.5 mg/L, pH of wastewater at 4, adsorbent dosage at 0.73 g/50 mL (14.6 g/L) and contact time at 3 h. Thus, predicted and experimental values of lead removal efficiency were found to be 100% and 97.54%, respectively. Adsorption capacity under the optimized conditions proved to be 6.45 mg/g. It is noted that lead adsorption capacity remained significantly lower, as previously reported, when ripe papaya peel adsorbents were used (Abbaszadeh et al., 2015). This means that higher amounts of adsorbent dosage were required for PP. Nonetheless, the clear advantage associated with using unripe papaya peel adsorbents was the ease of adsorbent preparation without any surface modification and much lower costs involved.
3.2.3 Interpretation of three-dimensional surface plots
In Fig. 8, three-dimensional surface plots of lead removal efficiency as a function of independent variables are displayed. Interaction between the adsorbent dosage (X3) and the remaining variables yielded apparent changes in lead removal efficiency. This is in contrast to the interaction between initial lead concentration (X1) and pH of wastewater (X2) and the interaction between initial lead concentration (X1) and contact time (X4), which resulted in relatively unchanged response curves. The results of the three-dimensional plots concurred with the ANOVA analysis, indicating the importance of adsorbent dosage on the percentage of lead removal.
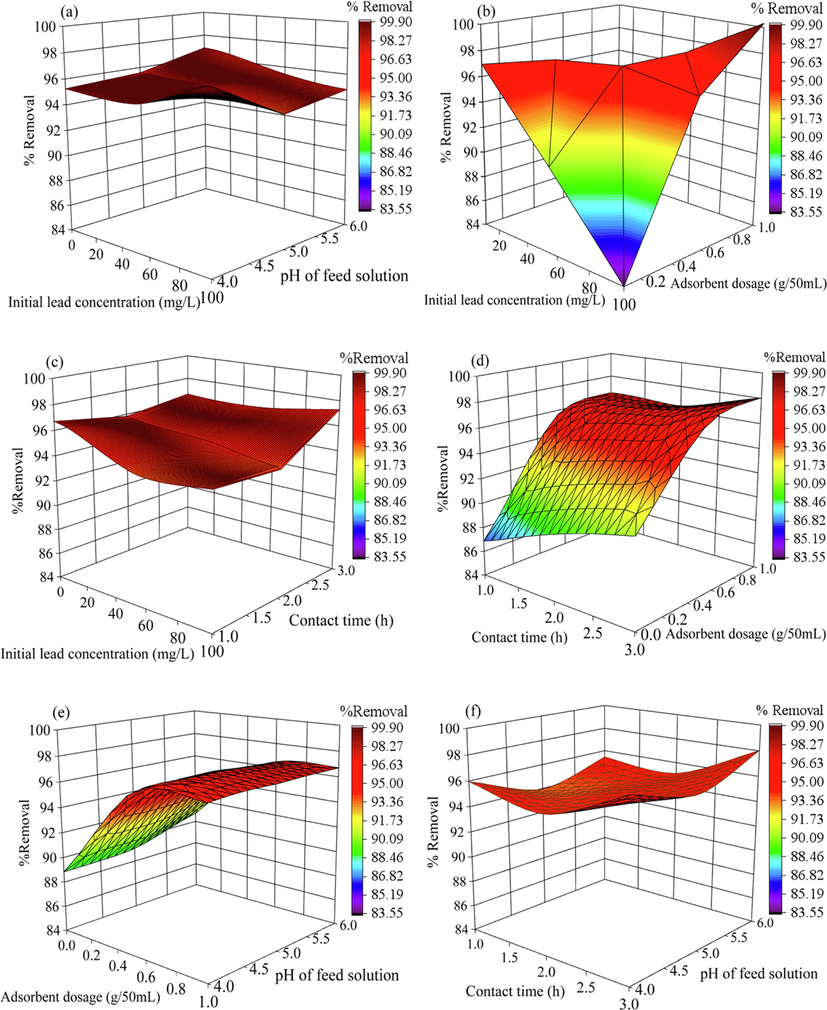
- Three-dimensional plots of (a) initial lead concentration and pH of feed solution (b) initial lead concentration and adsorbent dosage (c) initial lead concentration and contact time (d) contact time and adsorbent dosage (e) adsorbent dosage and pH of feed solution and (f) contact time and pH of feed solution.
3.3 Biosorption mechanism
The hydroxyl groups in cellulose are capable of being chemically modified by many reagents. In Fig. 9, the adsorption of lead on the surface of cellulose in the unripe papaya peel based bio-adsorbents occurred via –OH group (Adel, 2016). Thus, the formation of covalent bonds led to the adsorption of lead on the cellulose surface. Additionally, it has been reported that the unripe fruit of Carica papaya contains an important ingredient viz. papain, a thiol enzyme (He et al., 2010). Hence, papain is exhibited a potential for the selective binding of metal in an aqueous medium as well as the use in metal-chelated affinity separation. Previous research has shown that papain can react positively with various types of heavy metals (He et al., 2010; Cahyaningrum et al., 2013). Therefore, the unripe papaya peel is a good candidate than ripe papaya peel on account of its natural abundance waste, its ease of preparation and much lower costs involved.
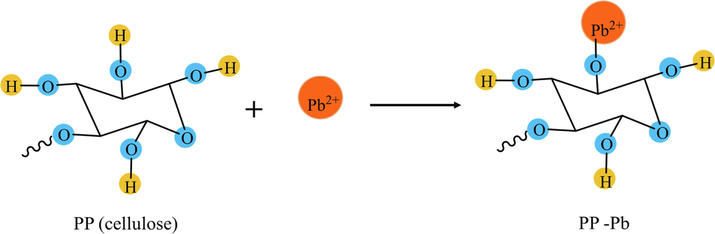
- Possible reaction mechanisms of PP with lead.
3.4 Adsorption isotherm
The results of lead adsorption applying PP were correlated using the Langmuir, Freundlich, Temkin and D-R isotherms. The fit of the model with the experimental results was evaluated using linear regression analysis (as seen in the Supplementary material, Fig. S2). Thus, it is noted that the R2 obtained from the Freundlich isotherm was the highest. Such a high value indicated that the Freundlich isotherm was the best model for lead adsorption, suggesting multilayer adsorption on the heterogeneous surface (Dada et al., 2012). Similar results were reported when adsorbents from ripe papaya peel were used to remove lead from an aqueous solution (Abbaszadeh et al., 2015). The value of n in this study was found to be 0.784, signifying that chemisorption took place (Nandiyanto et al., 2020; Desta, 2013). Yet, the values of the Langmuir parameters (qm and KL) were found to be negative; such negative results are perhaps due to the electrostatic nature of the process of adsorption (Huang et al., 2013; Ku and Caliskan, 2009; Özcan et al., 2004).
3.5 Adsorption kinetic
In the supplementary material, Fig. S3, the linear regression analysis and kinetic parameters of pseudo-first-order, pseudo-second-order and intraparticle diffusion models are given. Results revealed that the pseudo-second-order model yielded a higher correlation coefficient (R2 = 0.998) than the pseudo-first-order and the intraparticle diffusion models. This observation indicated that the pseudo-second-order was the most suitable model for lead adsorption using unripe papaya peel. Results also suggest that chemical adsorption was the rate determining step of the process (Ali et al., 2016). This aligns with the work of previous researchers, who have reported pseudo-second-order to be the best fit model for metal adsorption when using adsorbents prepared from biomass containing tannin (Abbaszadeh et al., 2016; Ramakul et al., 2012; Şengil and Özacar, 2009). Moreover, the magnitude of the adsorption capacity of the pseudo-second-order model (qe,cal = 6.25 mg/g) was found to be comparable to the value obtained experimentally (qe = 6.45 mg/g) under the optimized conditions via RSM. Such a result confirmed the validity of the pseudo-second-order, proving that the kinetic model applied for lead adsorption using PP was the most suitable. As for the intraparticle diffusion model, its low correlation coefficient (R2 = 0.7701) suggests that the adsorption process was not controlled by intraparticle diffusion as normally reported for other porous materials (Carvalho et al., 2018; Cheng et al., 2013). Due to the relatively smooth surface morphology of the prepared bio-adsorbents, it is possible to conclude that the intraparticle diffusion of lead into the adsorbent was unlikely.
3.6 Thermodynamic study
In the supplementary material, Fig. S4, and Table 7, plots are displayed of ln kc versus 1/T that consequently yielded the values of ΔH° and ΔS° at 13.61 J/mol and 54.30 J/mol⋅K, respectively. A positive value of ΔH° was observed indicating that the process of adsorption was endothermic. Previous research also reported the same outcome of an endothermic lead adsorption process when employing papaya seeds as bio-adsorbents (Yadav et al., 2014; Olu-Owolabi et al., 2018). Such a result can be explained given that the total energy released in bond breaking was lower than the total energy adsorbed in bond making between the lead and adsorbent (Mousavi et al., 2010; Abubakar et al., 2020). The positive value of ΔS° indicated an increase in randomness at the adsorbent-solution interface (Kumar et al., 2019). Negative values of ΔG°, however, demonstrated that the adsorption process was both thermodynamic and spontaneous, which is quite feasible; the increase of ΔG° with temperature implies that the process favors higher energy input (Abbaszadeh et al., 2016).
Δ⁰G (kJ/mol) | Δ⁰H (J/mol) | Δ⁰S (J/mol K) | ||
---|---|---|---|---|
303 K | 323 K | 343 K | ||
−2.941 | −3.703 | −5.142 | 13.612 | 54.304 |
3.7 Desorption study
Desorption experiments were evaluated by varying the concentration of HNO3. It is noted that the amount of excessive protons in HNO3 solution was seen to react with the adsorbent and can disrupt the bonds of adsorbate and adsorbent (Chatterjee and Abraham, 2019). Results found that the highest desorption efficiency was obtained at 3 M, as shown in Fig. S5. The desorption efficiency of PP was found to be 77.16%.
4 Conclusion
In this paper, results demonstrate that bio-adsorbents prepared from unripe papaya peel waste can effectively remove lead from aqueous solution. Herein, percentages of predicted and actual lead removal efficiencies reached 100% and 97.54%, respectively. Besides, isotherm and kinetic analyses revealed that Freundlich and pseudo-second-order models were best suited for the process of lead adsorption using unripe papaya peel based bio-adsorbents. Thermodynamic parameters proved that the process was both endothermic and spontaneous. Although the adsorption capacity was slightly lower than the values from the modified adsorbents prepared from ripen papaya peel, the ease of preparation and the likely lower expense associated with the process are clear advantages. Finally, this work underlines the case of utilizing agricultural waste and environmental friendly processes to remove pollutants from aqueous solutions.
CRediT authorship contribution statement
Wilavan Jaihan: Methodology, Data curation, Investigation, Writing - original draft. Vanee Mohdee: Validation, Writing - review & editing. Sompop Sanongraj: Project administration. Ura Pancharoen: Supervision, Conceptualization, Funding acquisition. Kasidit Nootong: Project administration, Validation, Writing - review & editing.
Acknowledgement
The authors profoundly appreciate the financial support by the 90th Anniversary of Chulalongkorn University Fund (Ratchadaphiseksomphot Endowment Fund), the Department of Chemical Engineering Fund (Faculty of Engineering, Chulalongkorn University for Master's Degree students) and the Research Cess Fund (Malaysia-Thailand Joint Authority), the Thailand Science Research and Innovation Fund Chulalongkorn University (CU_FRB65_bcg(25)_139_21_05). Sincere thanks also are extended to the Separation Laboratory (Department of Chemical Engineering, Faculty of Engineering, Chulalongkorn University) for their help and support. And authors gratefully thanks also go to Dr. Thidarat Wongsawa for her kind help.
Declaration of Competing Interest
The authors declare that they have no known competing financial interests or personal relationships that could have appeared to influence the work reported in this paper.
References
- Use of pristine papaya peel to remove Pb(II) from aqueous solution. Chem. Eng. Trans.. 2015;45:961-966.
- [CrossRef] [Google Scholar]
- Bio-adsorbent derived from papaya peel waste and magnetic nanoparticles fabricated for lead determination, Pure Appl. Chem.. 2017;90:79-92.
- [CrossRef] [Google Scholar]
- Treatment of lead-contaminated water using activated carbon adsorbent from locally available papaya peel biowaste. J. Clean. Prod.. 2016;118:210-222.
- [CrossRef] [Google Scholar]
- Thermodynamics Modelling of Lead (II) Biosorption using Cystoseira stricta Biomass. BSTR.. 2020;8:21-23.
- [Google Scholar]
- Incorporation of nano-metal particles with paper matrices. Interdiscip. J. Chem.. 2016;1:36-46.
- [CrossRef] [Google Scholar]
- Biosorptive removal of Pb2+ and Cd2+ onto novel biosorbent: Defatted Carica papaya seeds. Biomass Bioenergy.. 2011;35:2517-2525.
- [CrossRef] [Google Scholar]
- Potential of using green adsorbent of heavy metal removal from aqueous solutions: Adsorption kinetics, isotherm, thermodynamic, mechanism and economic analysis. Ecol. Eng.. 2016;91:317-332.
- [CrossRef] [Google Scholar]
- Synthesis, electron microscopy properties and adsorption studies of Zinc(II) ions (Zn2+) onto as-prepared carbon nanotubes (CNTs) using Box-Behnken design (BBD) Sci. Afr.. 2019;3:e00069
- [CrossRef] [Google Scholar]
- 6 - The observed significance level (P-value) procedure. In: Alkarkhi A.F.M., ed. Applications of hypothesis testing for environmental science. Elsevier; 2021. p. :79-119.
- [Google Scholar]
- Tea waste as a low cost adsorbent for the removal of Cu and Pb from wastewater. Chem. Eng. J.. 2007;132:299-309.
- [CrossRef] [Google Scholar]
- Removal of copper and lead using banana biochar in batch adsorption systems: isotherms and kinetic studies. Arab. J. Sci. Eng.. 2018;43:5711-5722.
- [CrossRef] [Google Scholar]
- Ariffin, N., Abdullah, M.M.A.B., Rozainy, Z., Murshed, M.F., Zain, H., Meor Ahmad Tajudin, M.A.F., Bayuaji, R., 2017. Review on adsorption of heavy metal in wastewater by using geopolymer, MATEC Web Conf. 97, 01023. https://doi.org/10.1051/matecconf/20179701023.
- Comparison of activation media and pyrolysis temperature for activated carbons development by pyrolysis of potato peels for effective adsorption of endocrine disruptor bisphenol-A. J. Colloid. Interface. Sci.. 2016;466:101-112.
- [CrossRef] [Google Scholar]
- Preparation and properties of papain immobilized onto metal ions cross-linked chitosan beads. Res. J. Pharm. Biol. Chem. Sci.. 2013;4:120-126.
- [Google Scholar]
- Caesalpinia ferrea fruits as a biosorbent for the removal of Methylene Blue dye from an aqueous medium. Water Air Soil Pollut.. 2018;229:297.
- [CrossRef] [Google Scholar]
- Desorption of heavy metals from metal loaded sorbents and e-wastes: A review. Biotechnol. Lett.. 2019;41:319-333.
- [CrossRef] [Google Scholar]
- Esterification of sugar beet pectin using octenyl succinic anhydride and its effect as an emulsion stabilizer. Food Hydrocoll.. 2015;49:53-60.
- [CrossRef] [Google Scholar]
- Toward 3D graphene oxide gels based adsorbents for high-efficient water treatment via the promotion of biopolymers. J. Hazard. Mater.. 2013;263:467-478.
- [CrossRef] [Google Scholar]
- Catalytic activation and application of micro-spherical carbon derived from hydrothermal carbonization of lignocellulosic biomass: Statistical analysis using Box-Behnken design. RSC Adv.. 2016;6:102680-102694.
- [CrossRef] [Google Scholar]
- Langmuir, Freundlich, Temkin and Dubinin-Radushkevich Isotherms studies of equilibrium sorption of Zn2+ unto phosphoric acid modified rice husk. J. Appl. Chem.. 2012;3:38-45.
- [CrossRef] [Google Scholar]
- Application of treated eggplant peel as a low-cost adsorbent for water treatment toward elimination of Pb2+: Kinetic modeling and isotherm study. Adsorp. Sci. Technol.. 2018;36:1112-1143.
- [CrossRef] [Google Scholar]
- Integral technique for evaluation and optimization of Ni (II) ions adsorption onto regenerated cellulose using response surface methodology, Arabian. J. Chem.. 2018;11:370-379.
- [CrossRef] [Google Scholar]
- Recovery and recycle of wastewater contaminated with heavy metals using adsorbents incorporated from waste resources and nanomaterials-A review. Chemosphere.. 2021;273:129677
- [CrossRef] [Google Scholar]
- Batch sorption experiments: Langmuir and Freundlich Isotherm studies for the adsorption of textile metal ions onto Teff Straw (Eragrostis tef) agricultural waste. J. Thermodyn.. 2013;2013:375830
- [CrossRef] [Google Scholar]
- Response surface modeling and optimization of major medium variables for glycolipopeptide production. Biocatal. Agric. Biotechnol.. 2017;10:113-121.
- [CrossRef] [Google Scholar]
- Chapter-12 Modelling of adsorption processes. In: Brennan C.R., ed. Mathematical modelling. UAE: Nova Publishers Inc.; 2013. p. :579-600.
- [Google Scholar]
- Analysis of polysaccharide-poly(ethylene-co-acrylic acid) composites by fourier-transform infrared spectroscopy. Carbohydr. Polym.. 1991;14:393-409.
- [CrossRef] [Google Scholar]
- Characterization and application of alkali-soluble polysaccharide of Carica papaya seeds for removal of indigo carmine and Congo red dyes from single and binary solutions. J. Environ. Chem. Eng.. 2019;7:103343
- [CrossRef] [Google Scholar]
- Characterization of adsorptive capacity and mechanisms on adsorption of copper, lead and zinc by modified orange peel. Trans. Nonferrous Met. Soc. China.. 2012;22:1224-1231.
- [CrossRef] [Google Scholar]
- Study of adsorption property and mechanism of lead(II) and cadmium(II) onto sulfhydryl modified attapulgite. Arabian J. Chem.. 2021;14:102960
- [CrossRef] [Google Scholar]
- Magnetic dithiocarbamate functionalized reduced graphene oxide for the removal of Cu(II), Cd(II), Pb(II), and Hg(II) ions from aqueous solution: Synthesis, adsorption, and regeneration. Chemosphere.. 2018;209:449-456.
- [CrossRef] [Google Scholar]
- Factorial experimental design for the optimization of highly selective adsorption removal of lead and copper ions using metal organic framework MOF-2(Cd) J. Mol. Liq.. 2018;272:15-26.
- [CrossRef] [Google Scholar]
- Kinetic and equilibrium study of Ni(II) sorption from aqueous solutions onto Peganum harmala-L. Int. J. Environ. Sci. Technol.. 2014;11:1835-1844.
- [CrossRef] [Google Scholar]
- FTIR-ATR spectroscopy as a tool for polysaccharide identification in edible brown and red seaweeds. Food Hydrocoll.. 2011;25:1514-1520.
- [CrossRef] [Google Scholar]
- Papain adsorption on chitosan-coated nylon-based immobilized metal ion (Cu2+, Ni2+, Zn2+, Co2+) affinity membranes. Sep. Sci. Technol.. 2010;45:525-534.
- [CrossRef] [Google Scholar]
- Adsorption kinetics and thermodynamics of water-insoluble crosslinked β-cyclodextrin polymer for phenol in aqueous solution. Macromol. Res.. 2013;21:726-731.
- [CrossRef] [Google Scholar]
- Iqbal, M., Saeed, A., Zafar, S.I., 2009. FTIR spectrophotometry, kinetics and adsorption isotherms modeling, ion exchange, and EDX analysis for understanding the mechanism of Cd2+ and Pb2+ removal by mango peel waste, J. Hazard. Mater. 164, 161-171. https://doi.org/10.1016/j.jhazmat.2008.07.141.
- Kampalanonwat, P., Supaphol, P., 2014. The study of competitive adsorption of heavy metal ions from aqueous solution by aminated polyacrylonitrile nanofiber mats, Energy Procedia. 56, 142–151. https://doi: 10.1016/j.egypro.2014.07.142.
- Heavy metals contamination in soil, surface water, crops, and resident blood in Uthai District, Phra Nakhon Si Ayutthaya, Thailand. Environ. Geochem. Health.. 2020;42:545-561.
- [CrossRef] [Google Scholar]
- Efficiency and kinetic rate of nanoscale and microscale of zero valent iron and zinc oxide particles for chromium, cadmium, and lead removal from Pak Thong Chai silk dyeing effluent, Thailand. TURCOMAT.. 2021;12:2690-2702.
- [CrossRef] [Google Scholar]
- Equilibrium and kinetic studies of the adsorption of Zn(II) ions onto natural and activated kaolinites. Adsorpt. Sci. Technol.. 2009;27:85-105.
- [CrossRef] [Google Scholar]
- Adsorption modeling and mechanistic insight of hazardous chromium on para toluene sulfonic acid immobilized-polyaniline@CNTs nanocomposites. J. Saudi Chem. Soc.. 2019;23:188-197.
- [CrossRef] [Google Scholar]
- Solid phase extraction of carbamate pesticides with banana peel derived hierarchical porous carbon prior to high performance liquid chromatography. Anal. Methods.. 2017;9:593-599.
- [CrossRef] [Google Scholar]
- Chitosan modification persimmon tannin bioadsorbent for highly efficient removal of Pb(II) from aqueous environment: The adsorption equilibrium, kinetics and thermodynamics. Environ. Technol.. 2019;40:112-124.
- [CrossRef] [Google Scholar]
- The study of lead removal from aqueous solution using an electrochemical method with a stainless steel net electrode coated with single wall carbon nanotubes. Chem. Eng. J.. 2013;218:81-88.
- [CrossRef] [Google Scholar]
- Heavy metal contamination near industrial estate areas in Phra Nakhon Si Ayutthaya Province, Thailand and human health risk assessment. Int. J. Environ. Res. Public Health.. 2018;15:1890.
- [CrossRef] [Google Scholar]
- Removal of lead from aqueous solution using waste tire rubber ash as an adsorbent. Braz. J. Chem. Eng.. 2010;27:79-87.
- [CrossRef] [Google Scholar]
- Cation exchange removal of Pb from aqueous solution by sorption onto NiO. J. Hazard. Mater.. 2009;168:364-368.
- [CrossRef] [Google Scholar]
- Isotherm adsorption characteristics of carbon microparticles prepared from pineapple peel waste. Commun. sci. technol.. 2020;5:31-39.
- [CrossRef] [Google Scholar]
- FTIR study of two different lignite lithotypes from Neocene Achlada lignite deposits in NW Greece. BGSG.. 2010;5:2284-2293.
- [CrossRef] [Google Scholar]
- Pawpaw (Carica papaya) peel waste as a novel green heterogeneous catalyst for Moringa oil methyl esters synthesis: Process optimization and kinetic study. Energies.. 2020;13:5834.
- [CrossRef] [Google Scholar]
- Fractal-like concepts for evaluation of toxic metals adsorption efficiency of feldspar-biomass composites. J. Clean. Prod.. 2018;171:884-891.
- [CrossRef] [Google Scholar]
- Adsorption of Acid Blue 193 from aqueous solutions onto Na-bentonite and DTMA-bentonite. J. Colloid Interface Sci.. 2004;280:44-54.
- [CrossRef] [Google Scholar]
- Removal of lead, zinc and iron by coagulation-flocculation. J. Taiwan. Inst. Chem. Eng.. 2011;42:809-815.
- [CrossRef] [Google Scholar]
- Adsorption isotherms and thermochemical data of FD and C Red N° 40 binding by chitosan, Brazilian. J. Chem. Eng.. 2011;28:295-304.
- [CrossRef] [Google Scholar]
- Optimization of sugar release from banana peel powder waste (BPPW) using box-behnken design (BBD): BPPW to biohydrogen conversion. Int. J. Hydrog. Energy.. 2019;44:25505-25513.
- [CrossRef] [Google Scholar]
- Comparison studies on biosorption of lead (II) from an aqueous solution using anacardium occidentale and Carica papaya leaves powder. IJETED.. 2013;3:273-283.
- [Google Scholar]
- Sorption of lead(II) ions from wastewater using carica papaya leaf powder. Int. J. Eng. Sci. Adv. Technol.. 2012;2:1577-1581.
- [Google Scholar]
- Biosorption of palladium(II) and platinum(IV) from aqueous solution using tannin from Indian almond (Terminalia catappa L.) leaf biomass: Kinetic and equilibrium studies. Chem. Eng. J.. 2012;193–194:102-111.
- [CrossRef] [Google Scholar]
- Adsorption of lead onto a waste biomaterial-biochar. Nat. sci.. 2017;15:154-164.
- [CrossRef] [Google Scholar]
- Energy Dispersive X-ray (EDX) microanalysis: A powerful tool in biomedical research and diagnosis. Eur. J. Histochem.. 2018;62:2841.
- [CrossRef] [Google Scholar]
- Competitive biosorption of Pb2+, Cu2+ and Zn2+ ions from aqueous solutions onto valonia tannin resin. J. Hazard. Mater.. 2009;166:1488-1494.
- [CrossRef] [Google Scholar]
- Application of response surface methodology (RSM) for optimization of nutrient supplementation for Cr(VI) removal by Aspergillus lentulus AML05. J. Hazard. Mater.. 2009;164:1198-1204.
- [CrossRef] [Google Scholar]
- Detoxification of wastewater by Paw-Paw (Carica papaya L.) seeds adsorbents. Asian J. Chem.. 2019;31:2249-2256.
- [CrossRef] [Google Scholar]
- Optimization and experimental design of the Pb2+ adsorption process on a nano-Fe3O4-based adsorbent using the response surface methodology. ACS Omega.. 2020;5:28305-28318.
- [CrossRef] [Google Scholar]
- Demands and consumption behavior of papaya salad restaurants in the mid northeastern part, Khon Kaen Agr. J.. 2018;46:702-707.
- [Google Scholar]
- Simultaneous extraction and stripping of lead ions via a hollow fiber supported liquid membrane: Experiment and modeling. J. Ind. Eng. Chem.. 2014;20:2584-2593.
- [CrossRef] [Google Scholar]
- Optimization of adsorption parameters for Ultra-Fine calcite using a Box-Behnken experimental design. Open Chem.. 2018;16:992-1000.
- [CrossRef] [Google Scholar]
- Removal of 4-nitrophenol from aqueous solution by natural low-cost adsorbents, Indian. J. Chem. Technol.. 2012;19:7-25.
- [Google Scholar]
- A nanoporous carbon material derived from pomelo peels as a fiber coating for solid-phase microextraction. RSC Adv.. 2016;6:113951-113958.
- [CrossRef] [Google Scholar]
- Development of dispersive liquid-liquid microextraction based on solidification of floating organic drop for the sensitive determination of trace copper in water and beverage samples by flame atomic absorption spectrometry. Bull. Korean Chem. Soc.. 2011;32:829-835.
- [CrossRef] [Google Scholar]
- Sensitive determination of cadmium in water, beverage and cereal samples by a novel liquid-phase microextraction coupled with flame atomic absorption spectrometry. Anal. Methods.. 2011;3:210-216.
- [CrossRef] [Google Scholar]
- Chemical carbonization of papaya seed originated charcoals for sorption of Pb (II) from aqueous solution. J. Environ. Chem. Eng.. 2014;2:9-19.
- [CrossRef] [Google Scholar]
- Assessment of toxic copper(II) biosorption from aqueous solution by chemically-treated tomato waste. J. Clean. Prod.. 2015;88:152-159.
- [CrossRef] [Google Scholar]
- Treatment of lead contaminated water using synthesized nano-iron supported with bentonite/graphene oxide. Arab. J. Chem.. 2020;13:3474-3483.
- [CrossRef] [Google Scholar]
- Recovery of Lead (II) from Aqueous Solutions by Zea mays Tassel Biosorption. Am. J. Biochem. Biotechnol.. 2010;6(1):1-10.
- [CrossRef] [Google Scholar]
Appendix A
Supplementary material
Supplementary data to this article can be found online at https://doi.org/10.1016/j.arabjc.2022.103883.
Appendix A
Supplementary material
The following are the Supplementary data to this article: