Chemical composition, antioxidant, antimicrobial and antiproliferative activity of Laureliopsis philippiana essential oil of Chile, study in vitro and in silico
⁎Corresponding author at: Laboratorio de Productos Bioactivos, Facultad de Medicina, CIB, Universidad Diego Portales, Ejército 141, código postal 8370007, Santiago, Chile. jessica.bravo@mail.udp.cl (Jessica Bravo)
-
Received: ,
Accepted: ,
This article was originally published by Elsevier and was migrated to Scientific Scholar after the change of Publisher.
Peer review under responsibility of King Saud University.
Abstract
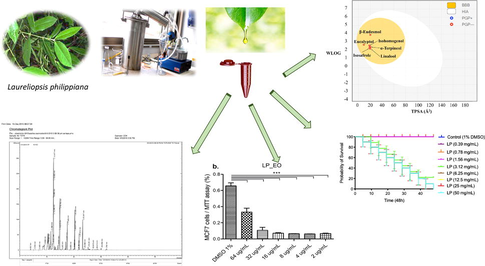
Abstract
Chilean Laureliopsis philippiana has been used in traditional medicine by the Mapuche and their ancestors. To evaluate its pharmacological activity, Laureliopsis philippiana leaf essential oil extract (LP_EO) was chemically and biologically characterized in the present study. In vitro antioxidant potential was analyzed, and antitumor activity was evaluated in non-tumor and tumor cell culture lines. Caenorhabditis elegans was used as a model for evaluating toxicity, and the chemical composition of the essential oil was analyzed using gas chromatography–mass spectrometry. The oil contains six major monoterpenes: eucalyptol (27.7 %), linalool (27.6 %), isozaphrol (19.5 %), isohomogenol (12.6 %), α-terpineol (7.7 %), and eudesmol (4.8 %). Based on quantum mechanical calculations, isosafrole and isohomogenol conferred in vitro antioxidant and antimicrobial activity to LP_EO. In addition, LP_EO showed antimicrobial activity against clinical Helicobacter pylori isolates (MIC 64 and MBC > 128 μg·mL−1), Staphylococcus aureus (MIC 32 and MBC > 64 μg·mL−1), Escherichia coli (MIC 8 and MBC 16 μg·mL−1) and Candida albicans (MIC 64 and > 128 μg·mL−1). LP_EO could selectively inhibit the proliferation of epithelial tumor cell lines but showed low toxicity against Caenorhabditis elegans (0.39 to 1.56 μg·mL−1). Therefore, LP_EO may be used as a source of bioactive compounds in novel pharmacological treatments for veterinary and human application, cosmetics, or sanitation.
Keywords
Essential oil
Laureliopsis philippiana
Antioxidant
Antiproliferative
Antimicrobial activity
Chemical composition
- EO
-
essential oil
- HP
-
Helicobacter pylori
- EC
-
Escherichia coli
- SA
-
Staphylococcus aureus
- CA
-
Candida albicans, and MC, majority compounds
- AC
-
antioxidant capacity
- GC–MS
-
Gas chromatography–mass spectrometry
- MIC
-
minimum inhibitory concentration, HD, hydrodistillation
- LP
-
Laureliopsis philippiana
- MW
-
molecular weight
- TPSA
-
Topological polar surface area
- HBA
-
H-bond acceptors
- HBD
-
H-bond donor
- RB
-
Distribution of number of rotatable bonds
- Log P
-
Decimal logarithm of the partition coefficient between n-octanol and water
- Log S
-
Decimal logarithm of the molar solubility in water
- log Kp
-
predicting the skin permeability coefficient
- FRAP
-
ferric-reducing antioxidant power
Abbreviations
1 Introduction
Laureliopsis philippiana (Looser) Schodde grows in humid places and deep soils. It is part of sclerophyll forests, abundant in Llanquihue and Chiloé. It reaches 20 m in height. Polygamous-monoecious or dioecious with a straight trunk up to 1 m in diameter and a smooth bark. Hanging lower branches. Evergreen and bright green leaves, decussate, opposite, coriaceous, oblong-lanceolate, serrate margin with a gland at the tip of each tooth and very aromatic. Flowers gathered in axillary inflorescence and hairy, 6 to 8 greenish yellow tepals. Fruit: free achenes enveloped in the receptacle. Hairy seeds with persistent style (Bittner et al., 2009; Sukmark et al., 2011).
L. philippiana wood is use as a raw material in construction, and its byproducts are greatly appreciate in sculpture and woodcarving. However, it was decided to focus on the therapeutic potential of this tree. Mapuche aborigines and their ancestors, practicing the traditional Mapuche medicine, have used Laureliopsis philippiana. Both Chilean and Argentinian aborigine populations use this tree to treat a group of diseases described in allopathic medicine as nervous system disorders(Molares and Ladio 2009). In addition, the bark, flowers, and leaves of this species have conventionally been used as expectorants and to treat inflammation and cold symptoms (Molgaard et al., 2011).
Phytochemical studies have revealed high contents of aporphinoid and bisbenzylsoquinoline alkaloids in the stem bark and leaves of Laureliopsis philippiana (Staerk et al., 2009; Stanstrup et al., 2010). In addition, repellent and insecticidal properties of Laureliopsis philippiana leaves powdered against Sitophilus zeamais Motschulsky (Coleoptera) have been reported (Ortiz et al., 2012). Furthermore, insecticidal and fungicidal activities of Essential oils (EOs) from Laureliopsis philippiana leaves have been demonstrated (Bittner et al., 2008; Bittner et al., 2009; Avello Lorca et al., 2012).
EOs are terpene blends found in various plant parts, such as leaves, bark, roots, flowers, and fruits. Since ancient times, EOs have been used in traditional medicine to treat various human diseases. Today, their application has been extended to the cosmetic industry, beverages and food production (Molares and Ladio 2009; Man et al., 2019), food processing, and the agricultural industry, in general (e.g., for food preservation and in insect repellents) (Sameh et al., 2019).
EOs are secondary metabolites, formed by a mixture of different compounds defined as terpenes (monoterpenes and sesquiterpenes), aromatic compounds (aldehydes, alcohols, phenols, and methoxy derivatives, among others), and terpenoids (isoprenoids) (Amin Mohamed 2010). In vitro antimicrobial, antitumor, and antioxidant activities of EOs extracted from various plant species are known (Soliman et al., 2017).
In 2017, WHO published the “Global Priority List of Antibiotic-Resistant Bacteria to Guide Research, Discovery, and Development of New Antibiotics.” Following this, the need for the development of novel strategies to combat bacterial infections was emphasized, and a list of multidrug-resistant bacteria was published. According to this list, specific attention should be given to Priority 2 bacteria, including clarithromycin-resistant Helicobacter pylori, vancomycin-resistant Staphylococcus aureus, penicillin-non-susceptible Streptococcus pneumoniae, and ampicillin-resistant Haemophilus influenzae. In addition, the report for the Secretary General of the United Nations issued in April 2019 by the Inter-Agency Coordination Group on Antimicrobial Resistance published by WHO (Robertson et al., 2021), mentioned that more efforts, investments, and incentives are warranted to promote innovation in antimicrobial drugs. Also in diagnostic tests, vaccines, waste management tools, safe and effective alternatives to antimicrobials, and alternative practices, in addition to operational research and studies on their applications in the field of human, non-human animal, and plant health (Robertson et al., 2021).
It has been reported antibacterial activity of the methanolic extracts of Laureliopsis philippiana leaves containing EOs against Escherichia coli, Pseudomonas aeruginosa, Staphylococcus aureus, Bacillus subtilis, Streptococcus pneumoniae, and Penicillium expansum (Molgaard et al., 2011). Additionally, Laureliopsis philippiana EOs obtained via hydrodistillation (HD) showed antibacterial activity against Enterobacter aerogenes, Escherichia coli, Staphylococcus aureus and Staphylococcus epidermidis (Toledo et al., 2014). Furthermore, antifungal activity of Laureliopsis philippiana against various pathogenic fungi affecting trees and small plants, including Fusarium oxysporum, Rhizoctonia solani, Pythium irregularulare, Ceratocystis pilifera, and Phragmidium violaceum, has been demonstrated (Bittner et al., 2009). Laureliopsis philippiana EO exhibited antimicrobial activity against the oomycete Saprolegnia spp.(Madrid et al., 2015).
Oxidative stress is well-known to trigger many pathological conditions, including cancer (Schieber and Chandel 2014), neurological disorders (Parkinson and Alzheimer disease) (Trist et al., 2019), hypertension, and diabetes, cancer among others (Yadav et al., 2016). To counteract the effects of oxidative stress, antioxidant substances of endogenous or exogenous origin are used. The efficacy of exogenous antioxidants, specifically those constituting EOs, as substances used to protect biological tissues from oxidative stress depends on the nature of the antioxidant and its mechanism of action (Niki 2010). Currently, diverse in vitro methods are available which are complementary and allow the approximation of the possible nature of antioxidant activity (Brewer 2011). However, no study has quantified flavonoids and polyphenols in or assessed the antioxidant and antitumoral activity of Laureliopsis philippiana (Bittner et al., 2009; Staerk et al., 2009; Zapata and Smagghe 2010; Madrid et al., 2015; Torres et al., 2015; Bustos et al., 2017).
A good approximation to estimate biological activity is in silico tests. Boundary orbital calculations provide energies of the highest occupied molecular orbital (HOMO) and the lowest unoccupied molecular orbitals (LUMO). Based on these calculations, the possibility of electronic transfer can be estimated as a function of the energy of HOMO (electron donor) and LUMO (electron acceptor) (Contreras et al., 1999). The smaller the HOMO/LUMO energy difference is, the more favorable the electron transfer and higher the reactivity of the molecule. In addition, the distribution of boundary orbitals enables the identification of molecular regions with the greatest reactivity. Meanwhile, a higher HOMO energy is associated with a higher capacity to perform nucleophilic attacks (donate electrons), which is an important electronic parameter to describe antioxidant activity (Contreras et al., 1999; Teles Fujishima et al., 2018).
Based on available literature and potential medical applications, the present study in vitro and in silico aimed to evaluate the chemical composition, antioxidant, anti-bacterial and anti-proliferative activity of EO extracted from Laureliopsis philippiana (LP-EO).
2 Materials and methods
2.1 Collection, identification, and extraction
Laureliopsis philippiana (Looser) Schodde Mol. (Monimiaceae) leaves (the aerial parts) were collected in the Valdivian Forest (wild) at the beginning of the flowering season in September 2014 from Cutipay (39°49′52″S 73°20′27″W) Valdivia, Chile. Dr. Carlos Valdovinos identified the plant, and a specimen was deposited in the Herbarium of the Faculty of Chemical and Pharmaceutical Sciences, University of Chile (No. 22696 SQF). Fresh leaves (500 g) were used for the essential oil extraction process and the EO was obtained using the HD technique in a Clevenger-type apparatus. Anhydrous sodium sulfate was used to dry the essential oil. Samples were stored at 4 °C until their subsequent analysis, as described above (Avello Lorca et al., 2012).
2.2 Composition
The chemical characterization of the extract was performed using GC–MS analysis on a Varian series 431 gas chromatograph (Agilent Technologies, Inc., Santa Clara, CA, USA) equipped with a DB-5 ms fused silica capillary column (30 × 0.25 mm; film thickness, 0.25 µm) with split/no-split injection and coupled to a 220 series mass detector (Agilent Technologies, Inc.). The following conditions were set: helium as the carrier gas at 1.5 mL·min−1 in constant flow; injection volume: 0.8 µL, with 1:80 split ratio; injector temperature: 250 °C; oven temperature: 50–260° C at 2 °C·min−1. The electron impact ionization (EI + ) was set at 70 eV, with the ion source temperature of 260 °C. Mass spectra were recorded within a range of 40–300 atomic mass units. Terpenes in EO were identified based on retention index (RI) relative to a homologous series of n-alkanes (C5-C28; PolyScience, Niles, IL, USA) under the same experimental conditions. Standards (Eucalyptol, linalool and isosafrole Sigma-Aldrich®) and standard isolates were co-injected as identification standards. To calculate the Kovats index or linear retention index, the programmed temperature formula is used. Compounds were identified using an MS library (NIST 05 and Wiley; NIST/EPA/NIH Mass Spectral Library with Search Program, NIST data version 11; software version 2.0 g), available online at https://www.nist.gov/srd/nist1a.cfm) and comparison with previously reported MS data (Adams 2007).
2.3 Antioxidant capacity
2.3.1 Chemical materials
1,1-Diphenyl-2-picrylhydrazyl radical (DPPH), 2,4,6-tris-(2-pyridyl)-s-triazine (TPTZ), (-)-6-hydroxy-2,5,7,8-tetramethylchromane-2-carboxylic acid (Trolox), potassium persulfate (K2S2O8), H2O for HPLC, and monobasic (NaH2PO4) and dibasic (Na2HPO4) sodium phosphate were purchased from Sigma-Aldrich (St. Louis, MO, USA). Ιron (III) chloride hexahydrate (FeCl3 6H2O), iron (II) sulfate heptahydrate (FeSO4 7H2O), hydrochloric acid (HCl), methanol (MeOH), 2, 2′-azino-bis (3-ethylbenzothiazoline-6-sulfonic acid) diammonium salt (ABTS), and EDTA disodium salt were purchased from Merck (Darmstadt, Germany).
2.3.2 FRAP assay
The FRAP assay was performed using a previously described method (Karadag et al., 2009). Briefly, 10 μL of the EO solution at a final concentration of 500 μg·mL−1 (10 mg EO dissolved in 1 mL absolute EtOH and subsequently diluted to obtain a working solution of 500 μg·mL−1) (Karadag et al., 2009; Pinchuk et al., 2012) was mixed with 70 μL of freshly prepared FRAP solution at a ratio of 10:1 (v/v) [300 mM acetate buffer (pH 3.6), 10 mM 2,4,6-tris-(2-pyridyl)-s-triazine (TPTZ) dissolved in 40 mM HCl, and 20 mM FeCl3·6H2O aqueous solution). After incubating the solution for 30 min at 37 °C, absorbance was measured at 593 nm with a microplate reader (BioTek Synergy HTX Multimodal Kit; Winooski, VT, USA), and the values were interpolated onto a Trolox calibration curve over a concentration range of 0–500 μg·mL−1. FRAP results were expressed as milligrams of Trolox equivalents per gram of EO. All experiments were performed in triplicate.
2.3.3 Metal chelating activity (Ferrozine)
The chelating activity of LP_EO against iron (II) ions was assayed using the method described by Sudan (Sudan et al., 2014) with some modifications. Briefly, 50 μL of ΕΟ at a final concentration of 500 μg·mL−1 was mixed with 10 μL of 2 mM FeCl2 (prepared in HPLC-grade water). The reaction was started by the addition of 20 µL of 5 mM ferrozine (prepared in HPLC-grade water). The total volume was adjusted to 150 µL with methanol (70 µL). After incubation at 25 °C for 10 min, absorbance was measured in triplicate at 562 nm with a microplate reader (BioTek Synergy HTX Multimodal Kit). EDTA (0–250 μg·mL−1) was used as the standard control. The results were expressed as milligrams of EDTA equivalents per gram of EO.
2.3.4 DPPH radical scavenging assay
Free radical scavenging activity of LP_EO was quantified using previously described methodology (Touma et al., 2020). Briefly, 70 μL of 0.2 mM DPPH solution dissolved in methanol was mixed with 20 μL of EO or Trolox at concentrations of 0–1,000 μg·mL−1; the mixture was incubated at room temperature (22 °C) and in the dark for 30 min and then absorbance was measured with a microplate reader (BioTek Synergy HTX Multimodal Kit). All analyses were performed in triplicate, and the results were expressed as IC50 values in μg·mL−1 (defined as the concentration of EO required to inhibit 50 % DPPH radicals present in the solution).
2.3.5 ABTS radical scavenging assay
The ABTS radical scavenging activity of LP_EO was quantified following a previously described method (Touma et al., 2020). To prepare the working ABTS solution, 7 mM ABTS in PBS (pH 7.4) was mixed with 2.5 mM K2S2O8 (final concentration), followed by storage in the dark at room temperature for 16 h. After radical generation, the mixture was diluted with PBS to an absorbance of 0.700 ± 0.02 units at 734 nm. Then, 20 μL of each diluted solution of EO or Trolox as the standard in the concentration range of 0–1,000 μg·mL−1 was mixed with 180 μL of ABTS solution. Absorbance was measured 6 min after initial mixing at 734 nm with a microplate reader (BioTek Synergy HTX Multimodal Kit). All measurements were performed in triplicate. Scavenging activity was expressed as calculated IC50 values in μg·mL−1 (defined as the concentration of EO required to inhibit 50 % ABTS radicals present in the solution). As blank, 180 μL solution of ABTS moiety in 20 μL of PBS was used.
2.4 Computational methods
Density functional theory (DFT) calculations for the six compounds from LP_EO, including α-terpineol, β-eudesmol, eucalyptol, isohomogenol, isosafrole, and linalool (Fig. 1) selected for their antioxidant, antimicrobial, and antitumoral activity, were drawn using Discovery Studio (BIOVIA, Dassault Systèmes; Discovery Studio Modeling Environment 3.1. Dassault Systèmes: San Diego. 2017; Accelrys, CA). Geometries were optimized using the M06-2X-D3 method (Stewart, 2007a,b) in conjunction with the 6-31G (d, p) basis set. M06-2X-D3 is the best dispersion-corrected meta-GGA hybrid functional GMTKN30 database (Contreras et al., 1999), and it is implemented in the Gaussian16 software program suite. Water was simulated as a solvent using SMD parametrization of IEF-PCM. Some DFT-based global and local reactivity descriptors (Table 4), such as HOMO–LUMO gap, electronegativity (χ), global hardness (η), global electrophilicity index (ω), ionization potential (I), electron affinity (A), and Fukui functions, were calculated to better understand the reactivity of the molecules.
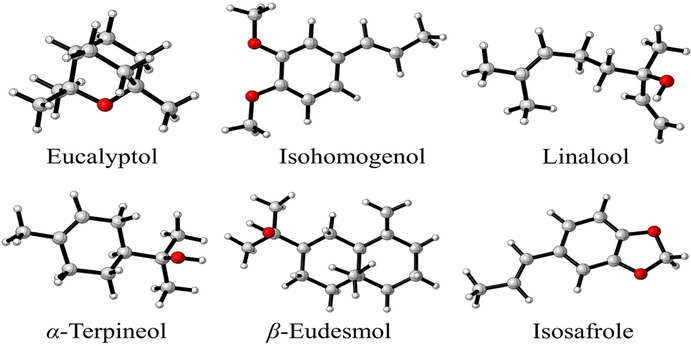
- Structures of α-terpineol, β-eudesmol, eucalyptol, isohomogenol, isosafrole, and linalool from Laureliopsis philippiana.
2.5 Antibacterial activity
2.5.1 Chemical materials and antibiotics
The DENT® antibiotic cocktail (SR014E), selective for HP growth, was purchased from Oxoid, USA (contains 10 mg·mL−1 vancomycin; 5 mg·mL−1 cefsulodin, 5 mg·mL−1 amphotericin B, and 5 mg·mL−1 trimethoprim). Other stocks of antibiotics used, including ampicillin (100 mg·mL−1) and tetracycline (12.5 mg·mL−1), as well as monoterpenes, eucalyptol, linalool, and isohomogenol (99.9 % pure) were purchased from Sigma-Aldrich®(Avello Lorca et al., 2012).
2.5.2 Microbial strains
The bacterial strains used for microbiological tests, including Staphylococcus aureus (15 clinical isolates and the reference strain ATCC 25923) and Escherichia coli (15 clinical isolates and the reference strain ATCC 25922), were obtained from the Microbiology Laboratory collection, Department of Medical Technology, Universidad Diego Portales. Moreover, 15 clinical isolates of Candida albicans and Candida parapsilosis (ATCC 22019), were used as a reference strain in the trials of the genus Candida, which were kindly provided by Dr. Alvarez, Institute of Biomedical Sciences, Faculty of Medicine, Universidad de Chile. The strains were grown on Mueller Hinton Agar at 37 °C.
From the collection of clinical isolates of the Microbial Pathogenesis Laboratory, Faculty of Medicine, Diego Portales University, Santiago, Chile, 7 strains of Helicobacter pylori were obtained. The bacteria were cultured on 1.5 % Brucella agar (from BD, USA), supplemented with 5–7 % horse blood and 1: 200-diluted DENT cocktail and incubated for 3–7 days under microaerophilic conditions (10 % CO2) and 95 % humidity at 37 °C (Ultee et al., 2002; CLSI 2015).
2.5.3 Determination of the MIC and MBC of LP_EO
To determine MIC, the microdilution method in 96-well plates was used according to the instructions approved by the Clinical and Laboratory Standards Institute (CLSI 2015). Pure LP_EO and monoterpenes, eucalyptol, linalool and isohomogenol were added in 0.5-fold decreasing serial concentrations 128, 64, 32, 16, 8, 4, 2, 1, 0.5 or 0.25 µg·mL−1 in Mueller Hinton BD® broth (MHB) in 96-well plates. The methodology also included positive and negative controls. The positive control included a well with inoculum and MHB, a medium without LP_EO or monoterpenes, and the negative control comprised a well with LP_EO or monoterpenes and MHB medium, without inoculum. Test wells with only MHB medium served as blank control. To inoculate the microplates, fresh bacterial suspensions were used (equivalent to 1 × 108 bacteria·mL−1), and the plates were incubated as previously describe (Waitz 1990; CLSI 2015). The MIC was defined as the lowest concentration of antimicrobial concentration required to inhibit the visible growth in the test tube. The MBC was defined as the lowest concentration of antimicrobial concentration required to diminish more than 99.9 % (3 Log reduction) of the initial bacterial amount at 24 h (Sukmark et al., 2011).
LP_EO was solubilized in DMSO at 2 % (v/v) (does not affect bacterial growth) and added to the previously inoculated microplates. The plates were incubated as described above for 1 day. Absorbance was measured at 405 nm to calculate MIC, as described in the Clinical and Laboratory Standards Institute (CLSI) protocol. Bacterial control microplates without EO and without bacteria were used as controls. Data were compared with ANOVA using the GraphPad Prism 5 to identify significant differences between the studied groups (Waitz 1990; CLSI 2015).
2.6 Cytotoxicity
2.6.1 Cell lines culture
The human epithelial mammary cell lines MCF10A (non-tumoral) and MCF7 (tumoral), the human epithelial renal cell lines HK-2 (non-tumoral), 786-O, and ACHN (tumoral) were obtained from American Type Culture Collection (ATCC, Rockville, MD). Glioblastoma cell lines U87GM and primary human fibroblasts were kindly provided by Dr. Gino Corsini, Universidad Autómona de Chile. The cells were cultured in Dulbecco’s modified Eagle’s/F2 medium (DMEM-F12; Gibco, USA) supplemented with 10 % fetal bovine serum (FBS; Gibco, USA) and maintained at 37 °C under 5 % CO2. When the cells reached 80 % confluence, the cultures were replicated. The numbers of cell passages was approximately 5–10. In vitro assays were performed with triplicates of three independent experiments for each cell line (Touma et al., 2020).
2.6.2 Crystal violet proliferation assay
To evaluate the dose–response at given concentrations and the time-course of EOs, the cells were detached with Trypsin–EDTA 0.5 % (Gibco, USA). Next, 5 × 103 cells·well−1 were seeded into a 96-well cell plate containing DMEM-F12 supplemented with 10 % FBS and incubated for 24 h. The supernatant was removed. The cells were washed once with 1 × PBS (Gibco, USA) and treated with known concentrations (64, 32, 16, 8, 4, or 2 µg·mL-1) of LP_EO dissolved in culture medium (DMEM-F12 supplemented with 1 % FBS). The vehicle was 1 % DMSO dissolved in DMEM-F12 supplemented with 1 % FBS as the control treatment (LP_EO was dissolved in 1 % DMSO). After 24 or 48 h, the supernatant was discarded, the cells were washed with 1 × PBS, and incubated with 100 µL of CV solution (0.2 % w/v in 10 % ethanol) (Gibco, USA) for 20 min. Then, the CV solution was removed, and Na2HPO4 (0.1 M, pH 4.5, in ethanol 50:50 v/v) (Sigma, USA) was added to elute the intra-cellular colorant. Absorbance of each sample was measured at 570 nm. The results were expressed as the percentage of color intensity and normalized to the values for cells grown in 1 % DMSO as the control treatment (Schmidt et al., 1989; De Abreu Costa et al., 2017; Touma et al., 2020).
2.6.3 MTT proliferation assay
The dose–response was assessed at known concentrations of LP_EO (64, 32, or 16 µg·mL−1) dissolved in culture medium (DMEM with 1 % FBS) or control medium (1 % DMSO in DMEM with 1 % FBS). Next, 5 × 103 cells·well−1 at 70 % confluence were incubated with DMEM-F12 supplemented with 10 % FBS for 24 h in a 96-well cell plate. After 24 or 48 h of treatment, the supernatant was discarded. The MTT solution was added to each well, and the plates were incubated at 37 °C for 2 h protected from light, according to the manufacturer’s instruction (Sigma®). Subsequently, the MTT solution was discarded, and acidified isopropanol (4 % of HCl 1 N) was added. Absorbances of each well was measured with a spectrophotometer (Thermo, USA) at 570 nm. The results were graphed, expressed as the percentage of color intensity, and normalized to control (cells grown in 1 % DMSO) values (Touma et al., 2020).
2.6.4 Statistical analysis
Independent data were analyzed using one-way ANOVA, followed by Tukey’s post hoc test. A p < 0.05 was considered as statistically significant (Touma et al., 2020).
2.7 Toxicity
2.7.1 Maintenance of Caenorhabditis elegans culture
For toxicity assays, the wild strain of Caenorhabditis elegans, N2, was used. The N2 strain was maintained on agar plates with nematode growth medium (NGM) in the presence of a layer of Escherichia coli OP50. These plates were incubated at 20 °C for 3 days. Subsequently, gravid nematodes were collected and treated in the presence of a chlorine solution (0.45 N NaOH and 2 % HOCl) to obtain eggs. To hatch the eggs and obtain synchronized adult nematodes, eggs were placed in plates with OP50 for 3 days. Finally, nematodes were collected in M9 saline solution (1.5 g KH2PO4, 3 g Na2HPO4, 2.5 g NaCl, 0.5 mL of 1 M MgSO4, and distilled water to raise the final volume to 500 mL) (Brenner 1974).
2.7.2 Test preparation
LP_EO was prepared at concentrations of 0.39, 0.78, 1.56, 3.12, 6.25, 12.5, 25, and 50.0 mg·mL−1 and added at a final volume of 100 µL to 96-well plates. Control tests were performed with 1 % DMSO and M9 saline solution. Caenorhabditis elegans (10 adult nematodes·well−1) was used in each assay. The plates were incubated at 20 °C for 24 and 48 h. The experiments were performed in triplicate and repeated twice. To determine the survival rate, all nematodes were counted at 24 and 48 h. Individuals were considered alive if they presented any motility of the tail, head, or pharynx during 5 s of observation and were considered dead otherwise. Counts were obtained to determine the mortality rate (Skantar et al., 2005).
3 Results
3.1 Composition
Chemical characterization of LP_EO using GC–MS revealed 5 oxygenated monoterpene (*) y 1 oxygenated sesquiterpene (**) (Table 1 and Fig. 2). Among these, eucalyptol was the most abundant (27.7 %), followed by linalool (27.6 %) and isosafrole (19.5 %). Isohomogenol (12.6 %), α-terpineol (7.7 %), and β-eudesmol (4.8 %) were present at lower amounts.
Fraction number | Retention time (min) | CAS | KI cal | KI lit | [M + ] | Fragment | % | Name |
---|---|---|---|---|---|---|---|---|
1 | 12.48 | 470–82-6 | – | 1030 | 154 | 139 (1 0 0) 154 (85) 27 (28) | 27.7 | Eucalyptol* |
2 | 14.76 | 78–70-6 | 1045 | 1098 | 154 | 71 (1 0 0) 93 (78) 55 (62) | 27.6 | Linalool* |
3 | 17.55 | 10482–56-1 | 1050 | 1187 | 154 | 59 (1 0 0) 93 (59) 121 (58) | 7.7 | α-Terpineol* |
4 | 20.29 | 120–58-1 | 1063 | 1298 | 162 | 162 (1 0 0) 104 (31) 78 (13) | 19.5 | Isosafrole* |
5 | 21.91 | 473–15-4 | 1555 | 1356 | 164 | 164 (1 0 0) 77 (39) 103 (38) | 4.8 | β-Eudesmol** |
6 | 23.25 | 93–16-3 | – | – | 178 | 178 (1 0 0) 163 (48) 107 (30) | 12.6 | Isohomogenol* |
Approximate retention times.
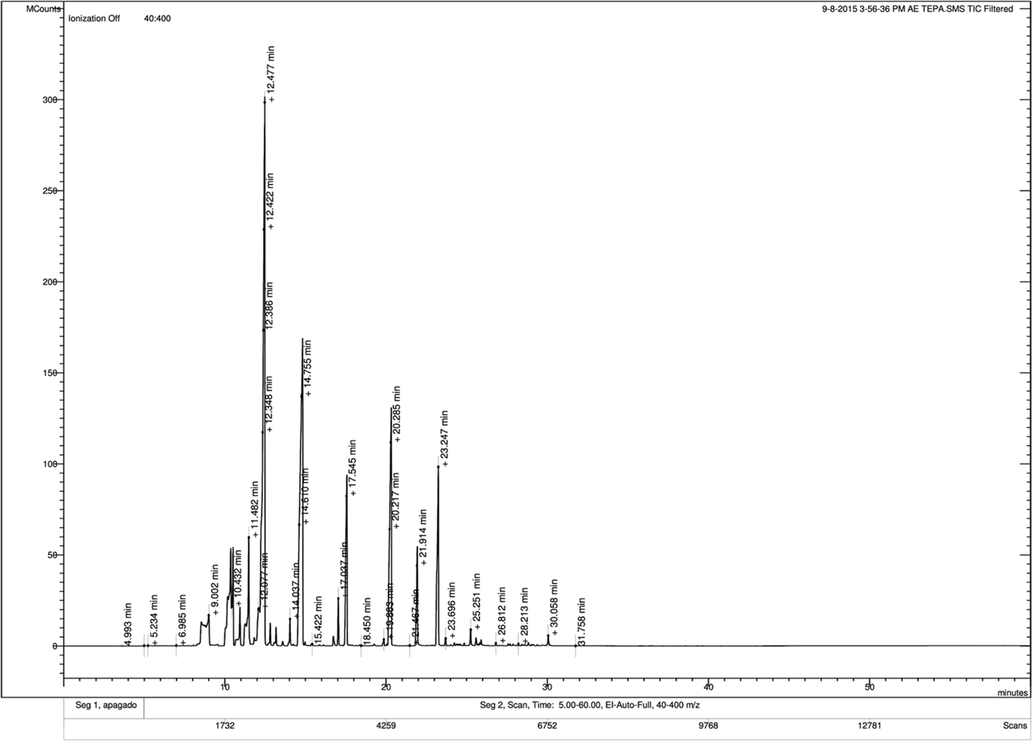
- Chromatograph of Laureliopsis philippiana essential oil.
3.2 Antioxidant activity
The antioxidant activity of LP_EO was evaluated using four complementary in vitro methods. DPPH assay evaluates antioxidant activity in organic media (particularly alcoholic media), while ABTS assay evaluates antioxidant activity of hydrophilic and lipophilic compounds at physiological pH (Moon and Shibamoto 2009). Both assays are used to evaluate the ability to donate or transfer hydrogen atoms per part of the EO component, that is, the ability to act as free radical scavengers (Huang et al., 2005). LP_EO exhibited a lower antioxidant activity as a free radical scavenger in both DPPH and ABTS assays (IC50 = >1,000 μg·mL−1 in both tests) than Trolox (IC50 = 11.7 ± 2.1 and 35.6 ± 1.5 μg·mL−1, respectively) (Table 2). Despite dose-dependent behavior (data not shown), LP_EO did not significantly inhibit DPPH• and ABTS•+ radicals.
Essential oil | FRAPa | Ferrozineb | IC50 DPPHc | IC50 ABTSc |
---|---|---|---|---|
Laureliopsis philippiana | 113.5 ± 6.0 | 57.3 ± 3.1 | >1000 | >1000 |
Trolox | – | – | 11.7 ± 2.1 | 35.6 ± 1.5 |
Values are expressed as mean ± SEM of three independent experiments.
3.3 Reactivity of LP_EO components
LP_EO is a mixture of several EOs with antioxidant, antimicrobial, or cytotoxic activities. As determined by GC–MS, eucalyptol, linalool, and isosafrole accounted for 74.8 % of LP_EO and showed similar antimicrobial activity to LP_EO. However, other EOs present at lower quantities may still contribute to bioactivity. To evaluate the individual activities of the six major components of LP_EO, quantum mechanics calculations were performed. From these calculations, the boundary orbitals and their energy were obtained, and the reactivity of the LP_EO components were evaluated based on chemical descriptors.
3.3.1 Frontier orbitals
Table 3 and Fig. 3 present the values for HOMO and LUMO energies and their differences. The order of energy gap was as follows: eucalyptol > β-eudesmol > α-terpineol > linalool > isohomogenol > isosafrole. As the energy gap decreases, the reactivity of the molecule increases, and it can be easily excited by low energy. Remarkably, isohomogenol and isosafrole have two oxygen (O) atoms that enable function as a chelating agent. Meanwhile, with a lower energy gap, isohomogenol and isosafrole would be less favorable to reduction processes. Of note, the energy gaps of isohomogenol and linalool were smaller than that of eucalyptol; thus, isohomogenol and linalool would be more reactive than eucalyptol, since these compounds are present at greater proportion in LP-EO.
Compound | EHOMO | ELUMO | ΔE | χ | Η | Ω | I | A |
---|---|---|---|---|---|---|---|---|
Eucalyptol | −8.11 | 2.89 | 10.99 | 2.61 | 5.50 | 0.62 | 8.11 | −2.89 |
Isohomogenol | −6.69 | 0.35 | 7.03 | 3.17 | 3.52 | 1.43 | 6.69 | −0.35 |
Linalool | −7.71 | 1.52 | 9.22 | 3.09 | 4.61 | 1.04 | 7.71 | −1.52 |
α-Terpineol | −7.64 | 2.05 | 9.69 | 2.79 | 4.85 | 0.81 | 7.64 | −2.05 |
β-Eudesmol | −7.99 | 1.78 | 9.76 | 3.11 | 4.88 | 0.99 | 7.99 | −1.78 |
Isosafrole | −6.80 | 0.19 | 6.99 | 3.30 | 3.50 | 1.56 | 6.80 | −0.19 |
Compound | Log P | MW (g·mol−1) | TPSA (Å2) | HBA | HBD | RB | Log S | log Kp (cm·s−1) |
---|---|---|---|---|---|---|---|---|
Linalool | 2.29 | 140.22 | 20.23 | 1 | 1 | 4 | −2.41 | −5.49 |
Isohomogenol | 2.78 | 178.23 | 18.46 | 2 | 0 | 3 | −3.47 | −4.97 |
Eucalyptol | 2.67 | 154.25 | 9.23 | 1 | 0 | 0 | −2.59 | −5.30 |
Isosafrole | 2.68 | 162.19 | 18.46 | 2 | 0 | 1 | −3.73 | −4.70 |
β-Eudesmol | 3.61 | 222.37 | 20.23 | 1 | 1 | 1 | −3.86 | −5.00 |
α-Terpineol | 2.58 | 154.25 | 20.23 | 1 | 1 | 1 | −3.49 | −4.83 |
MW = 150–500 g·mol−1; TPSA = 20–130 Å2; HBA = N° ≤ 10; HBD = N° ≤ 5; RB = 0–9. Log S = Insoluble < -10 < Poorly soluble < -6 < Moderately soluble < -4 < Soluble < -2; Log P ≤ 5; log Kp ≥ -2.5 considered permeable; N°: Violations of Lipinskís rule is zero.
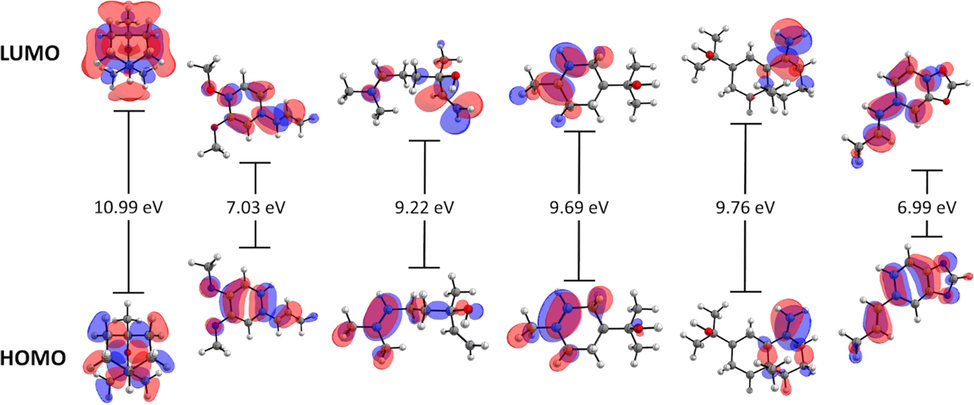
- Frontier molecular orbitals and HOMO-LUMO energy gap of eucalyptol, isohomogenol, linalool, α-terpineol, β-eudesmol, and isosafrole at the M06-2X-D3/6-31G (d, p) level of theory.
3.3.2 Chemical descriptors
Based on the frontier molecular orbital theory, Koopman’s approximation can calculate several chemical descriptors. These descriptors are useful for predicting the reactivity of both atoms and molecular systems and are divided into two groups: global and local descriptors. The former are particularly useful for analyzing the reactivity of a molecule in its entirety, while the latter allow for the analysis of specific regions within molecular species. Ionization energy (I) and electron affinity (A) are probably the most used descriptors, and these are complemented by the local descriptors electronegativity (χ), global hardness (η), and global electrophilicity index (ω). Table 4 presents the chemical reactivity descriptors for eucalyptol, isohomogenol, linalool, α-terpineol, β-eudesmol, and isosafrole using the Koopman’s approach. For isohomogenol, α-terpineol, and isosafrole, the ionization energy was low, indicating that these species are better electron density donors. Meanwhile, for eucalyptol, β-eudesmol, and linalool, electron affinity was more negative than the other compounds, indicating that these species would hardly accept more electrons. This was also reflected in their electronegativity values relative to other compounds. Moreover, isosafrole and isohomogenol molecules showed lower hardness values than eucalyptol, α-terpineol, and β-eudesmol, indicating that they are less averse to electron arrival and are thus more reactive systems.
To simultaneously present electrophilic and/or nucleophilic activation at different sites of molecules, the Koopman’s approach, which is based on the molecular border orbital theory, calculates global (as mentioned above) and local reactivity indices. For this kind of analysis, the Fukui functions are used (Fukui 1982). In addition to their use for predicting reactive sites for nucleophilic, electrophilic, and radical attacks, these functions can be interpreted as a measure of the sensitivity of the chemical potential of a system to change in the external potential or a measure of how electron density varies as the number of electrons in the system increases. Atoms with a marked volume on the isosurface are the identified sites for nucleophilic attack. Eucalyptol, isohomogenol, and isosafrole present O atoms as part of the reactive sites, whereas linalool, α-terpineol, and β-eudesmol present aromatic rings and hydrocarbon chains. In all molecules, atoms present in parts of aromatic rings and hydrocarbon chains were identified as electrophilic attack sites, highlighting the isosurface volume level of the O atoms of isohomogenol and isosafrole as reactive sites. Considering the potential application of the tested molecules as antioxidants, it is interesting to elucidate the active sites for radical attack. The local radical indices showed O atoms as part of the reactive site in eucalyptol, isohomogenol, and isosafrole. These results are consistent with previously reports that eucalyptol acts as an organic chelating agent and a DNA antioxidant protector (Horvathova et al., 2014).
3.3.3 Electrostatic potential maps (EPMs)
EPMs were developed to represent the distribution of electrons in the molecule (Fig. 4). These maps allow the prediction of physicochemical properties and provide information on the shape and nature of electrostatic forces (i.e., whether they are positive, negative, or neutral). Based on electrostatic distribution, EPMs allow for the estimation of whether an electrophilic or a nucleophilic attack is favorable during interaction with other molecules or radicals. The positive regions (blue/green) indicate the portion of the molecule most likely to undergo nucleophilic attack, while the negative regions (green/red) indicate regions most likely undergo electrophilic attack (Parr and Yang 1984; Teles Fujishima et al., 2018). EPMs of eucalyptol, isohomogenol, linalool, α-terpineol, β-eudesmol, and isosafrole molecules showed negative zones (red color) of electrostatic potential in the position of the hydroxyl (–OH) and oxygen (O) atoms, which are favored for electrophilic attacks. In EPMs of isohomogenol and isosafrole, aromatic rings presented a slight negative zone, which can facilitate π–π stacking interactions. Thus, the attacking electrophile would be attracted to the negatively charged sites. Notably, in isohomogenol, the O atoms act as a chelating region for metals, making it a chelating-susceptible molecule. These differences in the potential and charges around compounds may primarily be responsible for variations in their antioxidant activity.
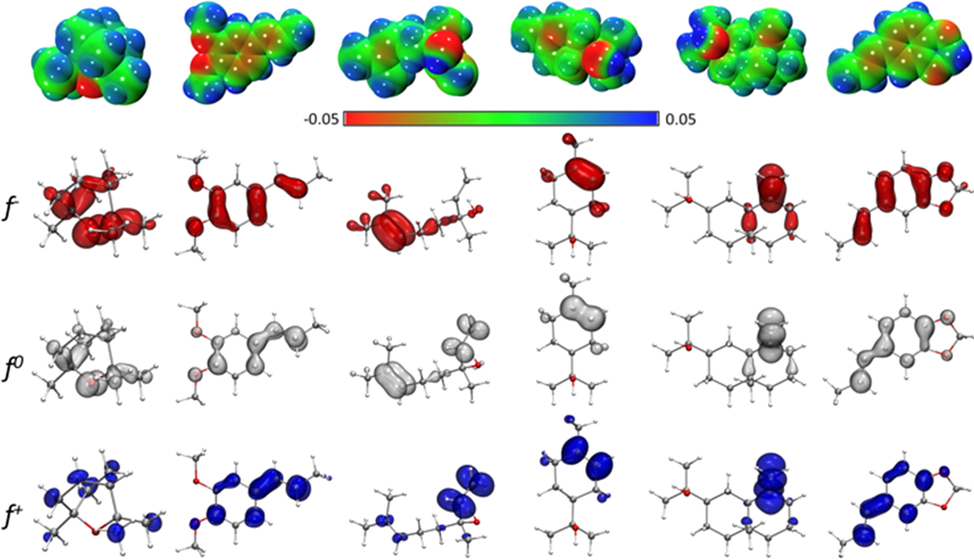
- Electrostatic potential maps (in a.u.) and graphical representation of the electrophilic f-, radical f0, and nucleophilic f + Fukui functions of eucalyptol, isohomogenol, linalool, α-terpineol, β-eudesmol, and isosafrole. All isosurfaces were generated at a 0.0025 a.u. at the M06-2X-D3/6-31G (d, p) level of theory.
3.3.4 In silico pharmacokinetic prediction
A good drug candidate is absorbed in required time and distributed throughout the organism for effective metabolism and action. To assess the viability of a compound as a drug, six physicochemical properties, together called the ADME-Tox parameters, are calculated: lipophilicity, size, polarity, solubility, flexibility, and saturation. In addition, the toxicity of the tested compound must be below a threshold, and compounds exceeding this threshold can be discarded due to their high toxicity, regardless of acceptable ADME-Tox parameters. To assess the viability of the six monoterpenes detected in LP_EO, the online tool SwissADME was used for calculating molecular descriptors based on their similarity to other synthesized drugs (Contreras et al., 1999) (Table 4).
The propensity of the six terpenes to be inhibitors of pharmacokinetic-related proteins, mainly P-glycoprotein 1 (P-gp) and major cytochrome P450 (CYP) isoforms, was evaluated. Since P-gp is a membrane protein that expels compounds from the cell, it is critical that the new drugs are not substrates for P-gp. As shown in Table 5, all the terpenes studied in silico met this condition. Further, CYP is involved in the oxidative metabolism of many xenobiotics and molecules, and these enzymes are necessary for the compound to be eliminated from the system in a given time. In this regard, the ability of the six terpenes to act as the major cytochrome inhibitors P450 (CYP) isoforms was predicted. As shown in Table 5, none of the terpenes studied inhibited the CYP isoforms tested. Since the qualitative in silico study predicted that LP_EO could inhibit CYP1A2 and CYP2C9, the test was extended to other isoforms using a quantitative methodology. Briefly, molecular docking was used to assess the EOs affinity to crystallized CYP isoforms and identify CYP residues that could stabilize binding. The CYP1A2 isoform showed high affinity to EOs (binding energy between −4.4 and −8.8 kcal·mol−1), while β-eudesmol could interact with the CYP1A2 (-8.8 kcal·mol−1), CYP51 (-9.0 kcal·mol−1), CYP2C9*2 (-8.3 kcal·mol−1) isoforms (These calculations are provided in the Supplementary Material Figure S1, Table S1, and Figure S2). Based on these results, in vitro studies can be designed to identify the target proteins of EOs and propose the underlying mechanisms of action.
Compound | HIA | BBB | P-gp substrate | CYP1A2 inhibitor | CYP2C19 inhibitor | CYP2C9 inhibitor | CYP2D6 inhibitor | CYP3A4 inhibitor |
---|---|---|---|---|---|---|---|---|
Linalool | High | Yes | No | No | No | No | No | No |
Isohomogenol | High | Yes | No | Yes | No | No | No | No |
Eucalyptol | High | Yes | No | No | No | No | No | No |
Isosafrole | High | Yes | No | Yes | No | No | No | No |
β-Eudesmol | High | Yes | No | No | No | Yes | No | No |
α-Terpineol | High | Yes | No | No | No | No | No | No |
HIA: gastrointestinal absorption; BBB: blood–brain barrier permeation; P-gp: permeability glycoprotein; CYP1A2, CYP2C19, CYP2C9, CYP2D6, and CYP3A4 are the five major isoforms of cytochromes P450.
Furthermore, all six molecules fulfilled Lipinski's rule (24), which predicts the feasibility of a compound to be used as a drug based on empirical observations. ADMET properties were highly similar among the six molecules, indicating that they can be used in advanced clinical trials (Fig. 5).
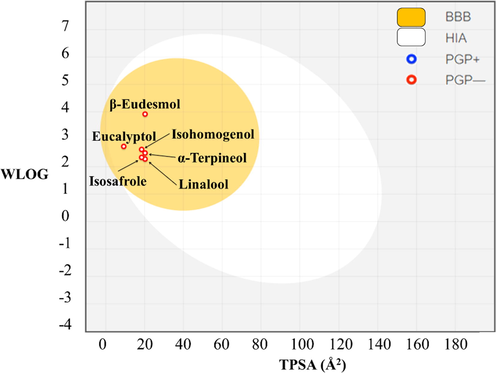
- Brain (BBB) or intestinal (Frisch et al., 2016) estimated permeation method (BOILED-egg) plot of the six compounds.
Membrane permeability of a compound depends mainly on its size and hydrophobicity. Small, hydrophobic compounds can better cross membranes than large, hydrophilic ones. Based on the physicochemical properties of the six terpenes predicted using SwissADME, the capacity of each terpene to permeate the intestinal (Frisch et al., 2016) or encephalic (BBB) membrane was determined. As shown in Fig. 5, the intestinal and hematoencephalic membranes were permeable to the six terpenes studied, and these terpenes would not be eliminated from the system through P-gp.
3.4 Antimicrobial activity
The results of antibacterial and antifungal activity tests of LP_EO are presented in Table 6. The compounds analyzed were obtained from Sigma. The majority compounds included eucalyptol, linalool, and isosafrole. However, since isosafrole could not be obtained in Sigma, isohomogenol was considered in the test. The MIC and MBC of LP_EO, linalool, eucalyptol, and isohomogenol against Helicobacter pylori was 64 μg·mL−1 and > 128 μg·mL−1 (strains). Moreover, the MIC and MBC of LP_EO was 32 μg·mL−1 and > 64 μg·mL−1, for monoterpenes 64 μg·mL−1 and > 128 μg·mL−1 respectively against Staphylococcus aureus (clinical isolates). Against Escherichia coli (clinical isolates), the MIC and MBC, of LP_EO, linalool, eucalyptol, and isohomogenol were 8–16, 19–38, 34- >64, and 62- >64 μg·mL−1 respectively. Meanwhile, against Candida albicans (clinical isolates), the MIC and MBC of LP-EO and each of the evaluated compounds were 64 and > 128 μg·mL−1. LP_EO (MIC 8 and MBC 16 μg·mL−1) was more effective than linalool (MIC 19 and MBC 38 μg·mL−1), eucalyptol (MIC 32 and MBC > 64 μg·mL−1) and isohomogenol (MIC 62 and MBC > 64 μg·mL−1) against Escherichia coli.
EO or its purified component | MIC (μg·mL−1) Helicobacter pylori | MBC (μg·mL−1) Helicobacter pylori | MIC (μg·mL−1) Staphylococcus aureus | MBC (μg·mL−1) Staphylococcus aureus | MIC (μg·mL−1) Escherichia coli | MBC (μg·mL−1) Escherichia coli | MIC (μg·mL−1) Candida albicans | MBC (μg·mL−1) Candida albicans |
---|---|---|---|---|---|---|---|---|
LP_EO | 64 | >128 | 32 | >64 | 8 | 16 | 64 | >128 |
Linalool | 64 | >128 | 64 | >128 | 19 | 38 | 64 | >128 |
Eucalyptol | 64 | >128 | 64 | >128 | 34 | >64 | 64 | >128 |
Isohomogenol | 64 | >128 | 64 | >128 | 62 | >64 | 64 | >128 |
ANOVA followed by Tukey's test did not show significant differences between the purified compounds of linalool, eucalyptol and isohomogenol. The values represent the average of 15 clinical isolates (obtained from the Chilean population) for Staphylococcus aureus, Escherichia coli, and Candida albicans and 7 isolates for Helicobacter pylori. MIC = the lowest concentration of antimicrobial concentration required to inhibit the visible growth of bacteria tested in the test well. MBC = the lowest concentration of antimicrobial concentration required to diminish at least 99.9 % (3 Log reduction) of the initial bacterial amount at 24 h. The experiment was carried out in triplicate and the modal values are shown.
3.5 Antiproliferative activity
LP_EO presented a selective inhibitory effect on human epithelial tumor cells. To assess the selective cytotoxicity and antitumor activity of LP_EO, the mammary tumor cell line MCF-7 was incubated with different concentrations of LP_EO, and the treated cells were subjected to the MTT assay. The proliferation of MCF-7 cells treated with different concentrations (64–2 μg·mL−1) of LP_EO was inhibited (vs dimethyl sulfoxide, DMSO). There were no significant differences among treatments at lower concentrations (16–2 μg·mL−1) (Fig. 6).
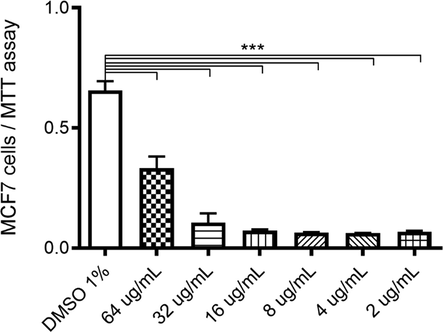
- Dose–response assay of human epithelial mammary tumor cell line MCF-7 treated with LP_EO. The bar graph presents the proliferation of MCF7 cells treated with LP_EO at different concentrations versus control (DMSO 1 %) for 48 h, as evaluated using the CV assay at 570 nm. Three independent experiments, in triplicate, were performed for each treatment and concentration. ***p < 0.001.
Furthermore, the human mammary tumor cell line MCF-7 and the human mammary non-tumor cell line MCF10A were incubated with LP_EO at 32 and 64 μg·mL−1 and the effects on proliferation were assessed using the crystal violet (CV) assay. Both concentrations, LP_EO significantly inhibited the proliferation of MCF-7 cells (Fig. 7a). Conversely, in MCF10A cells, no significant differences were noted in proliferation among treatments (Fig. 7b).
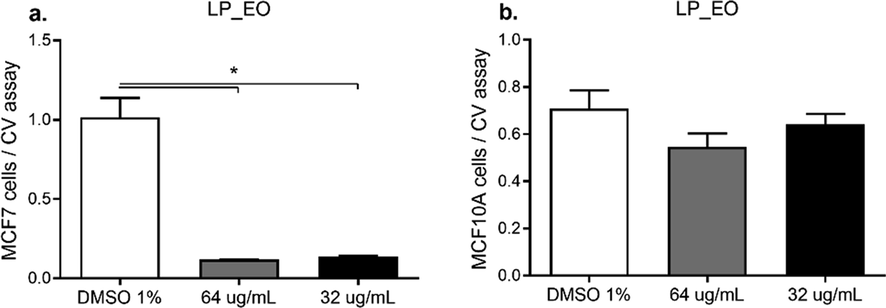
- Dose–response assay of human epithelial mammary tumor cell line MCF-7 (a) and non-tumor human epithelial cell line MCF10A (b) treated with LP_EO. The bar graph presents the proliferation of both cell lines incubated with LP_EO at different concentrations versus control (DMSO 1 %) for 48 h, as evaluated using the CV assay at 570 nm. Three independent experiments, in triplicate, were performed for each treatment and concentration. ***p < 0.001.
Next, non-tumoral (HK-2) and tumoral (786-O and ACHN) human renal epithelial cells were exposed to LP_EO, and dose–response curves were assessed (32 and 64 µg·mL−1). Contrary to the effect observed in non-tumoral mammary cells, LP_EO showed an inhibitory effect on the proliferation of all renal epithelial cell lines (Fig. 8a). In primary tumor (786-O) and metastatic site (ACHN) renal cell lines, LP_EO significantly reduced cell viability and proliferation (Fig. 8b and 8c, respectively).
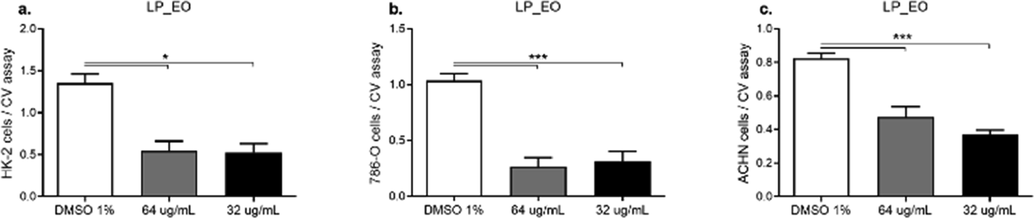
- Dose–response assay of human epithelial renal non-tumor cell line HK-2 (a), tumor cell lines 786–0 (b), and ACHN (c) treated with LP_EO. The bar graph presents to the proliferation of HK-2, 786-O, and ACHN cells treated with LP_EO at different concentrations versus control (DMSO 1 %) for 48 h, as evaluated using the CV assay at 570 nm. Three independent trials, in triplicate, were performed for each treatment and concentration. ***p < 0.001.
3.6 Toxicity
LP_EO exhibited low toxicity against Caenorhabditis elegans in the range of concentrations from 0.39 to 1.56 mg·mL−1 (Fig. 9); however, with increase in concentration from 3.12 to 50 mg·mL-1, toxicity increased in a dose-dependent manner, with concentrations of 25 and 50 mg·mL−1 being highly toxic at 24 h (Fig. 9a) of exposure. At 48 h (Fig. 9b), toxicity increased at concentrations of 12.5 to 3.12 mg·mL−1. Ivermectin (nematicide) was used as a lethality control (positive control), killing 100 % of worms at a concentration of 0.3 mg·mL-1 (data not shown). Similar findings have been reported in other studies on EOs extracted from Chilean herbs, with only the highest concentrations being toxic (50, 25, or 12.5 mg·mL−1), confirming that these EOs present low toxicity (Larrazabal-Fuentes et al., 2019; Touma et al., 2020).
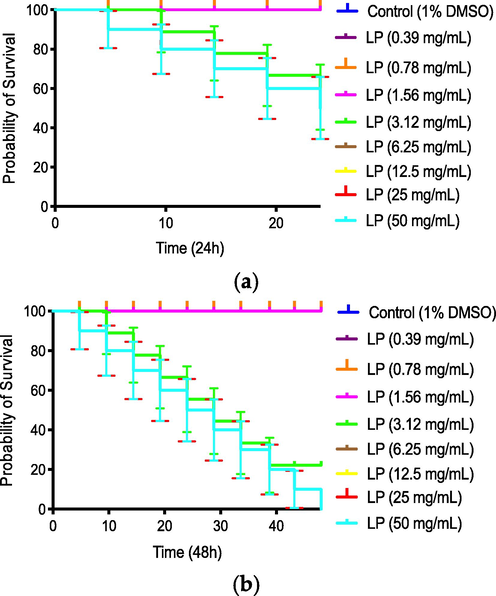
- Percent survival of Caenorhabditis elegans expose to Laureliopsis philippiana (LP) essential oil for 24 h (a) and 48 h (b).
4 Discussion
The chemical characterization of LP_EO by GC–MS yielded five compounds, the most abundant being eucalyptol (27.7 %), followed by linalool (27.6 %) and isosafrole (19.5 %) (Table 1, Fig. 2). Finding differences with other studies, Bittner and collaborators (Bittner et al., 2009), detected as majority compounds of EOs from LP leaves, 3-carene (53.81.9 %), eucalyptol (14.76 %), and 1,2-dimethoxy-4-(2-propenyl)-phenol (10.58 %), which were collected in June 2003 from the Huachi sector of Santa Bárbara (37°31′S, 71°51′W), Bío-Bío Region, Chile. In another study by Herrera-Rodríguez and colleagues (Herrera-Rodríguez et al., 2015), safrole (64.7 %), methyleugenol (14.6 %), and eucalyptol (1.4 %) were detected as majority compounds, leaves were collected from Los Lleuques zone (36°51′18″ S, 71°38′34″ W; 286 m a.s.l.), Ñuble province foothills, Chile, the chemical characterization of this study presented a higher % of eucalyptol.
A greater amount of terpenes registered Toledo and colleagues (Toledo et al., 2014) determined the chemical composition of LP_EO from leaves, identified 19 compounds, representing 98.8 % of the oil composition. The oxygenated monoterpenes eucalyptol and linalool were the major constituents of the oil, accounting for 37.4 % and 32.3 % of the total composition, respectively; the material was collected during March 2008 from the Rucamanque experimental field of the University of La Frontera. The basin and slopes of the Rucamanque Valley are covered by native forest, which is evergreen in the lower areas and partially deciduous at higher elevations. The climate of the area is humid and temperate with a Mediterranean influence. These differences may explain the higher abundance of eucalyptol and linalool in the previous work than in the present study (27.7 % and 27.6 %, respectively). Madrid and their group (Madrid et al., 2015) characterized LP_EO from leaves and detected safrole (96.92 %) and β-phellandrene (1.80 %) as the major constituents. In that study, the authors collected leaves in December 2012 from Antilhue, Los Ríos Region, Chile.
Although the collected samples were from the overlapping region, the differences in the chemotype of LP_EO could be attributed to the difference in the collection time. The sample in this study was collected in 2016 from a region geographically close to the coastal area in Niebla, whereas Madrid and collaborators collected samples in 2012 from a region geographically on the south bank of the Calle-Calle River in the border with the commune of Los Lagos. Thus, variations in the quantity and quality of components of the same species, may be due to different stages of development and harvest times (Saharkhiz et al., 2016) as well as to due to differences in genotype, climatic conditions, agricultural practices, and geographic and seasonal factors (Muñoz-Bertomeu et al., 2007).
The observed antioxidant activity in terms of radical scavenging is consistent with the composition of LP_EO. Specifically, three of its components, namely α-terpineol, linalool, and eudesmol, are capable of donating hydrogens to stabilize free radicals, and these components account for approximately 40.1 % of the total composition. Of these, α-terpineol (7.7 %) and linalool (27.6 %) are monoterpene alcohols, while eudesmol (4.8 %) is a sesquiterpene alcohol. Previous studies have reported that linalool and α-terpineol present a markedly lower antioxidant activity as isolated products compared with the overall oil (Baschieri et al., 2017; Larrazabal-Fuentes et al., 2019; Touma et al., 2020). This may be explained by the fact that the synergistic and antagonistic effects of different metabolites in the oil and their interactions with one another determine antioxidant activity (Caesar and Cech 2019).
The reducing ability of EO components, as potential substances that can transfer electrons to stabilize free radicals, was measured using the ferric reducing antioxidant power (FRAP) assay (Karadag et al., 2009). EO-LP showed high antioxidant activity in the FRAP assay (113.5 ± 6.0 mg Trolox equivalent·g−1 EO). However, this activity was 50 % lower than reported by other investigators in other species of the same family (Mohammed et al., 2022). These results are consistent with the chemical composition of LP_EO where α-Terpineol, linalool, and eudesmol are present, these structural features and together constitute 40.1 % of the total LP_EO composition. Additionally, the two aromatic derivatives isosafrole (19.5 %) and isohomogenol (12.6 %) possess aliphatic regions (Horvathova et al., 2014; Baschieri et al., 2017; Caesar and Cech 2019). Based on the FRAP assay, antioxidant activity is mainly conferred by the ability of LP_EO components to form complexes with metals, such as iron, which is involved in the Fenton reaction, inducing lipoperoxidation and subsequent cell damage (Karadag et al., 2009; Pinchuk et al., 2012).
LP_EO showed moderate chelating activity against the ferrozine assay (57.3 ± 3.1 mg EDTA equivalent·g−1 EO). However, the value obtained for LP_EO is higher than those reported for AE from other medicinal plants with important biological and antioxidant activities (Asghari et al., 2018; Mohammed et al., 2022). Therefore, LP_EO can act as a powerful Fe+2 chelator in cellular processes involving free radicals and prevent the progression and exacerbation of oxidative stress. This LP_EO activity can be attributed to the ability of hydroxylated terpenes (–OH groups) to form complexes with Fe+2. Linalool, eudesmol and α-terpineol in LP_EO possess this characteristic. Similar observations have been reported on essential oils from plants of Mediterranean origin (Mohammed et al., 2022).
To evaluate the individual contribution of each component in the oil, the chemical reactivity and antioxidant, antimicrobial and antitumor activity were studied in silico. Meanwhile, with a lower energy gap, isohomogenol and isosafrole would be less favorable to reduction processes. Of note, the energy gaps of isohomogenol and linalool were smaller than that of eucalyptol; thus, isohomogenol and linalool would be more reactive than eucalyptol, since these compounds are present at a greater proportion in Laureliopsis philippiana (Table 3 and Fig. 3).
The HOMO–LUMO energy gap is consistent with the higher reactivity of the aromatic terpenes isosafrole (19.5 %) and isohomogenol (12.6 %). Thus, based on ionization energy, electronic affinity, electronegativity, overall hardness, and overall electrophilicity index, aromatic terpenes appear to be more reactive than cyclic monoterpenes with conjugated double bonds (Parr and Yang 1984).
From the analysis of LUMO energies and chemical descriptors obtained, LP_EO terpenes that may provide the highest antimicrobial activity were identified (Parr and Yang, 1984). Considering that the antimicrobial activity increases with HOMO orbital energy and decreases with LUMO orbital energy, the antimicrobial activity of the six LP_EO terpenes could be ordered as follows: isosafrole > β-eudesmol > α-terpineol > linalool > isohomogenol > eucalyptol. According to this result, isosafrole > β-eudesmol. Corresponding to this analysis, isosafrole, isohomogenol and linalool contributed the most to the antimicrobial activity of LP_EO [Antimicrobial Activity and DFT Studies of a Novel Set of Spiropyrrolidines Tethered with Thiochroman-4-one/Chroman-4-one Scaffolds].
In addition, an ADME-Tox analysis (Table 4) was performed to evaluate the viability of the six LP_EO terpenes to be used as pharmaceuticals. Through the analysis, it is observed that all the compounds analyzed complied with Lipinski's rules, in addition to showing good intestinal and blood–brain barrier (BBB) permeability (Table 5 and Fig. 5). In this regard, according to the estimations made, ECs are not substrate for P-gp and do not inhibit several CYP isoforms (CYP2C19, CYP2D6 and CYP3A4) (Table 5), so they might have low toxicity. Overall, the tested compounds have high feasibility to be use as drugs (Supplementary Material Figure S1, Table S1 and Figure S2).
LP_EO, linalool and eucalyptol presented activity (MIC and MBC) against clinical isolates of Helicobacter pylori, Staphylococcus aureus, Escherichia coli and Candida albicans, showing a greater effect on gram-negative bacteria such as Escherichia coli (Table 6), the LP_EO effect against Escherichia coli was stronger than that of linalool.
The antibacterial activity against gram-positive and gram-negative bacteria presented in this study is higher than that described by other researchers (Toledo et al., 2014). As seen in Table 6, the MIC and MBC of E. coli for LP_EO (8 and 16 μg·mL−1) is lower than for the majority components (linalool 19 and 38 μg·mL−1, eucalyptol 34 and > 64 μg·mL−1). This indicates antimicrobial activity, which is consistent with the theoretical high chemical reactivity of some compounds such as Isosafrole,
The results obtained for LP_EO and its major monoterpenes (e.g., linalool, eucalyptol, and isohomogenol) presented the same activity against the clinical isolates of Candida albicans. Other authors have also reported antifungal activity of the LP_EO against other microorganisms such as Phragmidium violaceum, Fusarium oxysporum and Rhizoctonia solani (Bittner et al., 2009).
The results obtained in the antimicrobial activity of LP_EO can be attributed to the presence of eucalyptol and linalool as the main component. This is in accordance with the evidence presented in other studies, where it was observed that terpinen-4-ol and eucalyptol destabilize fungal and plant membranes, respectively. This finding may explain the effect of LP_EO and eucalyptol against Candida albicans (Hąc-Wydro and Szydło 2016). Also demonstrated the inhibitory effects of LP_EO and linalool anti-biofilm activity against Candida albicans (Manoharan et al., 2017). The higher antimicrobial activity of LP-EO compared to the main monoterpenes could be consistent with a synergistic effect of its components, as has been shown in other essential oils (Liu et al., 2015; Jafri and Ahmad 2020).
The results show that LP-EO exerts a selective inhibitory effect on the proliferation of human epithelial tumor cell lines with respect to non-tumor epithelial cell lines. Being more evident in human mammary tumor cell lines. This antiproliferative activity can be attributed to the main compounds present in the essential oil as reported in previous studies. (Sharma et al., 2022).
This results are consistent with various authors who reports similar effects of EO of the same plant species (Montenegro et al., 2014; Russo et al., 2019) and other members of the Winteraceae family (Allouche et al., 2009). In fact, plant-based products exhibited excellent potential to inhibit the cell proliferation, invasion, and metastasis and induce cell apoptosis in experimental cancer models, either alone or in combination with other natural products or as adjuvants to chemotherapeutic agents (Magalhães et al., 2021). The potential use of natural products (EOs and phenolic compounds) as therapeutic agents for diverse tumor types (e.g., breast and renal cancer) has been explored (Elshafie and Camele 2017; Sharifi-Rad et al., 2017), and the most described methods of action and molecular targets of these products include key signaling pathways involved in the regulation of cell proliferation, cell cycle regulation, and apoptosis (Magalhães et al., 2021). Future studies should verify the mechanisms of action of LP_EO and its link to the apoptotic pathway.
In this study, a low toxicity of LP_EO against Caenorhabditis elegans was found. Previous studies against C. elegans were not found, but Ortiz and collaborators (Ortiz et al., 2012) evaluated the insecticidal effect of Laureliopsis philippiana leaf dust against the corn weevil under laboratory conditions. The highest insect mortality was recorded with treatments containing 1 %, 2 % and 4 % leaf powder, with rates of 94.7–100 %, and adult emergence was 0 % at these concentrations. The insecticidal effect of the powder was maintained for 14 days. In future studies, the biopesticide effect on other pests that affect crops in this country may be evaluated.
In general, the present characterization of LP_EO highlighted three main monoterpenes: eucalyptol (27.7 %), linalool (27.6 %) and isosafrole (19.5 %). LP_EO presented antioxidant activity in four tests (FRAP, DPPH, metal chelating activity and ABTS tests), the in-silico calculations allowed to infer the possible mechanisms through which the different free radicals are inactivated. In addition, LP_EO exhibited antimicrobial effects, with the essence and its main compounds showing similar MICs against clinical isolates of Helicobacter pylori, Staphylococcus aureus, and Candida albicans. However, the LP_EO effect (MIC 8 and MBC 16 μg.mL−1) was more effective than linalool (MIC 19 and MBC 38 μg.mL-1), eucalyptol (MIC 32 and MBC > 64 μg.mL−1) and isohomogenol (MIC 62 and MBC > 64 μg.mL−1) against Escherichia coli. According to HOMO/LUMO calculations, energies and physicochemical properties of the six terpenes, isohomogenol and linalool are more reactive than eucalyptol, which is the most abundant compound by mass in LP_EO. In addition, all the LP_EO terpenes analyzed showed a high viability to be used as drugs according to the ADME-Tox parameters. In very interesting result is LP_EO selective inhibitory effect on the proliferation of epithelial tumor cell lines. Likewise, the low toxicity of LP_EO against Caenorhabditis elegans, this effect was dose dependent; concentrations>25 and 50 mg·mL−1 were more toxic at 24 h of exposure.
These results will allow projecting the applications of LP_EO in the field of pharmacological treatments for veterinary and human application, cosmetics, or sanitation.
5 Conclusions
Characterization of LP_EO highlighted three major monoterpenes, including eucalyptol, linalool, and isosafrol. LP_EO presented antioxidant activity. LP_EO and its main components presented antimicrobial effects on clinical isolates of Helicobacter pylori, Staphylococcus aureus, and Candida albicans; the effect of LP_EO against clinical isolates of Escherichia coli stand out and was greater than that of linalool and eucalyptol. The chemical reactivity estimates are consistent with relevant antimicrobial activity of less abundant compounds of LP_EO. LP_EO presented activity anti-proliferative against tumor cell lines and low toxicity against Caenorhabditis elegans. Future studies should include in vivo tests addressing pharmacological and toxicological aspects, advance formulations based on the present extract, and perform synergy analysis of the majority LP_EO components and the antimicrobial mechanisms of the LP_EO. This could help to understand and demonstrate their efficacy and contribution to the biological activity of LP_EO as antimicrobial, antitumor, and antiparasitic agents, among others. These results will allow projecting the applications of LP_EO, being able to be used as a source of bioactive compounds in novel pharmacological treatments for veterinary and human application, cosmetics, or sanitation.
CRediT authorship contribution statement
Flavia Bruna: Methodology, Investigation, Writing – review & editing, Funding acquisition. Katia Fernández: Methodology. Felipe Urrejola: Investigation. Jorge Touma: Methodology, Investigation. Myriam Navarro: Methodology, Investigation. Betsabet Sepúlveda: Investigation. María Larrazabal-Fuentes: . Adrián Paredes: Methodology, Investigation, Writing – original draft, Writing – review & editing, Funding acquisition. Iván Neira: Methodology, Investigation, Writing – review & editing, Funding acquisition. Matías Ferrando: Investigation, Writing – review & editing, Funding acquisition. Manuel Osorio: Writing – review & editing, Funding acquisition. Osvaldo Yáñez: Writing – review & editing, Funding acquisition. Jessica Bravo: Conceptualization, Investigation, Writing – original draft, Writing – review & editing, Funding acquisition.
Acknowledgments
Dr. Eduardo Álvarez, Instituto de Ciencias Biomédicas, Facultad de Medicina, Universidad de Chile. Pedro Cortes TM, Facultad de Salud y Odontología, Universidad Diego Portales. Dr. Gino Corsini of the Universidad Automona de Chile. To our dear colleague and friend, Dr. Alejandro Venegas (1945-2021), who was an important part of this work and who taught us how to work with Helicobacter pylori, among other things.
Funding
This work was supported by Universidad Diego Portales (academic projects).
Declaration of Competing Interest
The authors declare that they have no known competing financial interests or personal relationships that could have appeared to influence the work reported in this paper.
References
- Identification of essential oil components by gas chromatography/quadrupole mass spectroscopy. Allur. Publ. Corp.: Carol Stream. 2007;16:65-120.
- [Google Scholar]
- Cytotoxic sesquiterpenoids from Winteraceae of Caledonian rainforest. Phytochemistry. 2009;70:546-553.
- [CrossRef] [Google Scholar]
- Influence of some citrus essential oils on cell viability, glutathione –S-transferase and lipid peroxidation in Ehrlich ascites carcinoma cells. J. Am. Sci.. 2010;6
- [Google Scholar]
- Amylase, glucosidase, tyrosinase, and cholinesterases inhibitory, antioxidant effects, and GC-MS analysis of wild mint (Mentha longifolia var. calliantha) essential oil: a natural remedy. Eur. J. Integr. Med.. 2018;22:44-49.
- [CrossRef] [Google Scholar]
- Efectos antimicrobianos de extractos de plantas chilenas de las familias Lauraceae y Atherospermataceae. Rev. Cubana Plantas Med.. 2012;17:73-83.
- [Google Scholar]
- Explaining the antioxidant activity of some common non-phenolic components of essential oils. Food Chem.. 2017;232
- [CrossRef] [Google Scholar]
- Fungistatic Activity Of Essential Oils Extracted from Peumus boldus Mol., Laureliopsis philippiana (Looser) Schodde and Laurelia sempervirens (Ruiz & Pav.) Tul. (Chilean Monimiaceae) Chilean J. Agric. Res.. 2009;69:30-37.
- [Google Scholar]
- Effects of essential oils from five plant species against the granary weevils sitophilus zeamais and acanthoscelides obtectus (coleóptera) J. Chil. Chem. Soc.. 2008;53:1444-1448.
- [Google Scholar]
- Natural antioxidants: sources, compounds, mechanisms of action, and potential applications. Compr. Rev. Food Sci. Food Saf.. 2011;10:221-247.
- [CrossRef] [Google Scholar]
- Repelencia de Mezclas de Aceites Esenciales de Boldo, Laurel Chileno, y Tepa Contra el Gorgojo del Maíz. Southwestern Entomol.. 2017;42:551-562.
- [CrossRef] [Google Scholar]
- Synergy and antagonism in natural product extracts: when 1 + 1 does not equal 2. Nat. Prod. Rep.. 2019;36:869-888.
- [CrossRef] [Google Scholar]
- CLSI, 2015. Methods for dilution antimicrobial susceptibility tests for bacteria that grow aerobically; approved standard. CLSI document M07-A10. C. a. L. S. Institute. Clinical and Laboratory Standards Institute, Wayne, PA.
- Nonlocal (Pair Site) reactivity from second-order static density response function: gas- and solution-phase reactivity of the acetaldehyde enolate as a test case. J. Phys. Chem. A. 1999;103:1367-1375.
- [CrossRef] [Google Scholar]
- De Abreu Costa, L., M. Henrique Fernandes Ottoni, M. G. Dos Santos, et al., 2017. Dimethyl Sulfoxide (DMSO) Decreases Cell Proliferation and TNF-α, IFN-γ, and IL-2 Cytokines Production in Cultures of Peripheral Blood Lymphocytes. Molecules. 22, 1789.
- An overview of the biological effects of some mediterranean essential oils on human health. Biomed Res. Int.. 2017;2017:9268468.
- [CrossRef] [Google Scholar]
- Frisch, M. J., G. W. Trucks, H. B. Schlegel, et al., 2016. Gaussian 16 Rev. C.01. Wallingford, CT.
- Role of frontier orbitals in chemical reactions. Science. 1982;218:747-754.
- [CrossRef] [Google Scholar]
- The influence of environmentally friendly pesticide - Eucalyptol - alone and in combination with terpinen-4-ol - on model bacterial membranes. Colloids Surf. B Biointerfaces. 2016;146:918-923.
- [CrossRef] [Google Scholar]
- Bioactivity of Peumus boldus Molina, Laurelia sempervirens (Ruiz & Pav.) Tul. and Laureliopsis philippiana (Looser) Schodde (Monimiacea) essential oils against Sitophilus zeamais Motschulsky. Chilean J. Agric. Res.. 2015;75:334-340.
- [Google Scholar]
- Assessment of antioxidative, chelating, and DNA-protective effects of selected essential oil components (eugenol, carvacrol, thymol, borneol, eucalyptol) of plants and intact Rosmarinus officinalis oil. J. Agric. Food Chem.. 2014;62:6632-6639.
- [CrossRef] [Google Scholar]
- The chemistry behind antioxidant capacity assays. J. Agric. Food Chem.. 2005;53:1841-1856.
- [CrossRef] [Google Scholar]
- Thymus vulgaris essential oil and thymol inhibit biofilms and interact synergistically with antifungal drugs against drug resistant strains of Candida albicans and Candida tropicalis. J. Mycol. Med.. 2020;30:100911
- [CrossRef] [Google Scholar]
- Review of methods to determine antioxidant capacities. Food Anal. Methods. 2009;2:41-60.
- [CrossRef] [Google Scholar]
- Larrazabal-Fuentes, M., J. Palma, A. Paredes, et al., 2019. Chemical composition, antioxidant capacity, toxicity and antibacterial activity of the essential oils from Acantholippia deserticola (Phil.) Moldenke (Rica rica) and Artemisia copa Phil. (Copa copa) extracted by microwave-assisted hydrodistillation. Industrial Crops and Products. 142, 111830. https://doi.org/10.1016/j.indcrop.2019.111830.
- Synergy among thymol, eugenol, berberine, cinnamaldehyde and streptomycin against planktonic and biofilm-associated food-borne pathogens. Lett. Appl. Microbiol.. 2015;60:421-430.
- [CrossRef] [Google Scholar]
- Chemical characterization and anti-oomycete activity of Laureliopsis philippianna essential oils against Saprolegnia parasitica and S. australis. Molecules. 2015;20:8033-8047.
- [CrossRef] [Google Scholar]
- Chemoprevention and therapeutic role of essential oils and phenolic compounds: modeling tumor microenvironment in glioblastoma. Pharmacol. Res.. 2021;169:105638
- [CrossRef] [Google Scholar]
- Antimicrobial activity of six essential oils against a group of human pathogens: a comparative study. Pathogens (Basel, Switzerland). 2019;8:15.
- [CrossRef] [Google Scholar]
- Inhibitory effects of the essential oils α-longipinene and linalool on biofilm formation and hyphal growth of Candida albicans. Biofouling. 2017;33:143-155.
- [CrossRef] [Google Scholar]
- Bio-evaluation of the wound healing activity of Artemisia judaica L. as part of the plant's use in traditional medicine; phytochemical, antioxidant, anti-inflammatory, and antibiofilm properties of the plant's essential oils. Antioxidants (Basel). 2022;11
- [Google Scholar]
- Ethnobotanical review of the Mapuche medicinal flora: use patterns on a regional scale. J. Ethnopharmacol.. 2009;122:251-260.
- [CrossRef] [Google Scholar]
- Antimicrobial evaluation of Huilliche plant medicine used to treat wounds. J. Ethnopharmacol.. 2011;138:219-227.
- [CrossRef] [Google Scholar]
- Study on the cytotoxic activity of Drimane Sesquiterpenes and Nordrimane compounds against cancer cell lines. Molecules. 2014;19:18993-19006.
- [Google Scholar]
- Antioxidant assays for plant and food components. J. Agric. Food. Chem.. 2009;57:1655-1666.
- [CrossRef] [Google Scholar]
- Essential oil variation within and among natural populations of Lavandula latifolia and its relation to their ecological areas. Biochem. Syst. Ecol.. 2007;35:479-488.
- [CrossRef] [Google Scholar]
- Assessment of antioxidant capacity in vitro and in vivo. Free Radic. Biol. Med.. 2010;49:503-515.
- [CrossRef] [Google Scholar]
- Ortiz U, A., G. Silva A, A. Urbina P, et al., 2012. Bioactivity of Tepa (Laureliopsis philippiana (Looser) Shodde) Powder to Sitophilus zeamais Motschulsky Control in Laboratory. Chilean journal of agricultural research. 72, 68-73.
- Density functional approach to the frontier-electron theory of chemical reactivity. J. Am. Chem. Soc.. 1984;106:4049-4050.
- [CrossRef] [Google Scholar]
- Evaluation of antioxidants: Scope, limitations and relevance of assays. Chem. Phys. Lipids. 2012;165:638-647.
- [CrossRef] [Google Scholar]
- Variations in the consumption of antimicrobial medicines in the European Region, 2014–2018: findings and implications from ESAC-Net and WHO Europe. Front. Pharmacol.. 2021;12
- [Google Scholar]
- Antigrowth activity and induction of apoptosis in human melanoma cells by Drymis winteri forst extract and its active components. Chem. Biol. Interact.. 2019;305:79-85.
- [CrossRef] [Google Scholar]
- Essential oil analysis and phytotoxic activity of catnip (Nepeta cataria L.) Am. J. Essential Oils Nat. Prod.. 2016;4:40-45.
- [Google Scholar]
- Comparative metabolic profiling of essential oils from Spondias pinnata (Linn. F.) Kurz and characterization of their antibacterial activities. Ind. Crops Prod.. 2019;137:468-474.
- [CrossRef] [Google Scholar]
- ROS function in redox signaling and oxidative stress. Curr. Biol.. 2014;24:R453-R462.
- [CrossRef] [Google Scholar]
- Media and environmental effects on phenolics production from tobacco cell cultures. Biotechnol. Bioeng.. 1989;33:1437-1444.
- [CrossRef] [Google Scholar]
- Biological activities of essential oils: from plant chemoecology to traditional healing systems. Molecules (Basel, Switzerland). 2017;22:70.
- [CrossRef] [Google Scholar]
- Essential oils as anticancer agents: Potential role in malignancies, drug delivery mechanisms, and immune system enhancement. Biomed. Pharmacother.. 2022;146:112514
- [CrossRef] [Google Scholar]
- Effects of geldanamycin on hatching and juvenile motility in Caenorhabditis elegans and Heterodera glycines. J. Chem. Ecol.. 2005;31:2481-2491.
- [CrossRef] [Google Scholar]
- Calli essential oils synergize with lawsone against multidrug resistant pathogens. Molecules. 2017;22:2223.
- [CrossRef] [Google Scholar]
- New bisbenzylisoquinoline alkaloid from Laureliopsis philippiana. Fitoterapia. 2009;80:112-114.
- [CrossRef] [Google Scholar]
- Bisbenzylisoquinoline alkaloids as markers of Atherospermataceae: tetrandrine and fangchinoline from Laureliopsis philippiana. Biochem. Syst. Ecol.. 2010;38:450-453.
- [Google Scholar]
- Optimization of parameters for semiempirical methods V: modification of NDDO approximations and application to 70 elements. J. Mol. Model.. 2007;13:1173-1213.
- [CrossRef] [Google Scholar]
- Stewart, J. J., 2007. Stewart computational chemistry. http://openmopac. net/.
- Iron (FeII) chelation, ferric reducing antioxidant power, and immune modulating potential of Arisaema jacquemontii (Himalayan Cobra Lily) Biomed Res. Int.. 2014;2014
- [CrossRef] [Google Scholar]
- Antimicrobial activity of oligomer and polymer chitosan from different sources against foodborne pathogenic bacteria. Kasetsart J. – Nat. Sci.. 2011;45:636-643.
- [Google Scholar]
- Teles Fujishima, M. A., N. D. S. R. d. Silva, R. D. S. Ramos, et al., 2018. An Antioxidant Potential, Quantum-Chemical and Molecular Docking Study of the Major Chemical Constituents Present in the Leaves of Curatella americana Linn. Pharmaceuticals. 11, 72.
- Chemical composition and antibacterial activity of Laureliopsis philippiana (Looser) essential oil. Bol. Latinoam. Caribe Plant. Med. Aromat.. 2014;13:117-125.
- [Google Scholar]
- Propiedades insecticidas del polvo de Laurelia sempervirens L. para el control de Sitophilus zeamais Motschulsky (Coleoptera: Curculionidae) Bol. Latinoam. Caribe Plantas Med. Aromát.. 2015;14:48-59.
- [Google Scholar]
- The chemical compositions of essential oils derived from cryptocarya alba and Laurelia sempervirens possess antioxidant, antibacterial and antitumoral activity potential. Molecules. 2020;25:5600.
- [Google Scholar]
- Oxidative stress in the aging substantia nigra and the etiology of Parkinson's disease. Aging Cell. 2019;18:e13031
- [CrossRef] [Google Scholar]
- The phenolic hydroxyl group of carvacrol is essential for action against the food-borne pathogen Bacillus cereus. Appl. Environ. Microbiol.. 2002;68:1561-1568.
- [CrossRef] [Google Scholar]
- Methods for dilution antimicrobial susceptibility tests for bacteria that grow aerobically. Nat. Committee Clin. Lab. Standards 1990
- [Google Scholar]
- Oxidative stress in metabolic disorders: pathogenesis, prevention, and therapeutics. Oxid. Med. Cell. Longevity. 2016;2016:9137629.
- [CrossRef] [Google Scholar]
- Repellency and toxicity of essential oils from the leaves and bark of Laurelia sempervirens and Drimys winteri against Tribolium castaneum. Ind. Crops Prod.. 2010;32:405-410.
- [CrossRef] [Google Scholar]
Appendix A
Supplementary material
Supplementary data to this article can be found online at https://doi.org/10.1016/j.arabjc.2022.104271.
Appendix A
Supplementary material
The following are the Supplementary data to this article: