Translate this page into:
In vitro and in silico evaluation of the antimicrobial and antioxidant activities of spiropyrazoline oxindole congeners
⁎Corresponding authors. maboulsoud@ksu.edu.sa (Mourad A.M. Aboul-Soud), elyazidimohamed@hotmail.com (Mohamed El Yazidi)
-
Received: ,
Accepted: ,
This article was originally published by Elsevier and was migrated to Scientific Scholar after the change of Publisher.
Abstract
Abstract
The in vitro antimicrobial and antioxidant activities of spiropyrazoline oxindole congeners were carried out. Some spiro-compounds represent interesting antimicrobial and antioxidant activities. The in vitro activities were supported by in-silico studies using DFT method, Molecular Docking, Molecular Dynamics Simulation and POM analysis. A good correlation between the experimental results and in-silico studies was observed. POM analyses show that all compounds exhibited good bioavailability, pharmacokinetic, and no toxicity profiles.
Abstract
The search for novel powerful antimicrobial and antioxidant agents is considered a dynamic field in medicinal chemistry. In this context, a series of spiropyrazoline indolin-3-one congeners were assessed for their in vitro bioactivities, and in-silico studies were conducted to support the experimental results. The antimicrobial screening of the spiropyrazoline oxindole congeners against the selected microbe strains (Staphylococcus aureus (CECT 976), Bacillus subtilis (DSM 6633), Escherichia coli (K12), and Candida albicans (ATCC 10231)) exhibited moderate to excellent, compared to control standard antibiotics (Ampicillin, streptomycin and fluconazole). This activity was observed to be tightly dependent upon the nature of the substituents carried by the aromatic rings. Moreover, the tested compounds showed variable dose-dependent antioxidant activity. Notably, congeners 2c, 2d and 2e exhibited a remarkable antioxidant activity, due to the positive impact of the electron-donating groups (CH3 and OCH3) on the antioxidant activity. Density functional theory (DFT) simulations were executed on the target molecules to better understand their structural and electronic properties, as well as to explain the results obtained from the antioxidant activity. The molecular docking studies showed that the studied congeners have good binding affinities and interactions with the target proteins (catalase compound II and CYP51). Moreover, the 100 ns molecular dynamics (MD) simulation analysis was conducted to follow the behavior of the complexes formed between ligand 2e and the target proteins (2CAG and 5V5Z) under in-silico physiological conditions to explore and evaluate its stability over time. MD simulation indicated a stable conformation and binding patterns in a stimulating environment of the congeners (2CAG-2e and 5V5Z-2e). The results of Petra/Osiris/Molinspiration (POM) analyses suggested that all the spiranic cycloadducts have good oral bioavailability and pharmacokinetics without any evidence of observed toxicity. Taken together, our findings provide valuable experimental and theoretical information that will be helpful for designing novel spiranic molecules with potential pharmacological applications.
Keywords
Spiropyrazoline oxindoles
Antimicrobial property
Antioxidant potency
Molecular docking
MD simulations
POM analyses
1 Introduction
Bacteria and yeasts are responsible for many pathogenic microbial infections that pose a threat to the health of animals and humans, potentially leading to a serious global crises (de Lastours et al., 2020; Riley, 2014). The fight against bacterial and fungal infections is a serious and permanent problem for the world scientific community, due to the resistance developed by microorganisms following the modifications of their genomes via random mutations (Aragon-Martinez et al., 2017). This developed multi-drug resistance leads to major risk and a higher mortality and morbidity rate, which requires more effective alternative therapies and drugs with new and different modes of action (Ali et al., 2018; de Lastours et al., 2020). Moreover, oxidative stress (OS) induced by the excessive production of free radicals during cellular metabolism leads to oxidative cellular damage to biological macromolecules (Ray et al., 2012; Valko et al., 2007). Currently, OS is considered the main cause of the occurrence and development of various pathologies such as cardiovascular diseases, cancer, diabetes mellitus, inflammation, and neurodegenerative diseases (Aragon-Martinez et al., 2017). To combat these diseases, it is imperative to design and develop antioxidants that are capable of slowing down the formation of free radicals and thereby reducing oxidative damage.
Synthesis of novel compounds as well as the chemical and structural modification of readily available bioactive molecules are amongst the strategies of choice for discovering promising drug candidates (Barbier et al., 2022). Heterocyclic compounds are widely studied for their biological activities (Barbier et al., 2022; Chalkha et al., 2022a; Khattab and Al-Karmalawy, 2021; Malik et al., 2021). Many heterocyclic molecules are already used as pharmacophores in several drugs to treat various diseases. They remain one of the main sources of new drug molecules. Spiro-heterocyclic compounds form an important class of heterocycles that have received considerable attention owing to their presence in bioactive, natural and synthetic products (Chupakhin et al., 2019; Zheng et al., 2014). These heterocycles are endowed with a characteristic conformational rigidity and three-dimensional geometry allowing them to interact strongly with biological targets (Zheng et al., 2014). In particular, spiro-indolinones, also referred to as spiro-oxindoles, are among the most privileged spiranic structures in the field of medicinal chemistry (Al-Majid et al., 2020; Altowyan et al., 2019; Boddy and Bull, 2021; Zhou et al., 2020). They are predominant in the basic skeleton of several natural alkaloids characterized by their pronounced pharmaceutical properties such as Fluorocurine, Mitragynine, Rauniticine, Mitraphylline, Horsfiline and Rhynchophylline (Boddy and Bull, 2021; Chupakhin et al., 2019; Váradi et al., 2016). On the other hand, spiropyrazolines form another class of spiro-compounds present in the skeleton of many synthetic molecules with various pharmacological applications such as antimicrobial, antioxidant and anticancer (Farghaly et al., 2020; Sadiq et al., 2019). Spirocyclic compounds formed by indolin-2-one and pyrazoline nuclei have shown remarkable bioactivity against cancer cell lines and pathogenic microorganisms (Monteiro et al., 2014; Raposo et al., 2021; Yang et al., 2015). However, spiropyrazoline indolin-3-ones are not sufficiently studied and exploited in the field of medicinal chemistry. This prompted us to prepare spiropyrazoline indolin-3-ones and evaluate their biological activity.
Recently, cheminformatics techniques have become widely employed in the field of medicinal chemistry (Bakchi et al., 2022). These approaches help medicinal chemists in identifying and selecting new molecules likely to become drug candidates and also allow them to justify the experimental data obtained (Chalkha et al., 2022a; Daoui et al., 2021; Ibrar et al., 2018). POM analysis (Petra/Osiris/Molinspiration) is among the in-silico tools used to predict the physicochemical and pharmacokinetic properties of small molecules (Ben Hadda et al., 2014; Chalkha et al., 2022b). Molecular docking studies make it possible to understand the modes and ligand-receptor affinity energies (Chalkha et al., 2022a, 2022b; Daoui et al., 2021; Kavaliauskas et al., 2020). In addition, advances in technology and breakthroughs have greatly improved the capabilities of both programs and computers used for theoretical calculations, making it easier to compare the activities of molecules through computing chemical parameters (Lakhrissi et al., 2022; Rbaa et al., 2022). Among the computational methods, the DFT approach is a powerful tool that offers significant information on the chemical, electronic and physical characteristics of molecules, allowing a better understanding of their biological activities (Khattab and Al‐Karmalawy, 2021; Vásquez-Espinal et al., 2019).
Encouraged by the potential pharmacological properties of spiro-compounds, and as an extension of our research focused on the synthesis of novel aza-heterocyclic compounds for therapeutic purposes (Chalkha et al., 2023, Chalkha et al., 2022a, Chalkha et al., 2022b, Chalkha et al., 2022c, 2022d). The aim of this study is to evaluate a series of spiropyrazoline indolin-3-one congeners for their in vitro antioxidant capacity and antimicrobial activities against four pathogenic microorganisms. Moreover, DFT calculations, POM analysis, docking studies and MD simulations were conducted to explain the experimental results of the biological activity.
2 Experimental section
2.1 Chemistry
The used reagents and solvents, the synthesis procedure of the spiropyrazoline oxindole congeners, and the different spectroscopic techniques used in their characterization are available in our previously published work (Chalkha et al., 2020).
2.2 Antioxidant activity
2.2.1 DPPH assay
To evaluate the antioxidant capacity of the target spiropyrazoline indolinones, we used the DPPH assay adopting the protocols described in the literature (Chalkha et al., 2022b, 2022c; El Moussaoui et al., 2021, 2019). A fresh methanolic solution of DPPH (0.004 %) was prepared and conserved at room temperature in the darkness. Different concentrations of the studied compounds and butylated hydroxytoluene (BHT: positive control) were prepared and selected for a good comparison (62.5, 125, 250, 500, and 1000 μg/mL). Then, 100 µL of various concentrations were combined with 750 µL of a methanolic solution of DPPH and stirred. After 30 min of incubation in a dark room, the discoloration was registered at 517 nm. The absorbance of DPPH solution without sample was used as a control and methanol 95 % used was as blank. The absorbances of the reaction mixture recorded with a UV–Visible spectrophotometer (Jasco V-530) were compared to those of the control and blank. Antioxidant activity was measured and expressed as percent inhibition (%) of DPPH utilizing described method (Chalkha et al., 2022b, 2022c; El Moussaoui et al., 2021; El Moussaoui et al., 2019).
2.2.2 Reducing power capacity (FRAP)
The reducing antioxidant capacity of the synthesized spiropyrazolines was determined by following the procedures previously published in the literature with slight modifications (El Moussaoui et al., 2021; Tan et al., 2022). The target spiro-compounds 2(a-i) were tested at various concentrations and the absorbances of the reaction mixture were measured at 700 nm using a UV spectrophotometer. The antioxidant BHT was used as a standard. The average absorbance of each compound is calculated after three tests. The ferric reducing antioxidant power is indicated by observing the absorbance of the tested compounds at 700 nm.
2.3 Antimicrobial activity
2.3.1 Antimicrobial screening
The antimicrobial properties of the target spiropyrazoline oxindole congeners 2(a-i) against Staphylococcus aureus (CECT 976), Bacillus subtilis (DSM 6633), Escherichia coli (K12), and Candida albicans (ATCC 10231) were explored using the standard agar disk diffusion technique, following the protocols outlined in the literature with some modifications (Barmak et al., 2019; Chebbac et al., 2022; Esfahani et al., 2021; Ravindar et al., 2018). Stock solutions of the spiro-congeners 2(a-i) were prepared in 1 mL of N,N-Dimethylformamide (DMF) to obtain the necessary concentrations (5, 2.5 and 1.25 mg/mL). Then, 10 μL of the spiranic molecules 2(a-i) at various concentrations were then added to the Whatman Filter Paper disk (6 mm diameter) and dried for 10 min. Ampicillin and streptomycin were used as standard antibacterial agents, while Fluconazole was used as a standard agent for antifungal activity. DMF alone was used as a control, and it showed no effect on the growth of microorganisms. Then, the plates were incubated at 37 °C for 24 h for the bacteria and at 30 °C for 48 h for the yeasts. Finally, the average zone of inhibition around the disk was measured using a transparent ruler (in mm) and the MIC values were recorded as the lowest concentration of antimicrobial agent significantly inhibiting the growth of the tested microbes. The percentage activity index for the tested congeners 2(a-i) was estimated using the equation shown below (Abu-Dief et al., 2020; Shehab et al., 2022):
2.3.2 Minimum inhibitory concentrations (MICs) measurement
The MIC values of the spiro-compounds were determined using the microdilution susceptibility test following a slightly modified method described in literature (Alici et al., 2022; Bishoyi et al., 2021). The serial dilutions were prepared from a stock DMF solution of 5 mg/mL of the investigated compounds. The details of the preparation of the used culture media, the dilutions of the tested spiropyrazoline oxindoles solutions, and the incubation were described in our previously published work (Chalkha et al., 2022a, 2022b, 2022c). The MIC value is recorded as the lowest concentration of the synthesized congeners that prevents the growth of the tested microorganisms. The experiments were repeated twice, and the average results were calculated for possible antimicrobial activity.
2.4 Theoretical calculations
The used programs are Gaussian 09 RevD.01 (Frisch et al., 2009), and GaussView 6.0(R. Dennington et al., 2016). By using these programs, the simulations were made in B3LYP, HF and M06-2X (Becke, 1992; Hohenstein et al., 2008; Vautherin and Brink, 1972) methods with the 6–31++g(d,p) basis set (Csonka, 2002; Turi and Dannenberg, 1993). The SCF procedure is used default settings. Numerous quantum chemical descriptors can be calculated in these basis sets. These computed parameters are given in Table 4 (Rezaeivala et al., 2022b).
The calculation of multiple thermodynamic parameters is necessary to theoretically compare the antioxidant activity of different compounds. The following thermodynamic variables are involved: bond dissociation enthalpy (BDE), proton dissociation enthalpy (PDE), proton affinity (PA), ionization potential (IP), and electron transfer enthalpy (ETE) (Koç et al., 2019; Marković et al., 2015; Tüzün, 2020; Urbaniak et al., 2013). The anti-oxidant investigation in this study looked at three mechanisms. The process for moving hydrogen atoms is the first (HAT).
According to this mechanism, the anti-oxidant (AH) is transformed into its free radical form (
) by the free radical molecule (
), which takes a hydrogen atom from it. Calculating the bond dissociation enthalpy (BDE) of the A-H bond is important to determine the compound's reactivity. Single electron transport and proton transfer make up the second mechanism (SET–PT). There are two successive responses in this process. In the first, the proton transfer is followed immediately by the removal of the electron from the AH.
The proton affinity (PA) of the anion is the reaction enthalpy of the first reaction. The second reaction's reaction enthalpy is that of abstraction of electrons, or electron transfer enthalpy (ETE).
2.5 Molecular docking
Molecular docking studies were made by Autodock Vina software (Trott and Olson, 2010), to explain the experimental results by investigating the interaction modes of the Spiropyrazoline indolinone derivatives with target proteins involved in antimicrobial and antioxidant activities. The crystal structure of Catalase compound II (2CAG) as a protein target for antioxidant compounds (Gouet et al., 1996), was downloaded from the RCSB Protein Data Bank available online at (https://www.RCSB.org/structure/2CAG). The crystal structure of CYP51 isolated and characterized by Mikhail V. Keniya (Keniya et al., 2018) as important protein for Candida Albicans cell growth (Masood et al., 2018), was obtained from the RCSB Protein Data Bank (PDB ID: 5V5Z). To prepare the target proteins (catalase compound II and CYP51) for docking, we applied the following procedure via AutoDockTools (ADT / AutoDockTools — AutoDock, 2021 https://autodock.scripps.edu/resources/adt (accessed Sep. 18, 2021).,” n.d.):
-
Step 1: Water molecules and ligands were deleted from the protein structures.
-
Step 2: Kollman charges and polar hydrogen were added.
-
Step 3: A docking gird box (coordinates: x = 61.290, y = 13.865, z = 13.906 at 40 Å size and 0.375 Å spacing) was generated to cover the active site of catalase compound II during docking. Besides, x = -40.656, y = -10.618, z = 25.111 were set as the docking center for ligands targeting the CYP51 protein.
-
Step 4: PDBQT files corresponding to the prepared structures were generated.
Besides, the ligand structures were optimized by applying the MMFF94 force field (Halgren, 1996) along with the steepest Descent method (5000 steps) available in Avogadro software (“‘Avogadro - Free cross-platform molecular editor - Avogadro’ https://avogadro.cc/ (accessed Mar. 12, 2022).,” n.d.; Hanwell et al., 2012). Thereafter performing the optimization, AutoDockTools was used to add Gasteiger charges, merge polar hydrogen and generate the PDBQT files corresponding to the prepared ligands. Finally, the prepared ligands were docked to the protein target and their interactions were evaluated through Discovery Studio 2021 software (Free Download: BIOVIA Discovery Studio Visualizer - Dassault Systèmes, 2021 https://discover.3ds.com/discovery-studio-visualizer-download (accessed Jun. 13, 2021).,” n.d.).
2.6 Molecular dynamics simulations (MD)
In order to assess the stability of the ligand–protein complexes, MD simulations were carried out employing GROMACS (Abraham et al., 2015) with the charmm27 force field (Lindahl et al., 2010). The SwissParam serve was employed to generate the ligand topologies (Zoete et al., 2012). Moreover, a cubic simulation box was used to cover the ligand–protein complexes which were then solvated with TIP3P water molecules. To make the overall system more neutral, sodium and chloride ions have been introduced. The steepest descent minimization method was thereafter employed to optimize the all-simulated systems in order to prevent steric crashes. Next, an NVT equilibration at 300 K for 1 ns, followed by an NPT equilibration employing the Parrinello-Rahman barostat at 1 atm for 1 ns (Parrinello and Rahman, 1981), was conducted for the purpose of stabilizing the systems at the designed conditions. The equilibrated systems were subsequently subjected to a 100,000 ps MD simulation, and a variety of parameters, including RMSD, RMSF, RoG, number of hydrogen bonds, and SASA, were computed from the simulation findings.
2.7 POM Theory and identification of pharmacophore sites
The POM Theory (Petra/Osiris/Molinspiration) developed by Taibi Ben Hadda group in collaboration with the American NCI and TAACF lead to considerable progress in the areas of pharmacology and drug design (Esharkawy et al., 2022). The most significant and critical descriptions in the POM Theory are:
-
The geometry of pharmacophore sites (cis or trans).
-
Type of 3D complementary interaction of drugs with specific bio targets.
-
The electrostatic drug/bio target interaction.
By using the POM Theory, it is now possible to discover and identify most of the antibacterial (Bhat et al., 2021), antifungal (Titi et al., 2020), antiviral (Ben Hadda et al., 2021), antiparasitic (Alawadi et al., 2014), and antitumor (Berredjem et al., 2022) pharmacophore sites. These sites can be identified one by one, based on their various physico-chemical proprieties and corresponding heteroatom electronic charge distributions. This young POM Theory has been successfully applied to a variety of diverse bio targets (Lahsasni et al., 2015).
3 Results and discussion
3.1 Synthesis of spiropyrazoline oxindoles
The spiropyrazoline oxindole congeners have been prepared following the synthetic procedure as outlined in scheme 1. The 2-arylideneindolin-3(2H)-ones 1(a-e) known as aza-aurones are prepared from anthranilic acid according to the procedures reported in our previous work (Chalkha et al., 2020). The reaction of α-chloroarylidene-phenyl-hydrazones precursors with aza-aurones 1(a-e) under the action of triethylamine in chloroform led to the formation of a series of spiropyrazoline indolin-3-ones 2(a-i) (Chalkha et al., 2020). The reaction leads to the formation of a single spiranic cycloadduct in high yields, and the structure of the synthesized cycloadducts were established by the usual spectroscopic methods (Chalkha et al., 2020).Synthesis route of spiropyrazoline indolin-3-ones 2(a-i).
3.2 Biological assessments
3.2.1 Antioxidant activity
-
DPPH assay
The results of the DPPH radical scavenging activity of the antioxidant standard BHT (butylated hydroxytoluene) and target spirocongeners at different concentrations are expressed as an average and summarized in Table 1.
% Radical scavenging activity (mean ± S.D.)*
Compounds
Concentrations in µg/mL
N°
R
R1
62.5
125
250
500
1000
2a
–
–
43.28 ± 1.25
46.71 ± 0.68
47.03 ± 0.81
54.92 ± 1.37
61.25 ± 0.44
2b
–
Cl
43.98 ± 1.43
44.45 ± 1.08
45.15 ± 0.78
45.85 ± 1.45
47.57 ± 1.74
2c
OCH3
–
55.54 ± 0.68
57.54 ± 0.97
60.26 ± 1.04
63.98 ± 1.40
81.12 ± 1.71
2d
OCH3
Cl
47.18 ± 0.75
51.42 ± 1.17
54.14 ± 0.77
59.84 ± 1.02
74.84 ± 0.61
2e
CH3
–
48.39 ± 0.62
50.73 ± 0.64
52.76 ± 0.72
54.61 ± 1.64
80.63 ± 1.42
2f
Cl
–
38.98 ± 0.43
42.73 ± 0.67
48.75 ± 0.42
49.61 ± 1.03
52.89 ± 0.67
2 g
Cl
Cl
31.01 ± 0.56
32.29 ± 0.92
34.76 ± 0.81
40.23 ± 0.94
54.85 ± 1.47
2 h
Br
–
28.04 ± 0.68
29.76 ± 0.84
46.79 ± 0.63
65.39 ± 1.32
76.52 ± 0.93
2i
Br
Cl
46.01 ± 0.56
46.71 ± 0.34
47.11 ± 1.31
48.13 ± 1.24
50.16 ± 0.64
BHT
–
–
65.66 ± 1.03
74.91 ± 1.35
81.33 ± 1.49
84.13 ± 0.98
89.30 ± 1.95
The results of the preliminary screening revealed that the tested spiro-compounds exhibit variable antioxidant activity against DPPH radicals. Indeed, the free radical (DPPH) scavenging capacity increased as a function of increasing concentrations of the tested spiropyrazoline oxindole congeners, with values approaching that of the standard at high concentrations for some of the tested congeners (Fig. 1). The observed difference in the antioxidant capacity among various congeners studied can be attributed to the effect and nature of the substituents carried by the phenyls (Ali et al., 2020). Among the tested products, compounds 2c (R = OCH3, R1 = H), 2d (R = OCH3, R1 = Cl), and 2e (R = CH3, R1 = H) exhibited remarkable antioxidant activity and disclosed higher inhibition, with IC50 values of 54.13 ± 1.86, 104.25 ± 3.91, and 119.07 ± 2.84 μg/mL, respectively (Fig. 1), which is likely due to the existence of the electron-donating methyl and methoxy substituents (Kumara et al., 2021). Moreover, for compound 2d (R = OCH3, R1 = Cl), it was observed that the introduction of a chlorine atom at the substituent R1 resulted in a slight decrease in the antiradical activity compared to compound 2c (R = OCH3, R1 = H). The same trend was observed for derivatives of the series 2a (R = H, R1 = H) and 2b (R = H, R1 = Cl). This obtained result further highlights the negative influence of electron-withdrawing substituents on the free radical scavenging ability. The rest of the congeners exhibited weak scavenging potential compared to the standard antioxidant, except for compound 2 h (R = Br, R1 = H) that exhibited significant antioxidant activity at high concentrations (Table 1).
-
FRAP assay
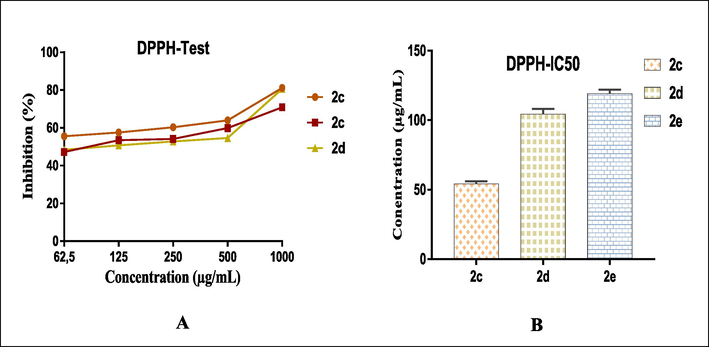
- Percentage of free radical scavenging DPPH according to the different concentrations used of spiro-congeners 3c, 3d and 3e (A) and IC50 values for the three compounds (B).
The reducing power of the spiranic compounds 2(a-i) was assessed by the FRAP (Ferric reduction antioxidant power) test using butylated hydroxytoluene (BHT) as the standard antioxidant. This test was used to assess the capability of the target spiro-compounds 2(a-i) to reduce the ferric ion (Fe3+) existing in the K3Fe(CN)6 complex to ferrous ion (Fe2+). The obtained results revealed that all the tested spiropyrazoline oxindole congeners possessed a concentration-dependent capacity to reduce Fe3+ (Table 2). This observation is consistent with that obtained in the DPPH assay (Table 1). Among the tested congeners, 2c (EC50 = 574.52 ± 3.68 μg/mL), 2d (EC50 = 737.66 ± 5.7 μg/mL), and 2e (EC50 = 841.39 ± 7.46 μg/mL), bearing electron-donating substituents in the aromatic rings, exhibited remarkable ferric reducing power compared to the positive control BHT at the maximum tested concentration of 1 mg/mL (Fig. 2). The same result of reductive capability is achieved with the non-substituted compound 2a. However, the other compounds with electron-withdrawing substituents exhibited a lower reducing power.
Mean Absorbance ± (S.D.) * at Different Concentrations
Compounds
Concentrations in µg/mL
N°
R
R1
125
250
500
1000
2a
–
–
0.146 ± 0.003
0.204 ± 0.012
0.298 ± 0.018
0.647 ± 0.022
2b
–
Cl
0.119 ± 0.018
0.160 ± 0.006
0.274 ± 0.023
0.406 ± 0.045
2c
OCH3
–
0.157 ± 0.013
0.284 ± 0.014
0.472 ± 0.033
0.781 ± 0.063
2d
OCH3
Cl
0.143 ± 0.033
0.197 ± 0.033
0.314 ± 0.009
0.683 ± 0.032
2e
CH3
–
0.138 ± 0.008
0.175 ± 0.012
0.308 ± 0.023
0.579 ± 0.075
2f
Cl
–
0.120 ± 0.027
0.152 ± 0.007
0.261 ± 0.010
0.382 ± 0.011
2 g
Cl
Cl
0.117 ± 0.015
0.160 ± 0.011
0.251 ± 0.055
0.302 ± 0.043
2 h
Br
–
0.124 ± 0.045
0.169 ± 0.044
0.298 ± 0.062
0.493 ± 0.042
2i
Br
Cl
0.114 ± 0.036
0.192 ± 0.032
0.301 ± 0.036
0.396 ± 0.071
BHT
–
–
0.203 ± 0.017
0.323 ± 0.026
0.702 ± 0.056
1.161 ± 0.016
The antioxidant reducing capacity in function of the different concentrations used of spiro-congeners 3c, 3d and 3e (A), EC50 values of the selected compounds (B).
3.2.2 Antimicrobial activity
The in vitro antimicrobial activity of the synthetized spiropyrazoline oxindole congeners 2(a-i) was assessed against four pathogenic microbes, using the standard agar diffusion method as reported previously with a minor modification (Esfahani et al., 2021; Ravindar et al., 2018). Streptomycin and Ampicillin were used as positive controls for antibacterial activity, whereas Fluconazole for antifungal activity. Preliminary results of the antimicrobial screening of spiropyrazoline oxindole derivatives 2(a-i) indicating the lowest dose that totally inhibits microorganism growth for each congener and the measured diameter of the inhibition zone for this concentration are presented in Table 3. NZ = No zone of inhibition; diameter of filter disk is 6 mm; NT: No tested; NE: This compound has No Effect or Negligible Effect on this strain; AI: Activity Index,
zone of inhibition in mm (mg/mL) *
Escherichia coli
AI (%)
Bacillus subtilis
AI (%)
Candida albicans
AI (%)
Staphylococcus aureus
AI (%)
N°
R
R1
2a
–
–
12.5 (2.25)
52
10 (5)
62
15 (1.25)
71
11 (1.25)
47
2b
–
Cl
12 (1.25)
50
7 (1.25)
43
NE
---
9 (1.25)
39
2c
OCH3
–
NE
---
NE
---
10 (1.25)
47
9 (2.25)
39
2d
OCH3
Cl
23 (1.25)
95
7.5 (1.25)
46
10.5 (2.25)
50
10.2 (1.25)
44
2e
CH3
–
NE
---
13 (1.25)
81
15.3 (1.25)
72
10 (5)
43
2f
Cl
–
7 (1.25)
29
NE
---
13 (1.25)
61
11 (2.25)
47
2 g
Cl
Cl
NE
---
14 (1.25)
87
NE
7 (1.25)
30
2 h
Br
–
10 (2.25)
41
NE
---
16 (1.25)
76
10 (2.25)
43
2i
Br
Cl
21 (1.25)
87
11 (1.25)
68
13 (1.25)
61
12 (1.25)
52
Ampicillin
NT
---
16
100
NT
---
23
100
Fluconazole
NT
---
NT
---
21
---
NT
---
Streptomycin
24
100
NT
---
NT
---
NT
---
DMF
6 (NZ)
---
6 (NZ)
---
6 (NZ)
---
6 (NZ)
---
Filter paper disk
6 (NZ)
---
6 (NZ)
---
6 (NZ)
---
6 (NZ)
---
Results revealed that the synthesized spiropyrazoline oxindole derivatives possess a variable antimicrobial activity towards the tested microbes. The inhibitory activity of the target compounds is dependent on the type of the substituents R (H, Br, Cl, OCH3, CH3) and R1 (H, Cl) on the phenyl rings, which is consistent with other works in the literature (Ravindar et al., 2018). For example, the unsubstituted compound 2a (R = H, R1 = H) was found to exhibit moderate antimicrobial activity against all bacteria, and significant activity as compared to the standard drug against the fungal strain Candida albicans with a zone of inhibition of 15 mm at a concentration of 1.25 mg/mL. The incorporation of a bromine atom at the substituent R results in a slight increase in the antifungal activity of compound 2 h against Candida albicans (16 mm, 1.25 mg/mL), and a negative effect on the activity against both Gram-positive (Staphylococcus aureus and Bacillus subtilis) and Gram-negative (Escherichia coli) bacterial strains (Fasiuddin et al., 2022). Compound 2i (R = Br, R1 = Cl) which carries two halogens displays antimicrobial activity against all tested organisms at a concentration of 1.25 mg/mL, with potent antibacterial activity against Escherichia coli (21 mm) compared with Streptomycin (24 mm) as standard antibacterial. This result indicates that halogens exert a considerable effect on the activity of the studied spiro-compounds, which is in accordance and consistent with previous research (Ravindar et al., 2018). A significant and remarkable improvement was observed in the activity of compound 2d (R = OCH3, R1 = Cl) against the pathogenic strain Escherichia coli (23 mm, 1.25 mg/mL) with a better activity index percentage of 95 % as compared to streptomycin, with an inhibition zone of 24 mm. This clearly shows the positive effect of chlorine at substituent R1 on antibacterial activity against Gram-negative bacteria. Compound 2 g (R = Cl, R1 = Cl) which carries two chlorine atoms in the phenyl rings is the most active compared to the other congeners against Bacillus subtilis with a zone of inhibition (14 mm, 1.25 mg/mL) and inhibition rate of 87 % in comparison to the standard drug Ampicillin (16 mm). At a concentration of 1.25 mg/mL, congener 2e (R = CH3, R1 = H) showed a significant inhibitory effect compared to positive controls with an inhibition zone diameter of 15.5 and 13 mm against Candida albicans and Bacillus subtilis, respectively. By contrast, the remaining spiro-congeners exhibited weak to moderate antimicrobial activity against the tested microorganisms.
The studied spiropyrazoline oxindoles were further evaluated to determine their minimum inhibition concentrations (MICs) using the broth dilution technique. The results depicted in Table 4 indicate that the studied compounds exhibit promising antibacterial and antifungal activities against the selected pathogenic microorganisms, even at low concentrations. Among all the tested congeners, compounds 2e (R = CH3, R1 = H), 2 h (R = Br, R1 = H) showed a MIC value of 0.156 mg/mL against Candida albicans. Likewise, compound 2i (R = Br, R1 = Cl) showed the lowest MIC values of 0.117 mg/mL and 0.312 mg/mL against Escherichia coli and Staphylococcus aureus, respectively. Additionally, compound 2e (R = CH3, R1 = H) had the lowest MIC value against Bacillus subtilis (0.312 mg/mL) and compound 2d (R = OCH3, R1 = Cl) exhibited a concentration of 0.156 mg/mL against Gram-negative Escherichia coli. NT: No tested.
Compounds
Minimum Inhibitory Concentration (MIC) (mg/mL)
N°
R
R1
Escherichia coli
Bacillus subtilis
Candida albicans
Staphylococcus aureus
2a
–
–
1.25
0.703
0.312
0.781
2b
–
Cl
0.937
0.937
NT
0.937
2c
OCH3
–
NT
NT
0.781
1.25
2d
OCH3
Cl
0.156
0.937
0.781
0.625
2e
CH3
–
NT
0.312
0.156
2.5
2f
Cl
–
0.312
NT
0.625
2
2 g
Cl
Cl
NT
0.390
NT
1.25
2 h
Br
–
0.625
NT
0.156
1.875
2i
Br
Cl
0.117
0.625
0.312
0.312
Ampicillin
NT
0.0781
NT
0.039
Fluconazole
NT
NT
0.0156
NT
Streptomycin
0.0781
NT
NT
NT
3.3 Gaussian calculations
The numerical values generated by Gaussian 09 calculations can be employed to describe the activities of molecules and provide valuable information about their electronic properties. Two most important parameters that can be obtained from these calculations are the Lowest Unoccupied Molecular Orbitals (LUMO) and the Highest Occupied Molecular Orbitals (HOMO). The LUMO is used to provide an explanation for the electron-accepting characteristic of the molecule (Alici et al., 2022; Gömeç et al., 2022a; Kaya et al., 2016; Yalazan et al., 2022), while the HOMO is utilized to describe the electron-donating feature of the molecule (Alici et al., 2022; Gömeç et al., 2022b).
As a result of the calculations, considering the HOMO parameter of the molecules, the antioxidant activity of the molecule 2c is higher because the numerical value of the HOMO parameter is more positive than the other molecules in all the calculations made at all three levels (Table 5)(Bhat et al., 2022). B3LYP, HF, and M062X methods were used in the calculations. The most important reason for using these methods is to examine their effect on the parameter values of molecules. Quantum chemical parameters: (A): Affinity (A= - ELUMO), (I): ionization energy (I=- EHOMO), (μ): chemical potential, (ω): electrophilicity, (η): chemical hardness, (σ): global softness, (ε): nucleophilicity, (χ) electronegativity.
EHOMO
ELUMO
I
A
ΔE
η
μ
χ
Pİ
ω
ε
dipol
Energy
B3LYP/6–31++g(d,p) LEVEL
2a
−5.2336
−2.1954
5.2336
2.1954
3.0382
1.5191
0.6583
3.7145
−3.7145
4.5414
0.2202
5.7174
−35886.7062
2b
−5.3318
−2.2771
5.3318
2.2771
3.0548
1.5274
0.6547
3.8045
−3.8045
4.7381
0.2111
6.3641
−48393.3329
2c
−5.1710
−2.1399
5.1710
2.1399
3.0311
1.5156
0.6598
3.6555
−3.6555
4.4084
0.2268
6.4892
−39002.4408
2d
−5.2771
−2.2086
5.2771
2.2086
3.0686
1.5343
0.6518
3.7429
−3.7429
4.5653
0.2190
7.0147
−51509.0702
2e
−5.1727
−2.1625
5.1727
2.1625
3.0102
1.5051
0.6644
3.6676
−3.6676
4.4686
0.2238
6.2817
−36956.0433
2f
−5.3365
−2.2882
5.3365
2.2882
3.0482
1.5241
0.6561
3.8123
−3.8123
4.7680
0.2097
3.7523
−48393.3013
2 g
−5.4306
−2.3677
5.4306
2.3677
3.0629
1.5315
0.6530
3.8992
−3.8992
4.9637
0.2015
4.7594
−60899.9234
2 h
−5.3465
−2.2874
5.3465
2.2874
3.0591
1.5296
0.6538
3.8170
−3.8170
4.7626
0.2100
3.8126
−105851.5747
2i
−5.4401
−2.3660
5.4401
2.3660
3.0741
1.5370
0.6506
3.9031
−3.9031
4.9557
0.2018
4.7414
−118358.2005
HF/6–31++g(d,p) LEVEL
2a
−7.9262
0.9129
7.9262
−0.9129
8.8391
4.4196
0.2263
3.5066
−3.5066
1.3911
0.7188
5.4638
−35658.3969
2b
−7.9216
0.8463
7.9216
−0.8463
8.7678
4.3839
0.2281
3.5376
−3.5376
1.4274
0.7006
6.0267
−48146.1181
2c
−7.7635
0.9075
7.7635
−0.9075
8.6710
4.3355
0.2307
3.4280
−3.4280
1.3552
0.7379
5.0537
−38756.6040
2d
−7.8854
0.8610
7.8854
−0.8610
8.7464
4.3732
0.2287
3.5122
−3.5122
1.4104
0.7090
6.0911
−51244.2676
2e
−7.8949
0.9162
7.8949
−0.9162
8.8111
4.4056
0.2270
3.4893
−3.4893
1.3818
0.7237
5.9232
−36720.0435
2f
−7.9262
0.9129
7.9262
−0.9129
8.8391
4.4196
0.2263
3.5066
−3.5066
1.3911
0.7188
5.4638
−35658.3969
2 g
−8.1442
0.8691
8.1442
−0.8691
9.0133
4.5066
0.2219
3.6375
−3.6375
1.4680
0.6812
4.3552
−60633.6908
2 h
−8.0353
0.9217
8.0353
−0.9217
8.9570
4.4785
0.2233
3.5568
−3.5568
1.4124
0.7080
3.5445
−105574.3502
2i
−8.1420
0.8713
8.1420
−0.8713
9.0133
4.5066
0.2219
3.6353
−3.6353
1.4662
0.6820
4.3271
−118062.0052
M062X/6–31++g(d,p) LEVEL
2a
−6.5471
−1.1951
6.5471
1.1951
5.3520
2.6760
0.3737
3.8711
−3.8711
2.8000
0.3571
5.5959
−35871.7077
2b
−6.6222
−1.2760
6.6222
1.2760
5.3463
2.6731
0.3741
3.9491
−3.9491
2.9170
0.3428
5.7939
−48377.6511
2c
−6.5028
−1.1470
6.5028
1.1470
5.3558
2.6779
0.3734
3.8249
−3.8249
2.7315
0.3661
6.5737
−38986.1714
2d
−6.5787
−1.2259
6.5787
1.2259
5.3528
2.6764
0.3736
3.9023
−3.9023
2.8448
0.3515
6.5336
−51492.1091
2e
−6.5071
−1.1663
6.5071
1.1663
5.3408
2.6704
0.3745
3.8367
−3.8367
2.7562
0.3628
6.1176
−36940.4426
2f
−6.6505
−1.2942
6.6505
1.2942
5.3563
2.6782
0.3734
3.9723
−3.9723
2.9460
0.3394
3.6401
−48377.6317
2 g
−6.7289
−1.3717
6.7289
1.3717
5.3571
2.6786
0.3733
4.0503
−4.0503
3.0623
0.3266
4.0334
−60883.5541
2 h
−6.6562
−1.2936
6.6562
1.2936
5.3626
2.6813
0.3730
3.9749
−3.9749
2.9464
0.3394
3.6726
−105838.7417
2i
−6.7417
−1.3712
6.7417
1.3712
5.3705
2.6852
0.3724
4.0564
−4.0564
3.0639
0.3264
3.9998
−118344.6531
The energy gap (ΔE) is a critical parameter that measures the energy difference between the numerical values of the HOMO and LUMO parameters (Rezaeivala et al., 2022a). The molecules with a lower energy gap parameter are known to exhibit greater activity compared to other molecules. In contrast, the electro-negativity parameter of the molecules demonstrates the ability of the atoms in the molecules to draw the bond electrons (Rezaeivala et al., 2022a). The activity of the molecule will decrease as this parameter's numerical value increases because more bond electrons will be attracted to the atoms in the molecule.
Electrophilicity can be specified as a measure of the reactivity to withdraw electrons from nucleophiles. Nucleophilicity (ε) is physically the opposite of electrophilicity (1/ω). Therefore, a molecule with a large electrophilicity value has a higher activity. The chemical hardness and softness of a molecule are significant characteristics that serve as indicators of its reactivity and stability. Softness (1/η), the opposite of chemical hardness, is an index of a molecule's polarizability. Indeed, soft molecules may easily donate electrons to an acceptor and are therefore more reactive than hard molecules.
On the other hand, the numerical value of the ΔE energy gap parameter of the molecules is higher in the calculations of the HF level, since the numerical value of the 2c molecule for this parameter is lower than the other molecules; hence, electron transfer will be easier. In addition to these, when the electronegativity value is examined, it is observed that the 2c molecule has a higher antioxidant activity owing to the lower numerical value of the electronegative parameter in the calculations made at all levels.
When considering the equations provided in the method section for the other parameters, a similar order is observed as all other parameters are derived from the numerical values of the HOMO and LUMO parameters. It should be noted that chemical interactions of molecules in biological materials determine the activity when comparing their activity in experimental studies.
After conducting the calculations, some parameters of the molecules are represented in visual form, as shown in Fig. 3. The figure contains four different images for each molecule, including the optimized shape of the molecule and the atoms where HOMO or LUMO orbitals are present. The fourth and last image displays the molecule's electrostatic potentials (ESPs), with red regions indicating higher electron density and blue areas referring to lower electron density (Majumdar et al., 2022). These calculations are crucial in identifying the active sites of the molecules.A representation of optimized structures, HOMO, LUMO, and ESP shapes of Spiropyrazoline oxindole congeners computed by HF/6–31++g(d,p) method.
In the theoretical calculations, the molecules were calculated with three different methods, and it was examined how these three different methods affect the molecule with the highest activity. however, the molecules are structurally very similar to each other. The activity change in the molecule was investigated by binding -Cl, -Br, –CH3, and –OCH3 at two different points in the molecule. It is seen that the –OCH3 bonded molecule in the molecules, namely 2c, has higher activity than the other molecules. The most important reason for this is that the –OCH3 group is a good electron-releasing group, so it donates electrons to the molecule. In addition to all these, it is seen that the electron density in the molecule is on the oxygen atom in the molecule. When this situation creates an interaction, it is obvious that if the electron is donated, it will be over the oxygen atom.
The Gaussian package program was used to examine these compounds' anti-oxidant properties. From the calculations performed with the Gaussian package program, numerous numerical results are obtained. Table 6 contains these obtained numerical values.
BDE
IP
PDE
PA
ETE
2a
87.70
0.74
401.46
337.89
64.31
2b
87.64
0.74
401.40
335.74
66.40
2c
87.16
0.13
401.53
337.87
63.79
2d
87.76
0.73
401.53
336.41
65.85
2e
87.86
0.74
401.62
338.43
63.93
2f
87.28
0.74
401.05
334.98
66.81
2 g
88.47
1.37
401.60
333.50
69.46
2 h
87.45
0.74
401.21
334.90
67.05
2i
87.34
0.74
401.10
332.77
69.07
By eliminating hydrogen from the molecules' NH bonds, calculations for the anti-oxidant properties of the molecules were performed. A parameter connected to the hydrogen atom transfer mechanism (HAT) is the numerical value of the bond dissociation enthalpy (BDE) for the molecules NH bonds.
It was discovered that the compounds with numerical values for the lesser. The lower numerical value of the BDE parameter, the more hydrogen the molecule releases, thus leading to better antioxidant activity. therefore, the molecule with the lowest numerical value of the BDE parameter is 2c with 87.16. Molecule 2c seems to lead to higher antioxidant activity than other molecules. The ionization potential (IP) value of these compounds is determined in the first step of the single electron transfer-proton transfer (SET–PT) process. It is understood that a molecule has a better capacity to give electrons if its IP value is lower. However, another parameter is proton dissociation enthalpy (PDE) values. It is seen that with 0.13 the numerical value of the IP parameter of molecule 2c is the lowest. The lower IP value of the 2c molecule indicates that it can donate electrons more easily than the other molecules. This means that the 2c molecule will more easily capture free radicals. Besides, with 401.05, it is seen that the numerical value of the PDE parameter of molecule 2f is the lowest. A reaction will be simpler if a molecule has a lower PDE value. The numerical value of these two parameters shows that electron and proton are easily removed from the molecule with antioxidant activity. The proton dissociation enthalpy (PDE) values are computed in the second step of the SET-PT process. These two digits are measured by the numerical values of the parameters PA and ETE. A lower numerical value of these parameters indicates higher antioxidant activity. It can be observed that the ETE parameter of molecule 2c has a lower numerical value compared to the other molecules, indicating higher antioxidant activity.
Therefore, based on the all calculations, it can be concluded that molecule 2c exhibits higher antioxidant activity than the other molecules.
3.4 Molecular docking
3.4.1 Docking process validation
To validate the adopted docking process, the original ligands were redocked into their respective protein pockets. From the Fig. 4, it is clear that there is perfect superposition between the original and re-docked ligands, confirming the validity of the adopted docking process.(a) Re-docking of the co-crystallized ligand into the binding site of 5V5Z. (b) Re-docking of the co-crystallized ligand into the binding site of 2CAG. (re-docked ligands highlighted in yellow).
3.4.2 Docking results
The docking outcomes revealed that all docked complexes are thermodynamically favorable; they had negatives binding energies values. The binding energies values of the investigated complexes are summarized in Table 7. Moreover, the binding energies of the investigated complexes were lower than those of the reference drugs (Fluconazole, BHT), which supports their observed antimicrobial activity. In this study, fluconazole and BHT are used as the reference drugs for antifungal activity and antioxidant activity, respectively. Besides that, we noticed that the ligands-5V5Z complexes showed high and comparable values of binding energy, leading to strong coherences. As described in Fig. 6 and Table S1 (Supplementary material), the studied complex’s coherence is principally attributed to the Pi-sigma, Pi-donor hydrogen bond and Pi-Alkyl interactions involved between the ligands and three amino acids namely ILE A:131, CYS A:470 and LEU A:376, respectively. Furthermore, as depicted in Fig. 5(a), ILE A:131, CYS A:470 and LEU A:376 are key residues for the enzymatic activity of the target ligand. These findings justify why compounds 2a, 2c, 2d, 2e, 2f, 2 h and 2i were experimentally determined to be able to act as fungal inhibitors by limiting the growth of Candida albicans strain.
Antioxidant activity
Anti-fungal activity
Ligand-protein Complex
Binding energy
(kcal/mol)Ligand-protein Complex
Binding energy (kcal/mol)
2e-2CAG
−10.0
2a-5V5Z
−10.4
2i-2CAG
−8.3
2c-5V5Z
−10.4
hem
−11.9
2d-5V5Z
−10.3
2e-5V5Z
−10.5
BHT
−7.8
2f-5V5Z
−10.5
2 h-5V5Z
−10.5
2i-5V5Z
−10.4
hem
−11.2
Fluconazole
−7.8
(a) The binding site of 5V5Z. (b) The binding site of 2CAG.
On the other hand, the absolute binding energy value of the 2e-2CAG complex was found to be higher than that exhibited by 2i-2CAG. Indeed, compound 2e was found to be stabilized in the pocket binding of 2CAG by involving Pi-donor hydrogen bond with SER A: 336, Pi-sigma interaction with ALA A: 340, two Pi-Pi interactions with TYR A: 337 and PHE A: 140 strengthened by five Pi-Alkyl interactions with ARG A: 52, PRO A: 141, ALA A: 340 and ARG A: 333, leading to enhancement of its antioxidant activity compared to compound 2i. In addition, among the interacting amino acids, there are three key residues responsible for the enzymatic activity of 2CAG (Fig. 5(b)), namely PHE 140, ALA A: 340 and ARG A:333. Therefore, the findings revealed by docking studies clearly corroborated that of the experimental assay.
3.5 Molecular dynamics simulations
The stability of compound 2e, which exhibited high activity and strong affinity towards the target proteins, in complexation with 2CAG and 5V5Z, was studied through molecular dynamics simulations. Initially, the stability of the systems was investigated by computing their root mean square deviation (RMSD) as expressed in Fig. 7. The RMSD of all systems fluctuated until 20 ns, and then it reached a steady state. The average RMSD values of 2CAG, 5V5Z, 2CAG-2e complex and 5V5Z-2e complex were found to be 0.738, 0.247, 0.736 and 0.294 nm, respectively. Moreover, the ligand 2e exhibited nearly stable RMSD values over time, indicating its stability in the binding pockets of the target proteins. In addition, the RMSD graphs for the investigated complexes displayed a similar profile to their corresponding unbound proteins, reflecting their stability in the MD simulation conditions.2D Diagram of protein–ligand interactions.
(a) RMSD of the backbone of 5V5Z and 5V5Z-2e complex as a function of time. (b) RMSD of the backbone of the 2CAG and 2CAG-2e complex as a function of time.
The root means square fluctuation (RMSF) was also computed to evaluate the flexibility of residues. Stable regions are characterized by lower RMSF values while increasing RMSF values lead to higher flexibility. The RMSF graphs of all examined systems are presented in Fig. 8. The 5V5Z-2e complex exhibited relatively more fluctuation compared to the 2CAG-2e complex (Fig. 8). In addition, except for the terminal positions, many residues were shown to exhibit a law RMSF value, indicating that the investigated systems were stable.(a) RMSF of Cα atoms of 5V5Z in the absence and presence of 2e. (b) RMSF of Cα atoms of 2CAG in the absence and presence of 2e.
The radius of gyration (RoG) is another parameter calculated to study the stability of the investigated systems. RoG is defined as the mass-weighted RMS distance of a collection of atoms from their common center of mass. Fig. 9 presents the RoG graphs corresponding to the target proteins (2CAG and 5V5Z) and its complexes. The average values of RoG exhibited by 5V5Z, 2CAG, 5V5Z-2e complex and 2CAG-2e complex were found to be 2.396, 2.378, 2.388 and 2.372 nm, respectively. Furthermore, during the overall MD simulation, the RoG of all systems remained relatively at a steady state, indicating the stability of the examined complexes in an aqueous environment.(a) RoG of 5V5Z and 5V5Z-2e complex as a function of time. (b) RoG of 2CAG and 2CAG-2e complex as a function of time.
We also assessed the number of hydrogen bonds formed by the studied ligand with their targets (Fig. 10). The obtained results exhibited that the studied ligand 2e formed greater hydrogen bonds with 5V5Z in some poses compared to when it complexed with 2CAG. In addition, almost all positions of the ligand 2e were found to form a hydrogen bond with 5V5Z, indicating its stability throughout the simulation.(a) Number of hydrogens formed by ligand 2e with 5V5Z as a function of time. (b) The number of hydrogens formed by ligand 2e with 2CAG as a function of time.
Solvent accessible surface area (SASA) is another significant parameter describing the change in the protein surface area(Nour et al., 2022). In this study, we calculated the SASA values of 5V5Z, 2CAG and their complexes (Fig. 11). The average SASA values of 2CAG, 2CAG-2e complex, 5V5Z and 5V5Z-2e complex were found to be 243.498, 242.579, 233.028 and 229.590 nm2, respectively (Table 8). Furthermore, we can notice that this parameter remained relatively at a steady state during the simulation, indicating the stability of the investigated systems. In conclusion, all the analyzed parameters including RMSD, RMSF, numbers of hydrogen bonds, SASA and RoG, revealed that the investigated complexes were relatively stable in an aqueous medium.(a) SASA of 5V5Z and 5V5Z-2e complex. (b) SASA of 2CAG and 2CAG-2e complex.
System
Parameter
2CAG
2CAG-2e complex
5V5Z
5V5Z-2e complex
RMSD
0.738 nm
0.736 nm
0.247 nm
0.294 nm
RMSF
0.183 nm
0.208 nm
0.104 nm
0.130 nm
RoG
2.378 nm
2.372 nm
2.396 nm
2.388 nm
SASA
243.498 nm2
242.579 nm2
233.028 nm2
229.590 nm2
3.6 POM (Petra/Osiris/Molinspiration) analyses
POM Theory (Petra/Osiris/Molinspiration), which was developed by Taibi Ben Hadda’s group in collaboration with the American NCI and TAACF, has been highly successful in the fields of pharmacology and drug design (Ben Hadda et al., 2021; Berredjem et al., 2022; Bhat et al., 2021; Titi et al., 2020). Here, our aim is to identify the pharmacophore sites of the screened spiropyrazoline oxindole congeners 2(a-i) by following the POM organigram (Fig. 12).The Concept and Applications of POM Theory in the identification and optimization of pharmacophore sites of various classes of drugs.
The kind of pharmacophore sites of the tested compounds was identified based on their physical and chemical properties using the POM bioinformatics platform (Fig. 12). The findings of the pharmacokinetic properties and bioactivity scores analysis are summarized in Table 9. Based on the results, it can be seen that all evaluated spiranic compounds 2(a-i) are considered safe with no reported side effects. Furthermore, the drug score (DS) is highly promising, with values ranging from 20 % to 31 %.
Compd. no.
MW
(g/mol)
Toxicity Risksa
Drug Score Calculationsb
MUT
TUM
IRRI
REP
cLogP
S
DL
DS
2a
415.50
5.91
−6.84
2.2
0.31
2b
449.94
6.51
−7.21
6.76
0.27
2c
445.52
5.84
−6.5
5.57
0.32
2d
469.97
6.44
−7.23
6.3
0.26
2e
429.52
6.25
−6.82
4.09
0.29
2f
449.94
6.51
−7.21
6.67
0.27
2 g
484.39
7.12
−7.95
6.19
0.23
2 h
494.39
6.63
−7.31
3.78
0.24
2i
528.84
7.24
−8.05
4.53
0.20
), highly toxic: (
), slightly toxic: (
). REP: Reproductive effective, IRRIT: Irritant, TUM: Tumorigenic, MUT: Mutagenic.
The toxicity risks and molecular properties of congeners 2(a-i) were estimated and reported in Table 9. All explored compounds were found to be non-mutagenic, non-tumorigenic, and non-reproductive (Table 9). The spiropyrazoline oxindole congeners 2(a-i) were found to have moderate to good bioactivity score according to the results of the calculations. In fact, these bioactivity scores can be classified as active (if the score > 0), moderately active (if the score is −5.0 to 0.0), and inactive (if the score is less than −5.0). Based on the results of all bioactivity parameters, the majority of the spiropyrazoline oxindole derivatives were found to be biologically active against all the enzymes concerned (Table 10).
Compd
Lipinski parameters calculationsa
Drug-likenessb
TPSA
NONH
NV
VOL
GPCRL
ICM
KI
NRL
PI
EI
2a
44.70
1
1
377.35
−0.14
−0.34
−0.49
−0.15
−0.32
−0.20
2b
44.70
1
1
390.88
−0.14
−0.34
−0.50
−0.17
−0.35
−0.22
2c
53.93
1
1
402.89
−0.17
−0.38
−0.50
−0.16
−0.35
−0.22
2d
53.93
1
1
416.42
−0.30
−0.34
−0.60
−0.28
−0.36
−0.22
2e
44.70
1
1
393.91
−0.17
−0.39
−0.51
−0.17
−0.36
−0.24
2f
44.70
1
1
390.88
−0.14
−0.34
−0.50
−0.17
−0.35
−0.22
2 g
44.70
1
1
404.42
−0.14
−0.33
−0.48
−0.16
−0.34
−0.21
2 h
44.70
1
1
395.23
−0.21
−0.39
−0.52
−0.24
−0.40
−0.25
2i
44.70
1
2
408.77
−0.21
−0.38
−0.52
−0.25
−0.42
−0.27
3.7 Identification of the pharmacophore sites
The identification of the pharmacophore site can be attributed to each molecule, on the basis of the X, Y atomic charge of each pocket and the (X---Y) distance (Fig. 13). The Figs. 13 and 14 show the coexistence of two combined antibacterial (NH–---N) and antifungal/antiviral (O---N) pharmacophore sites with a major problem of the aryl substituent of the central N atom.Atomic charge of the studied Spiro compounds 2(a-i).
Identification of pharmacophore sites.
4 Conclusion
In this study, we have examined the in vitro antioxidant and antimicrobial activities of a series of spiropyrazoline oxindole congeners. The results of the preliminary evaluation indicate that the investigated spiropyrazolines exhibit a moderate to excellent antimicrobial effect on the growth of the tested microbial strains compared to positive controls. The obtained results suggest that the substituents attached to the phenyl rings have an influence on the activity of the studied compounds. In addition, the spirop-congeners 2(a-i) were also assessed for their antioxidant activity. The obtained results indicated that the antioxidant capacity exhibits concentration-dependent increases of the tested congeners. Specifically, the congeners 2c, 2d and 2e, incorporating electron-donating substituents (CH3 and OCH3), can therefore be considered the most active ones in the series. DFT, molecular docking, and MD studies were executed to support and explain the experimental results of the biological activity of the target compounds. The molecular docking studies showed good binding affinities and interactions with the target proteins, catalase compound II and CYP51. Moreover, the MD simulation results suggested a stable conformation and binding patterns in a stimulating environment of the congeners (2CAG-2e and 5V5Z-2e). POM analyses of the relative antimicrobial activity of these derivatives are reported. Interestingly, drug-likeness analysis suggested that the tested spiropyrazoline oxindoles require structural modifications in order to obtain new potentially-active compounds with good oral bioavailability and appropriate brain penetration. Therefore, the results of the present investigation recommend that the studied congeners might be considered valuable candidates that deserve further in-depth investigation. Moreover, the current study provides additional valuable data on the antimicrobial and antioxidant potential of spiro-compounds, which warrants its use in research and development of more potent drug candidates in the future.
Authors contributions
The manuscript was written through contributions of all authors. All authors have given approval to the final version of the manuscript. M.C. and M.B. (Mohamed Bakhouch) designed the experiments, analyzed the data, methodology, wrote–original draft, Wrote–review & editing. K.C. performed the antimicrobial activity assay. A.E.M. performed the antioxidant activity assay. H.N. and S.C. performed the molecular Docking, Molecular dynamics simulations and interpretation of results. B.T. computational DFT and interpretation of results. M.B. (Mohammed Bourhia), M.A.M.A.-S., M.A. and S.M.A.K. Data validation, discussion of the data and manuscript writing and editing, English correction, edited the final version. H.L. and T.B.H. POM analyses and interpretation of results. M.Y. G.A.H. and A.N. conceptualization, methodology, manuscript preparation and review, validation of results, and supervision. All authors reviewed and approved the manuscript.
Acknowledgements
The authors are very much thankful to the staff members of the “Cité de l'Innovation” of the Sidi Mohamed Ben Abdellah University (Morocco). The authors are also grateful to TUBITAK ULAKBIM, High Performance and Grid Computing Center (TRUBA resources) for performing the DFT calculations reported in this paper.
Declaration of Competing Interest
The authors declare that they have no known competing financial interests or personal relationships that could have appeared to influence the work reported in this paper.
References
- “Free Download: BIOVIA Discovery Studio Visualizer - Dassault Systèmes.” https://discover.3ds.com/discovery-studio-visualizer-download (accessed Jun. 13, 2021)., n.d.
- Gromacs: High performance molecular simulations through multi-level parallelism from laptops to supercomputers. SoftwareX. 2015;1–2:19-25.
- [CrossRef] [Google Scholar]
- A robust in vitro anticancer, antioxidant and antimicrobial agents based on new metal-azomethine chelates incorporating Ag(I), Pd (II) and VO (II) Cations: Probing the aspects of DNA interaction. Appl. Organomet. Chem.. 2020;34:1-20.
- [CrossRef] [Google Scholar]
- “ADT / AutoDockTools — AutoDock” http://autodock.scripps.edu/resources/adt (accessed Sep. 18, 2021)., n.d.
- Metronidazole derivatives as a new class of antiparasitic agents: synthesis, prediction of biological activity, and molecular properties. Med. Chem. Res.. 2014;24:1196-1209.
- [CrossRef] [Google Scholar]
- Antimicrobial resistance mechanisms and potential synthetic treatments. Futur. Sci. OA. 2018;4
- [CrossRef] [Google Scholar]
- Potent α-amylase inhibitors and radical (DPPH and ABTS) scavengers based on benzofuran-2-yl(phenyl)methanone derivatives: Syntheses, in vitro, kinetics, and in silico studies. Bioorg. Chem.. 2020;104:104238
- [CrossRef] [Google Scholar]
- Alkyl chain modified metalophthalocyanines with enhanced antioxidant-antimicrobial properties by doping Ag+ and Pd2+ ions. J. Mol. Struct.. 2022;1257:132634
- [CrossRef] [Google Scholar]
- Synthesis of spiroindolone analogue via three components reaction of olefin with isatin and sarcosine: Anti-proliferative activity and computational studies. J. Mol. Struct.. 2020;1204:127500
- [CrossRef] [Google Scholar]
- Bacterial resistance and failure of clinical cure could be produced by oxidative stress in patients with diabetes or cardiovascular diseases during fluoroquinolone therapy. Med. Hypotheses. 2017;103:32-34.
- [CrossRef] [Google Scholar]
- An overview on applications of swissadme web tool in the design and development of anticancer, antitubercular and antimicrobial agents: A medicinal chemist’s perspective. J. Mol. Struct.. 2022;1259:132712
- [CrossRef] [Google Scholar]
- Synthesis and biological evaluation of benzo[b]thiophene acylhydrazones as antimicrobial agents against multidrug-resistant staphylococcus aureus. Biomolecules. 2022;12:131.
- [CrossRef] [Google Scholar]
- Antibacterial studies of hydroxyspiro[indoline-3,9-xanthene]trione against spiro[indoline3,9-xanthene]trione and their use as acetyl and butyrylcholinesterase inhibitors. Microb. Pathog.. 2019;130:95-99.
- [CrossRef] [Google Scholar]
- Density functional thermochemistry. I. The effect of the exchange only gradient correction. J. Chem. Phys.. 1992;96:2155-2160.
- [CrossRef] [Google Scholar]
- Impact of geometric parameters, charge, and lipophilicity on bioactivity of armed quinoxaline, benzothiaole, and benzothiazine : pom analyses of antibacterial and antifungal activity. phosphorus, sulfur. Silicon Relat. Elem.. 2014;189:753-761.
- [CrossRef] [Google Scholar]
- How to face COVID-19: proposed treatments based on remdesivir and hydroxychloroquine in the presence of zinc sulfate. Docking/DFT/POM structural analysis. J. Biomol. Struct. Dyn. 2021:1-14.
- [CrossRef] [Google Scholar]
- Antitumor activity, X-Ray crystallography, in silico study of some-sulfamido-phosphonates. Identification of pharmacophore sites. J. Mol. Struct.. 2022;1250:131886
- [CrossRef] [Google Scholar]
- Synthesis, biological activity and POM/DFT/docking analyses of annulated pyrano[2,3-d]pyrimidine derivatives: Identification of antibacterial and antitumor pharmacophore sites. Bioorg. Chem.. 2021;106:104480
- [CrossRef] [Google Scholar]
- Synthesis, characterization, molecular modeling against EGFR target and ADME/T analysis of novel purine derivatives of sulfonamides. J. Mol. Struct.. 2022;1257:132600
- [CrossRef] [Google Scholar]
- Design, molecular docking, and antimicrobial assessment of newly synthesized phytochemical thymol Mannich base derivatives. J. Mol. Struct.. 2021;1244:130908
- [CrossRef] [Google Scholar]
- Stereoselective synthesis and applications of spirocyclic oxindoles. Org. Chem. Front.. 2021;8:1026-1084.
- [CrossRef] [Google Scholar]
- Design, synthesis and characterization of functionalized pyrazole derivatives bearing amide and sulfonamide moieties from aza-aurones. J. Chem. Sci.. 2020;132:86.
- [CrossRef] [Google Scholar]
- Design, synthesis, characterization, in vitro screening, molecular docking, 3D-QSAR, and ADME-Tox investigations of novel pyrazole derivatives as antimicrobial agents. New J. Chem.. 2022;46:2747-2760.
- [CrossRef] [Google Scholar]
- Crystallographic study, biological evaluation and DFT/POM/Docking analyses of pyrazole linked amide conjugates: Identification of antimicrobial and antitumor pharmacophore sites. J. Mol. Struct.. 2022;1252:131818
- [CrossRef] [Google Scholar]
- Crystallographic study, biological assessment and POM/Docking studies of pyrazoles-sulfonamide hybrids (PSH): Identification of a combined Antibacterial/Antiviral pharmacophore sites leading to in-silico screening the anti-Covid-19 activity. J. Mol. Struct.. 2022;1267:133605
- [CrossRef] [Google Scholar]
- Synthesis, characterization, DFT mechanistic study, antimicrobial activity, molecular modeling, and ADMET properties of novel pyrazole-isoxazoline hybrids. ACS Omega. 2022;7:46731-46744.
- [CrossRef] [Google Scholar]
- Crystal structure, Hirshfeld surface and DFT computations, along with molecular docking investigations of a new pyrazole as a tyrosine kinase inhibitor. J. Mol. Struct.. 2023;1273:134255
- [CrossRef] [Google Scholar]
- Antioxidant and Antimicrobial Activities of Chemically-Characterized Essential Oil from Artemisia aragonensis Lam. against Drug-Resistant Microbes. Molecules. 2022;27:1136.
- [CrossRef] [Google Scholar]
- Proper basis set for quantum mechanical studies of potential energy surfaces of carbohydrates. J. Mol. Struct. (Thoechem). 2002;584:1-4.
- [CrossRef] [Google Scholar]
- QSAR, molecular docking and ADMET properties in silico studies of novel 4,5,6,7-tetrahydrobenzo[D]-thiazol-2-Yl derivatives derived from dimedone as potent anti-tumor agents through inhibition of C-Met receptor tyrosine kinase. Heliyon. 2021;7:e07463.
- [Google Scholar]
- Mortality in Escherichia coli bloodstream infections: Antibiotic resistance still does not make it. J. Antimicrob. Chemother.. 2020;75:2334-2343.
- [CrossRef] [Google Scholar]
- R. Dennington, Keith, T., J. Millam, 2016. GaussView, Version 6. Semichem Inc.
- Antibacterial, antifungal and antioxidant activity of total polyphenols of Withania frutescens.L. Bioorg. Chem.. 2019;93:103337
- [CrossRef] [Google Scholar]
- Promising Antioxidant and Anticorrosion Activities of Mild Steel in 1.0 M Hydrochloric Acid Solution by Withania frutescens L. Essential Oil. Front. Chem.. 2021;9:739273
- [CrossRef] [Google Scholar]
- Synthesis of some novel coumarin isoxazol sulfonamide hybrid compounds, 3D-QSAR studies, and antibacterial evaluation. Sci. Rep.. 2021;11:1-15.
- [CrossRef] [Google Scholar]
- In vitro potential antiviral SARS-CoV-19- activity of natural product thymohydroquinone and dithymoquinone from Nigella sativa. Bioorg. Chem.. 2022;120:105587
- [CrossRef] [Google Scholar]
- Structure Determination and Quantum Chemical Analysis of 1,3-Dipolar Cycloaddition of Nitrile Imines and New Dipolarophiles and POM Analyses of the Products as Potential Breast Cancer Inhibitors. Russ. J. Org. Chem.. 2020;56:1258-1271.
- [CrossRef] [Google Scholar]
- Synthesis, Spectroscopic, Molecular Docking and inhibitory activity of 6-Bromo-2-(4-chlorophenyl)-1H-benzimidazole- a DFT approach. J. Mol. Struct.. 2022;1261:132815.
- [CrossRef] [Google Scholar]
- Frisch, M.J., Trucks, G.W., Schlegel, H.B., Scuseria, G.E., Robb, M.A., Cheeseman, J.R., Scalmani, G., Barone, V., Mennucci, B., Petersson, G.A., Nakatsuji, H., Caricato, M., Li, X., Hratchian, H.P., Izmaylov, A.F., Bloino, J., Zheng, G., Sonnenberg, J.L., Hada, M., Ehara, M., Toyota, K., Fukuda, R., Hasegawa, J., Ishida, M., Nakajima, T., Honda, Y., Kitao, O., Nakai, H., Vreven, T., Montgomery, J.A., Peralta, J.J.E., Ogliaro, F., Bearpark, M., Heyd, J.J., Brothers, E., Kudin, K.N., Staroverov, V.N., Kobayashi, R., Normand, J., Raghavachari, K., Rendell, A., Burant, J.C., Iyengar, S.S., Tomasi, J., Cossi, M., Rega, N., Millam, J.M., Klene, M., Knox, J.E., Cross, J.B., Bakken, V., Adamo, C., Jaramillo, J., Gomperts, R., Stratmann, R.E., Yazyev, O., Austin, A.J., Cammi, R., Pomelli, C., Ochterski, J.W., Martin, R.L., Morokuma, K., Zakrzewski, V.G., Voth, G.A., Salvador, P., Dannenberg, J.J., Dapprich, S., Daniels, A.D., Farkas, O., Foresman, J.B., Ortiz, J.V., Cioslowski, J., Fox, D.J., 2009. Gaussian 09, revision D.01. Gaussian, Inc., Wallingford.
- Synthesis, in silico and investigation of anti-breast cancer activity of new diphenyl urea derivatives: Experimental and computational study. J. Mol. Struct.. 2022;1265:133414
- [CrossRef] [Google Scholar]
- Synthesis of diaryl urea derivatives and evaluation of their antiproliferative activities in colon adenocarcinoma. J. Mol. Struct.. 2022;1254:132318
- [CrossRef] [Google Scholar]
- Ferryl intermediates of catalase captured by time-resolved weissenberg crystallography and UV-VIS spectroscopy. Nat. Struct. Biol.. 1996;3:951-956.
- [CrossRef] [Google Scholar]
- Merck molecular force field. III. Molecular geometries and vibrational frequencies for MMFF94. J. Comput. Chem.. 1996;17:553-586.
- [CrossRef] [Google Scholar]
- Avogadro: An advanced semantic chemical editor, visualization, and analysis platform. J. Cheminform.. 2012;4:1-17.
- [CrossRef] [Google Scholar]
- Assessment of the performance of the M05–2X and M06–2X exchange-correlation functionals for noncovalent interactions in biomolecules. J. Chem. Theory Comput.. 2008;4:1996-2000.
- [CrossRef] [Google Scholar]
- Combined in vitro and in Silico Studies for the anticholinesterase activity and pharmacokinetics of coumarinyl thiazoles and oxadiazoles. Front. Chem.. 2018;6:61.
- [CrossRef] [Google Scholar]
- Synthesis, ADMET properties, and in vitro antimicrobial and antibiofilm activity of 5-Nitro-2-thiophenecarbaldehyde N-((E)-(5-Nitrothienyl)methylidene)hydrazone (KTU-286) against staphylococcus aureus with defined resistance mechanisms. Antibiotics. 2020;9:612.
- [CrossRef] [Google Scholar]
- Determination of corrosion inhibition effects of amino acids: Quantum chemical and molecular dynamic simulation study. J. Taiwan Inst. Chem. Eng.. 2016;58:528-535.
- [CrossRef] [Google Scholar]
- Crystal structures of full-length lanosterol 14α-demethylases of prominent fungal pathogens candida albicans and candida glabrata provide tools for antifungal discovery. Antimicrob. Agents Chemother.. 2018;62
- [CrossRef] [Google Scholar]
- Revisiting activity of some nocodazole analogues as a potential anticancer drugs using molecular docking and DFT calculations. Front. Chem.. 2021;9:628398
- [CrossRef] [Google Scholar]
- Antioxidant properties of methanolic extract of ‘Veronica multifida’ and DFT and HF analyses of its the major flavonoid component. J. Mol. Struct.. 2019;1197:436-442.
- [CrossRef] [Google Scholar]
- Design, synthesis, characterization, and antioxidant activity studies of novel thienyl-pyrazoles. Heliyon. 2021;7:e07592.
- [Google Scholar]
- POM analyses of Raltegravir derivatives: A new reflection enlightening the mechanism of HIV-integrase inhibition. Res. Chem. Intermed.. 2015;41:5121-5136.
- [CrossRef] [Google Scholar]
- Synthesis, structural confirmation, antibacterial properties and bio-informatics computational analyses of new pyrrole based on 8-hydroxyquinoline. J. Mol. Struct.. 2022;1259:132683
- [CrossRef] [Google Scholar]
- Implementation of the charmm force field in GROMACS: Analysis of protein stability effects from correction maps, virtual interaction sites, and water models. J. Chem. Theory Comput.. 2010;6:459-466.
- [CrossRef] [Google Scholar]
- Architectural view of flexible aliphatic–OH group coordinated hemi-directed Pb (II)-salen coordination polymer: Synthesis, crystal structure, spectroscopic insights, supramolecular topographies, and DFT Perspective. J. Inorg. Organomet. Polym Mater.. 2022;32:1159-1176.
- [CrossRef] [Google Scholar]
- Novel pyran-linked phthalazinone-pyrazole hybrids: Synthesis, cytotoxicity evaluation, molecular modeling, and descriptor studies. Front. Chem.. 2021;9:666573
- [CrossRef] [Google Scholar]
- Influence of structural characteristics of substituents on the antioxidant activity of some anthraquinone derivatives. Comput. Theor. Chem. 2015
- [CrossRef] [Google Scholar]
- 1,2,3-Triazole-quinazolin-4(3H)-one conjugates: evolution of ergosterol inhibitor as anticandidal agent. RSC Adv.. 2018;8:39611-39625.
- [CrossRef] [Google Scholar]
- Synthesis of novel spiropyrazoline oxindoles and evaluation of cytotoxicity in cancer cell lines. Eur. J. Med. Chem.. 2014;79:266-272.
- [CrossRef] [Google Scholar]
- 2D-QSAR and molecular docking studies of carbamate derivatives to discover novel potent anti-butyrylcholinesterase agents for Alzheimer’s disease treatment. Bull. Korean Chem. Soc.. 2022;43:277-292.
- [CrossRef] [Google Scholar]
- Polymorphic transitions in single crystals: A new molecular dynamics method. J. Appl. Phys.. 1981;52:7182-7190.
- [CrossRef] [Google Scholar]
- Exploiting the antiproliferative potential of spiropyrazoline oxindoles in a human ovarian cancer cell line. Bioorganic Med. Chem.. 2021;30:115880
- [CrossRef] [Google Scholar]
- Aryl fluorosulfate analogues as potent antimicrobial agents: SAR, cytotoxicity and docking studies. Bioorg. Chem.. 2018;81:107-118.
- [CrossRef] [Google Scholar]
- Reactive oxygen species (ROS) homeostasis and redox regulation in cellular signaling. Cell. Signal.. 2012;24:981-990.
- [CrossRef] [Google Scholar]
- Synthesis, characterization and bioactivity of novel 8-hydroxyquinoline derivatives: Experimental, molecular docking, DFT and POM analyses. J. Mol. Struct.. 2022;1258:132688.
- [CrossRef] [Google Scholar]
- Experimental and theoretical investigation of corrosion inhibition effect of two piperazine-based ligands on carbon steel in acidic media. Colloids Surfaces A Physicochem. Eng. Asp.. 2022;641:128538
- [CrossRef] [Google Scholar]
- Anti-corrosion behavior of 2-((3-(2-morpholino ethylamino)-N3-((pyridine-2-yl) methyl) propylimino) methyl) pyridine and its reduced form on Carbon Steel in Hydrochloric Acid solution: Experimental and theoretical studies. Thin Solid Films. 2022;741:139036
- [CrossRef] [Google Scholar]
- Pandemic lineages of extraintestinal pathogenic Escherichia coli. Clin. Microbiol. Infect.. 2014;20:380-390.
- [CrossRef] [Google Scholar]
- Spiropyrazolines: A Worthy Insight into the Recent Strategies and Synthetic Applications. Lett. Org. Chem.. 2019;16:357-391.
- [CrossRef] [Google Scholar]
- Design, Synthesis, Molecular Docking, and Evaluation Antioxidant and Antimicrobial Activities for Novel 3-Phenylimidazolidin-4-One and 2-Aminothiazol-4-One Derivatives. Molecules. 2022;27:767.
- [CrossRef] [Google Scholar]
- Synthesis and characterization of α-lipoic acid grafted chitosan derivatives with antioxidant activity. React. Funct. Polym.. 2022;172:105205
- [CrossRef] [Google Scholar]
- Synthesis, characterization, X-Ray crystal study and bioctivities of pyrazole derivatives: Identification of antitumor, antifungal and antibacterial pharmacophore sites. J. Mol. Struct.. 2020;1205:127625
- [CrossRef] [Google Scholar]
- AutoDock Vina: Improving the speed and accuracy of docking with a new scoring function, efficient optimization, and multithreading. J. Comput. Chem.. 2010;31:455-461.
- [CrossRef] [Google Scholar]
- Correcting for basis set superposition error in aggregates containing more than two molecules: ambiguities in the calculation of the counterpoise correction. J. Phys. Chem.. 1993;97:2488-2490.
- [CrossRef] [Google Scholar]
- Examination of anti-oxidant properties and molecular docking parameters of some compounds in human body. Turkish Comput. Theor. Chem.. 2020;4:76-87.
- [CrossRef] [Google Scholar]
- Theoretical investigation of stereochemistry and solvent influence on antioxidant activity of ferulic acid. Comput. Theor. Chem.. 2013;1012:33-40.
- [CrossRef] [Google Scholar]
- Free radicals and antioxidants in normal physiological functions and human disease. Int. J. Biochem. Cell Biol.. 2007;39:44-84.
- [CrossRef] [Google Scholar]
- Mitragynine/Corynantheidine Pseudoindoxyls As Opioid Analgesics with Mu Agonism and Delta Antagonism, Which Do Not Recruit β-Arrestin-2. J. Med. Chem.. 2016;59:8381-8397.
- [CrossRef] [Google Scholar]
- Theoretical Study of the Antioxidant Activity of Quercetin Oxidation Products. Front. Chem.. 2019;7:818.
- [CrossRef] [Google Scholar]
- Hartree-Fock Calculations with Skyrme’s Interaction. I. Spherical Nuclei. Phys. Rev. C. 1972;5:626.
- [CrossRef] [Google Scholar]
- Quinoline-fused both non-peripheral and peripheral ZnII and MgII phthalocyanines: Anti-cholinesterase, anti-α-glucosidase, DNA nuclease, antioxidant activities, and in silico studies. Appl. Organomet. Chem.. 2022;36:e6696.
- [CrossRef] [Google Scholar]
- Facile synthesis of spirooxindole-pyrazolines and spirobenzofuranone- pyrazolines and their fungicidal activity. Org. Biomol. Chem.. 2015;13:4869-4878.
- [CrossRef] [Google Scholar]
- The use of spirocyclic scaffolds in drug discovery. Bioorganic Med. Chem. Lett.. 2014;24:3673-3682.
- [CrossRef] [Google Scholar]
- An overview of spirooxindole as a promising scaffold for novel drug discovery. Expert Opinion on Drug Discovery. 2020;15:603-625.
- [CrossRef] [Google Scholar]
- SwissParam: A Fast Force Field Generation Tool for Small Organic Molecules. J. Comput. Chem.. 2012;32:2359-2368.
- [CrossRef] [Google Scholar]
Appendix A
Supplementary data
Supplementary data to this article can be found online at https://doi.org/10.1016/j.arabjc.2023.105465.
Appendix A
Supplementary data
The following are the Supplementary data to this article:Supplementary data 1
Supplementary data 1