Photocatalytic degradation of 1,2-dichlorobenzene using immobilized TiO2/SnO2/WO3 photocatalyst under visible light: Application of response surface methodology
⁎Corresponding author. wazelee@kimia.fs.utm.my (Wan Azelee Wan Abu Bakar)
-
Received: ,
Accepted: ,
This article was originally published by Elsevier and was migrated to Scientific Scholar after the change of Publisher.
Peer review under responsibility of King Saud University.
Abstract
Photodegradation of 1,2-dichlorobenzene over illuminated trimetallic oxide consisting of rutile TiO2 in major portion together with WO3 and SnO2 was studied with respect to the effect of physicochemical properties of the catalyst. The photocatalytic activity enhancement by the presence of surface defects due to calcination temperature was investigated with the information obtained from XRD, DRUV, PL, FESEM and XPS. Calcination of TiO2 at 950 °C resulted in highest activity. Decrease in percentage of degradation of 1,2-dichlorobenzene was noted when it was immobilized on PVC film and chitosan beads. The effect of calcination temperature, catalyst loading and pH was investigated for slurry and after immobilization. Further optimization study was carried out with the aid of response surface methodology utilizing Box–Behnken design. High correlation was obtained for the experimental and the predicted value (R2 = 0.9992, Adj. R2 = 0.9982 and Pred. R2 = 0.9971). Optimization result showed that the maximum percentage of degradation was achieved at calcination temperature of 961.2 °C, with catalyst loading of 0.22 g and pH 7.2. The presence of two intermediates was identified during the reaction using GC–MS. On top of that the photocatalyst could also be reused for several times.
Keywords
1,2-Dichlorobenzene
TiO2 rutile
Surface defects
Immobilization
Thin film
Response surface methodology
1 Introduction
Chlorinated organic compounds have attracted wide attention due to their toxicity and bioaccumulation. It has been a challenging task to decompose the chlorinated volatile organic compounds such as 1,2-dichlorobenzene (DCB). Most of the research works conducted on DCB was in gas phase using catalytic oxidation method and only few photocatalytic studies were employed in aqueous medium. In aqueous systems, chlorinated hydrocarbons exhibit low chemical reactivity and non-biodegradability due to its toxicity (Mahmoud et al., 2012). In earlier study by Lin et al. (2002), TiO2 with fluorinated surface has been used for the photodegradation of 1,2-dichlorobenzene in aqueous under UV light. Comparison of 1,4-dichlorobenzene photodegradation under UV and visible light was conducted by Mahmoud et al. (2012) which showed a slower degradation rate under visible light.
Alternatively, binary metal oxides have been explored and displayed better catalytic activity compared to pure TiO2. Study by Tae Kwon et al. (2000) showed that WO3 doped TiO2 demonstrated higher photocatalytic activity under UV light compared to TiO2 doped with SiO2, SnO2, Al2O3 and ZrO2. Song et al. (1999) claimed that Ag/TiO2 P25 presented higher catalytic activity compared to Cu/TiO2 catalyst. TiO2 P25 doped with SB, Nb and Bi metal ion by sol–gel method had been studied by Whang et al. (2005), with TiO2/Nb found to show good catalytic activity. On another study by the same group, Nd3+ doped TiO2 P25 was reported to exhibit higher catalytic activity compared to silica doped TiO2 (Kim et al., 2006a, 2006b). Chetty et al. (2012) reported remarkable photocatalytic activity and stability was achieved by using Ni/TiO2 for DCB degradation in the presence of ozone. Photodegradation of 1,4-dichlorobenzene under visible light was conducted by Chakraborty and Kebede (2011) using In2O3/TiO2 composite with In2O3 acting as sensitizer for TiO2 by absorbing visible light. All of these studies had reported the same fundamental process that took place in the enhancement of the photocatalytic activity which is the photogenerated charge transfer between the conduction band and valence band of the metal oxides. However, these oxides might be susceptible to catalytic deactivation due to adsorption of dissociative chloride ion that is generated from the decomposition processes. It has been reported that the incorporation of SnO2 could avoid the poisoning of the catalyst and enhance the catalytic activity (Li et al., 2014; Mao et al., 2015).
In early years, enhanced photocatalytic degradation of organic compounds has been associated with the use of anatase TiO2 or TiO2 P25 mixture of anatase/rutile catalyst in nanoparticle size with low surface area. On contrary, recent studies have demonstrated that single catalyst as rutile TiO2 (X. Liu et al., 2014), ZnO (X. Zhang et al., 2014), WO3 (Xie et al., 2012), SnO2 (Wang et al., 2015) and CeO2 (Jiang et al., 2015) with high crystallinity, large particle size with exposed facets and low surface area showed higher photocatalytic activity under visible light. Another important characteristic that has been described to influence the photocatalyst performance was the presence of surface defects. Yan et al. (2013) have mentioned that the existence of surface/bulk defects on anatase and rutile TiO2 improved the separation of photogenerated electron–hole pairs on TiO2 upon irradiation and thus increased the photocatalytic reaction. Recently, hydrogenated TiO2 (Amano and Nakata, 2014; Jiang et al., 2012; Zheng et al., 2012; Zhou et al., 2014) and reduced TiO2 (W. Fang et al., 2014; W.Q.I. Fang et al., 2014; Mao et al., 2014; Ren et al., 2015) have triggered extensive research interest because of the band gap narrowing and reduction of electron–hole recombination due to the synergistic presence of oxygen vacancies and surface disorder on TiO2.
Besides the surface modification of the catalyst, as mentioned above charge transfer and charge separation are the most essential aspects in photocatalytic reaction. Developing TiO2 based multi-heterogeneous photocatalyst by surface modification has been an effective strategy for charge carrier transfer in enhancing the separation of photogenerated electron and holes. The efficiency of charge transfer and separation depends on the position of conduction band and valence band of the metal oxides. A wide range of bimetallic oxides such as TiO2/WO3 (Luevano-Hipolito et al., 2014), TiO2/SnO2 (Sasikala et al., 2009), TiO2/ZnO (Hernandez et al., 2014), TiO2/CeO2 (Deng et al., 2015) and trimetallic oxide heterostructures ZnO/TiO2/SnO2 (Yang et al., 2012), SnO2/rutile TiO2/anatase TiO2/Pt (Zhao et al., 2014), SnO2/TiO2/CdS (Gao et al., 2015), TiO2/WO3/Pt (Wang et al., 2014) have been explored to demonstrate high photocatalytic activity under visible light due to the efficient charge separation and transfer. Meanwhile, the formation of heterojunction on binary metal oxides with exposed facets has also been reported to enhance the activity due to the improved charge carrier separation (Liu et al., 2015).
Based on the above discussion, developing highly active catalysts that are environmentally friendly, cheap and stable is of importance. Therefore, we planned to synthesize multiheterostructure by coupling TiO2 with WO3 and SnO2. All the three metal oxides have different potential energies with conduction band of SnO2 and WO3 positioned to be lower than TiO2 (Yu et al., 2013; Zhang et al., 2013). This would form a staggered band structure that could promote the charge transfer and separation for photocatalytic reaction improvement. Besides, it is known that addition of WO3 increases the surface acidity of the catalyst, thereby promoting the adsorption of water molecule and enhancing the formation of hydroxyl ion which is important in photodegradation reaction (Grabowska et al., 2012). Moreover WO3 acts as an electron acceptor whereby W6+ can easily be reduced to W5+ which further improves the photogenerated charge carriers separation (Riboni et al., 2013) while incorporation of SnO2 could avoid the deactivation of the catalyst. In addition, the mixed oxide TiO2/WO3 and TiO2/SnO2 with surface defects has been reported to enhance the photocatalytic activity under visible light irradiation (S. Liu et al., 2014; Yu et al., 2013). Thus in the preparation of multiheterostructure TiO2/SnO2/WO3, surface defects on the metal oxides have been adjusted by calcination at optimum temperature (result not shown) while mechanical mixing was employed to minimize the structural deterioration. The effect of surface defects and the efficiency of charge transfer in the trimetallic oxides towards the photodegradation of DCB has been explored in this study. In addition to this, the effect of solution pH and photocatalyst loading was considered as well. As most studies on photodegradation were done in slurry mode which is not applicable for industrial use, in this research the performance of photocatalyst immobilized on support materials has been explored. The optimum condition using photocatalyst immobilized on support material was determined using response surface methodology (Box–Behnken design). Response surface methodology (RSM) has been proven to be a useful technique to evaluate the optimum condition and the relative significance of several affecting factors (Jiang et al., 2013). To our knowledge, the use of TiO2/SnO2/WO3 photocatalyst immobilized on support material for the photodegradation of 1,2-dichlorobenzene under visible light and the optimization study has not been reported yet.
2 Material and methods
2.1 Materials
Titanium tetraisopropoxide (TTIP), tungstic acid (H2WO4) and chitosan with low molecular weight were purchased from Sigma Aldrich, tin (IV) chloride (SnCl4·5H2O) from QreC while diethanolamine (DEA), polyethylene glycol (PEG 2000), polyvinyl chloride (PVC), tetrahydrofuran (THF) and acetonitrile were from Merck. Ethanol (EtOH) and hydrogen peroxide 30% solution were obtained from Qrec. 1,2-Dichlorobenzene (DCB) was purchased from BDH (purity > 99%). The stock solution of these compounds was prepared separately at a concentration of 1000 ppm in acetonitrile/water (35:65). The standard solution containing 100 ppm of DCB was prepared by diluting the stock solution with distilled water.
2.2 Preparation of sol–gel/Hydrothermal photocatalyst
The powder photocatalyst of TiO2, WO3 and SnO2 was prepared according to the following modified procedure. TiO2 was prepared by the hydrolysis and condensation reaction of TTIP with EtOH. The TiO2 sol solution was prepared by mixing PEG 2000:EtOH:DEA:TTIP:H2O in the ratio of 1.1:43.7:3.8:7.5:1 one after another (Ali and Hassan, 2008). WO3 sol solution was prepared by dissolving 5.4 g of tungstic acid in 100 mL of hydrogen peroxide solution and stirred to colourless, followed by the addition of PEG (1.35 g) after it was aged for one day (Tada et al., 2004). To prepare SnO2 sol solution, 8.76 g of SnCl4·5H2O was dissolved in 100 mL of ethanol/water mixture in 1:1 ratio (Liu et al., 2006). The sol solutions were then transferred to Teflon vessels and heat treated at 170 °C for 5 h. The obtained product of WO3 and SnO2 was filtered and dried in oven at 95 °C. The filtered TiO2 was left at room temperature to form xerogel and then it was dried in oven. In order to determine the effect of surface defects at different calcination temperatures, TiO2 as the major component was chosen. The dried TiO2 was calcined at 850 °C, 950 °C and 1050 °C for 5 h to study the thermal effect while WO3 and SnO2 were calcined at 850 °C for 5 h. All the calcined products were ground to fine powders.
Trimetallic oxide TiO2/SnO2/WO3 in the optimum atomic ratio of 80:10:10 (from unpublished report), was mixed for 15 min using shaker at a speed of 150 rpm and followed by mechanical grinding. Three sets of trimetallic oxide were prepared and labelled as T(x)/SnO2/WO3 with x referred to TiO2 calcination temperature (x = 850 °C, 950 °C and 1050 °C).
2.3 Preparation of immobilized photocatalyst
The prepared photocatalyst powder was immobilized on two different polymers: (i) PVC as synthetic polymer and (ii) chitosan as the natural polymer. The PVC was prepared in thin film form while chitosan was prepared in beads form.
For the preparation of PVC thin film, 1 g of polyvinyl chloride powder was slowly dissolved in 25 mL of THF solution under vigorous stirring. The obtained clear solution was then poured onto a Petri dish and left for 10 min till the solution starts to solidify. The prepared photocatalyst was spread on top of the semi-solid PVC layer and left overnight to dry. It has to be noted that the formation of semi-solid PVC layer is very important as overdrying would lead to leaching of the catalyst as the catalyst would not be completely held on the film. The dried thin film was then dried at 95 °C for 1 h to remove any moisture.
To prepare the photocatalyst immobilized in chitosan, 1 g of low molecular weight commercial chitosan powder was first dissolved in 2% acetic acid solution and then followed by the addition of 1 g photocatalyst sample. The mixture solution was kept stirring overnight. Then this mixture was added dropwise into 0.5 M NaOH solution to form the beads. The chitosan beads embedded with photocatalyst were filtered and washed several times with distilled water to remove any excess NaOH. The beads were dried in oven at 60 °C.
2.4 Photocatalytic activity measurement
Photocatalytic degradation of DCB was carried out in a pyrex reactor placed in a dark box with the inner part covered with aluminium foil. A compact fluorescence visible lamp (λ > 400 nm, Philips, 36 W) was placed next to the reactor. The experiments were performed with 250 mL solution containing 100 ppm DCB added with 0.1 g of photocatalyst either in powder form or with immobilized photocatalyst. Before illumination, the dispersion was magnetically stirred for 30 min in the dark to allow for adsorption of DCB onto the surface of the photocatalyst. Aliquots of the solution were collected at different time intervals for a total of 240 min. The aliquots were filtered using 0.45 μm membrane filter and directed to UV–Vis spectrophotometer to check the degradation of DCB via its absorption peak at 269 nm. This absorption data was used in the determination of degradation of DCB through comparison with the absorbance at a certain time as a percentage of the initial absorbance.
In order to study the effect of pH, the pH of the solution was adjusted from 4 to 10 by using 0.1 M NaOH or HCl. Meanwhile to study the the effect of catalyst loading, the amount of the catalyst was varied from 0.05 g to 0.40 g. Both the studies were conducted using catalyst in powder form and after immobilization.
2.5 Material characterization
The powder diffraction patterns were recorded by X-ray diffractometer Bruker AXS D5000 utilizing Cu Kα radiation with wavelength 0.15406 nm at 40 kV and 30 mA. Data were collected over the range of 20–80° at 0.050° intervals with 1 s count accumulation per step. The BET specific surface areas of the samples were measured by N2 adsorption/desorption isotherms on a Micrometrics ASAP 2020 analyser. Surface morphologies were investigated using field emission scanning electron microscopy (FESEM, SU8020, Hitachi). Transmission electron microscopy (TEM) and high-resolution transmission electron microscopy (HRTEM) were performed on a JEOL JEM-2010 electron microscope. The diffuse reflectance UV–Vis (DRUV) spectra were taken using a Perkin Elmer Lambda 35 spectrophotometer. The surface electronic states of the samples were determined by X-ray photoelectron spectroscopy (XPS) on Axis Ultra DLD, Shimadzu/Kratos. All the binding energies were calibrated using the contaminant carbon (C 1s = 284.5 eV) as the reference. Atomic Force Microscopy (AFM) and Seiko model SP13800N with contact tip cantilever were used to record the surface roughness. The sample size of 1 μm × 1 μm was placed on Dynamic Force Mode (DFM) sample holder for measurement and software NanoNavi Analysis was used to analyse the data.
For the determination of the by-products, gas chromatograph Perkin Elmer (Clarus 680) with a 30 m, 0.25 mm HP-5MS capillary column coupled with Perkin–Elmer SQ8 mass detector operating at EI mode at 70 eV was utilized. During the reaction 5 mL sample was collected before and after every hour of photodegradation. The solution was extracted using 3 × 3 mL of isooctane and then added with anhydrous sodium sulphate. The collected organic solution was evaporated and then analysed using gas chromatography–mass spectrometry (GC–MS).
2.6 Experimental design
In this study, the optimization of experimental condition for the degradation of 1,2-dichlorobenzene was conducted with the aid of response surface methodology using Box–Behnken design. The study was conducted using Design expert software version 7. Three independent parameters, (i) calcination temperature, (ii) pH and (iii) catalyst loading were varied for the optimization of photodegradation of 1,2-dichlorobenzene. The experimental design was constructed using two coded value for each variable, maximum (+1) and minimum (−1) which are equally spaced as shown in Table 1. The model is expressed by a second order polynomial function defined by Eq. (1):
Independent variable | Range and levels | ||
---|---|---|---|
−1 | 0 | +1 | |
Calcination temperature of TiO2, X1 (°C) | 850 | 950 | 1050 |
pH, X2 | 5 | 7 | 9 |
Catalyst loading, X3 (g) | 0.10 | 0.25 | 0.40 |
3 Results and discussion
3.1 Characterization of the catalyst
The X-ray diffraction (XRD) patterns for TiO2/SnO2/WO3 with TiO2 calcined at different temperatures are depicted in Fig. 1. The TiO2 calcined at 850–1050 °C exists in pure rutile phase with peaks at 2θ = 27.4°, 36.0° and 41.2° denoted to h k l of (1 1 0), (1 0 1) and (1 1 1) which is consistent with reference data JCPDS no. 089-4202. SnO2 nanoparticles with cassiterite type tetragonal form, presented peaks at 2θ = 26.5° (1 1 0), 33.8° (1 0 1) and 39.0° (1 1 1) that matched well with JCPDS no. 072-1147. Meanwhile WO3 exists in monoclinic form (JCPDS no. 072-0677) with the main peaks at 2θ = 23.1°, 23.5°, 24.3° that indicates the presence of h k l plane (0 0 2), (0 2 0) and (2 0 0) respectively. The increase in calcination temperature of TiO2 causes peak narrowing and increased in intensity which signifies grain growth and improved in crystallinity. It has been reported that rutile TiO2 (X. Liu et al., 2014) and rutile SnO2 (Wang et al., 2015) have active facet at crystal plane (1 1 0) and (1 1 1) while for monoclinic WO3 it follows the order of (0 0 2) > (0 2 0) > (2 0 0) (Xie et al., 2012). From the XRD pattern, it could be noted that T1050/SnO2/WO3 has the highest (1 1 0) and (1 1 1) TiO2 peak with low intensity of WO3 (0 2 0) peak while T850/SnO2/WO3 has lower TiO2 peak intensity with increased intensity of WO3 (2 0 0) peak. Among all the three samples, T950/SnO2/WO3 showed balanced peak intensity which indicate the presence of active facets in proper ratio. No other significant changes were observed indicating no substitution of Sn or W into the lattice of TiO2 in this physical mixing. Similar observation was reported by Wu et al. (2013) in the MnO–CeO2 catalyst prepared by grinding using a mortar.
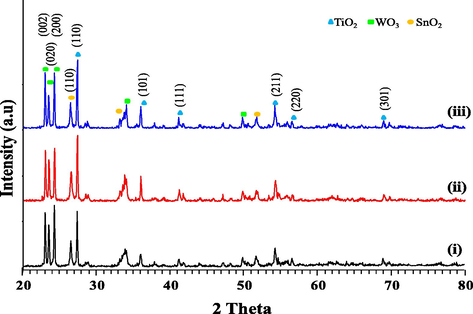
- The X-ray diffraction (XRD) patterns for (i) T850/SnO2/WO3, (ii) T950/SnO2/WO3 and (iii) T1050/SnO2/WO3 phtocatalyst.
The high calcination temperature may have an impact on the lattice strain and thus Williamson–Hall equation (Eq. 2) was used to obtain the particle size of TiO2 (Mote et al., 2012).
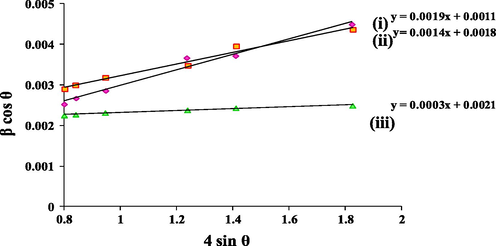
- Particle size determination from Williamson–Hall plot derived from X-ray diffraction (XRD) data of (i) T850/SnO2/WO3, (ii) T950/SnO2/WO3 and (iii) T1050/SnO2/WO3.
The effect of TiO2 calcined at different temperatures on the optical and electronic properties of the trimetallic oxides was evaluated. The absorption of light obtained from diffuse reflectance UV is displayed in Fig. 3a. The absorption edge for all the samples falls in the visible region (λ > 400 nm) which indicates that the entire sample is active at visible light, with enhanced absorption property observed for T950/SnO2/WO3 photocatalyst. The enhancement of the light absorption intensity may be attributed to the presence of oxygen vacancies and Ti3+ (Sekiya et al., 2000) which could be further verified from XPS data. The band gaps of the samples were determined from the [F(R)hν]1/2 versus photon energy (hν) plot as shown in Fig. 3b (Zhang et al., 2015). It could be noted that each spectrum has two slopes. Therefore extrapolating the linear region of the curve gives the band gap values for T850/SnO2/WO3, T950/SnO2/WO3 and T1050/SnO2/WO3 as 2.59/2.77 eV, 2.46/2.55 eV and 2.57/2.74 eV respectively. As there is no doping between the metal oxides, the existence of two band gaps in these samples could be related to the vast difference in the conduction band energy of the metal oxides (Zhang et al., 2015).
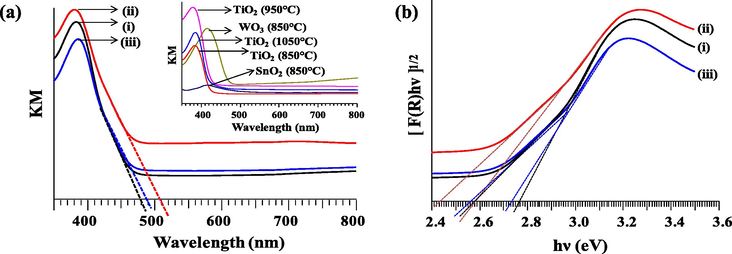
- (a) Kubelka–Munk absorption curve for trimetallic oxide: (i) T850/SnO2/WO3, (ii) T950/SnO2/WO3 and (iii) T1050/SnO2/WO3 with inset plot for single metal oxides calcined at different temperatures. (b) Band gap of trimetallic oxides.
Further verification on the nature of surface defects and chemical state of the metal oxides was carried out using XPS that is known for its surface sensitive analysis. The deconvolution of Ti, Sn, W and O is displayed in Fig. 4. The curve fitting done for Ti leads to three different environments. The binding energy of Ti 2p3/2 and 2p1/2 centred at 458.35–458.60 eV represents Ti4+ in bulk while peak at higher binding energy (459.46–459.80 eV) is suggested to be due to structural defects (Baia et al., 2014). The third peak at lower binding energy which is referred to the presence of Ti3+ was detected in sample T950/SnO2/WO3 and T1050/SnO2/WO3 only which is due to the effect of calcination temperature on TiO2. The larger amount of Ti3+ was observed in T950/SnO2/WO3 (9.96%) but reduced to 4.41% in T1050/SnO2/WO3 due to increased crystallinity. For Sn (Fig. 4b), two doublets were observed with their spin orbit of 3d5/3 and 3d3/2. Several research works have referred the presence of two doublets with the existence of Sn2+ and Sn4+. However the binding energy for Sn2+ according to the NIST database is 485.6 eV. Thus in this study, the first peak centred at 486.20–486.37 eV (3d5/2) with the major portion, was attributed to Sn4+ in bulk while a smaller ratio at higher binding energy (486.99–487.05 eV) is suggested to be due to the structural defect as observed in the Ti. Meanwhile, the curve of W 4f spectra was fitted with three pairs of spin orbit (4f7/2 and 4f5/2). The reduced W5+ was observed at low binding energy (34.03–34.13 eV) in all the samples with different ratios. The second 4f7/2 peak at binding energy 35.03–35.29 eV was denoted as W6+ while the third peak at higher binding energy (35.81–36.60 eV) was referred to structural defect. The presence of surface structural defects at the higher binding energy in each metal (as shown in Fig.4a–c) could be attributed to the surface atomic disorder caused by changes in the bonding and lattice strain upon calcination at high temperature (Sun, 2003). The amounts of W5+ present in T850/SnO2/WO3, T950/SnO2/WO3 and T1050/SnO2/WO3 are 4.2%, 14.4% and 9.4% respectively. Compared to the single WO3 with only 3.6% W5+ (result not shown), the larger amount of W5+ in all the trimetallic oxides signifies the occurrence of charge transfer towards WO3. This is in agreement with observation by Wu et al. (2013). Furthermore, the observed shift in the binding energies of Sn and W also indicates the interaction between the metal oxides (G. Zhang et al., 2014).
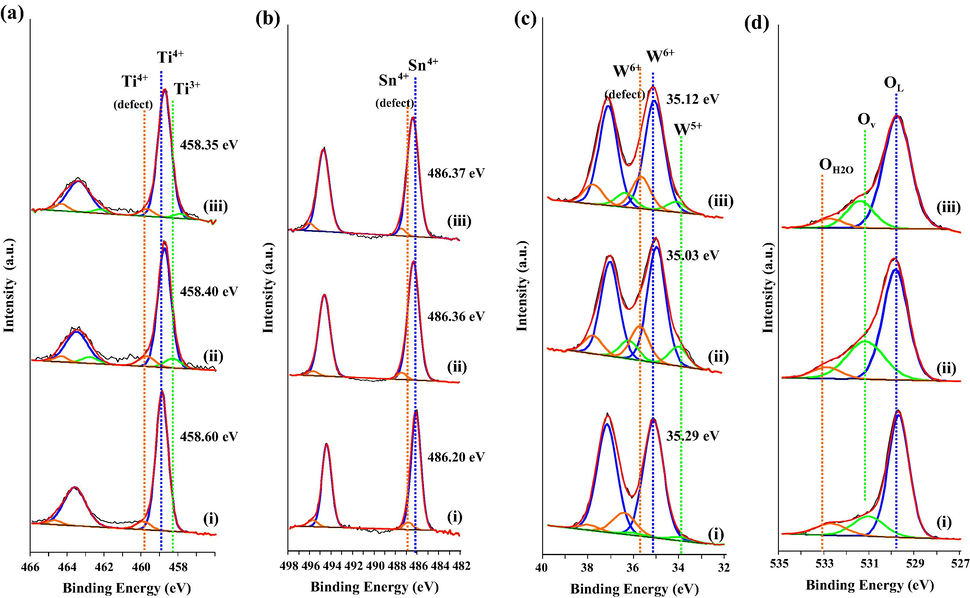
- X-ray photoelectron spectroscopy of (i) T850/SnO2/WO3, (ii) T950/SnO2/WO3 and (iii) T1050/SnO2/WO3 photocatalyst showing the deconvolution for (a) Ti 2p, (b) Sn 3d, (c) W 4f and (d) O 1s.
The formation of Ti3+ and W5+ also indicates the existence of oxygen vacancies which could be determined from the amount of adsorbed oxygen species. The asymmetric XPS spectrum of O 1s peak (Fig. 4d) revealed the presence of different oxygen species on the surface of the photocatalyst. The peak at binding energy around 530.0 eV, 531.5 eV and 532.9 eV could be ascribed to the lattice oxygen, oxygen vacancies or surface adsorbed oxygen and surface adsorbed H2O (Li et al., 2014; X. Zhang et al., 2014). T950/SnO2/WO3 photocatalyst demonstrated the highest concentration of Ti3+, W5+ and oxygen vacancies which is advantageous for the enhancement of photocatalytic activity.
Since the recombination of excited electrons and holes can amplify the PL emission signal, photoluminescence emission is a significant technique to investigate the separation efficiency of the photogenerated charge carriers in a semiconductor (Choudhury and Choudhury, 2014). Fig. 5 shows the photoluminescence spectra attained for all the photocatalysts which were almost identical in shape and position. The emission peaks that appeared between 420 nm and 465 nm may be attributed to the emission of band gap transition with the energy of emission corresponding to the band gap energy between different valence bands and conduction bands that exist in the trimetallic oxides. Meanwhile, the secondary PL emission peaks at 500 nm and above may be attributed to defects in the samples (Zhang et al., 2015). The emission intensity of T950/SnO2/WO3 that is lower than T850/SnO2/WO3 and T1050/SnO2/WO3 (Fig. 5) signifies a lower recombination rate of photogenerated electrons and holes. This could be correlated to the higher amount of oxygen vacancies that exist in T950/SnO2/WO3 resulting in a decreased PL intensity (Ma et al., 2014). Therefore, T950/SnO2/WO3 photocatalyst with suppressed electron–hole recombination rate is expected to increase the photocatalytic reaction.
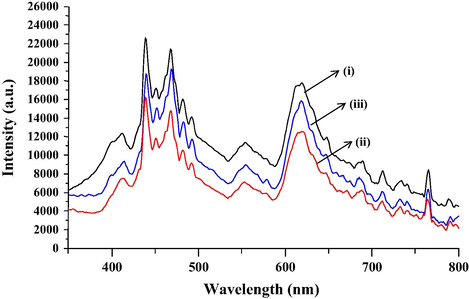
- Photoluminescence spectra of (i) T850/SnO2/WO3, (ii) T950/SnO2/WO3 and (iii) T1050/SnO2/WO3.
Morphologies of the samples were characterized using FESEM and TEM. Fig. 6 clearly points out that all the samples are irregularly shaped. The surface morphology for T850/SnO2/WO3 photocatalyst obtained from FESEM (Fig. 6a), showed wide distribution of small agglomerated SnO2 particles over TiO2 calcined at 850 °C. With the increase in calcination temperature of TiO2 to 950 °C, the existence of surface structural defects could be observed in T950/SnO2/WO3 that has sharp edges/cutting (Fig. 6b), but was not observed in T850/SnO2/WO3. These exposed surfaces with sharp edges are the reactive facets that contribute to their excellent activities in photocatalytic reaction. From Fig. 5b, it also revealed that the larger TiO2 and WO3 particles are closely bound with smaller SnO2 particles partially adsorbed on the surface. However, further increase in the calcination temperature of TiO2 to 1050 °C caused increase in the particle size as also revealed by XRD data. As shown by Fig. 6c, large particles could be seen in T1050/SnO2/WO3 catalyst. Even though the existence of facet could still be observed, the particles are not very closely bounded which might lead to inefficient charge transfer.
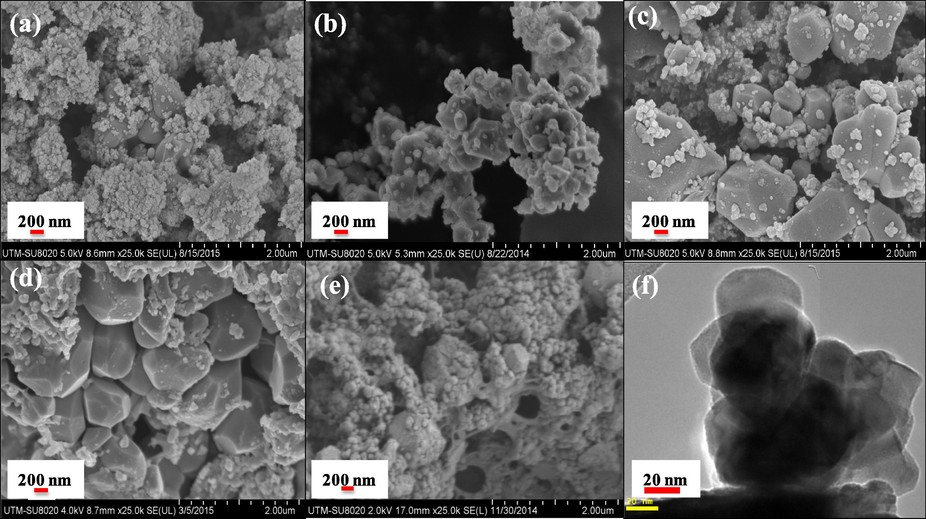
- FESEM image of sample (a) T850/SnO2/WO3, (b) T950/SnO2/WO3, (c) T1050/SnO2/WO3, (d) T950/SnO2/WO3 immobilized on PVC film, (e) T950/SnO2/WO3 immobilized on chitosan beads and (f) TEM for T950/SnO2/WO3.
Meanwhile, Fig. 6d and e presents the surface morphology of the T950/SnO2/WO3 photocatalyst immobilized on PVC and chitosan. From Fig. 6d, it could be noted that the surface of the photocatalyst with sharp edges was slightly covered by the PVC while almost diminished when immobilized using chitosan (Fig. 6e). To further understand the contact between the metal oxides, image from TEM was obtained as shown in Fig. 6f. It could be seen from the TEM image that all the metal oxides are interconnected which is beneficial for the interparticle charge transfer and to improve the photocatalytic reaction. The HRTEM image has been presented in Fig. S2 (supporting document). Since the particle size calculated from XRD was large, the BET surface area obtained was low as shown in Table 2. The low surface area with small variation in BET surface area would not result in any obvious differences in the catalytic performance of the trimetallic oxide.
Sample | BET specific surface area (m2/g) | Total pore volume (cm3/g) | Average pore diameter (nm) |
---|---|---|---|
T850/SnO2/WO3 | 4.075 | 0.0395 | 106.73 |
T950/SnO2/WO3 | 2.720 | 0.0460 | 282.46 |
T1050/SnO2/WO3 | 1.599 | 0.0371 | 92.80 |
3.2 Effect of support on photocatalytic activity
The use of photocatalyst in powder form to study the reusability of catalyst is time consuming and might cause weight loss during the process of filtering and drying. This leads to the use of support materials. Hence, in this study PVC and chitosan were utilized as support materials. Based on the characterization, the potential photocatalyst T950/SnO2/WO3 was used to investigate the effect of support on photocatalytic activity. To determine the efficiency of these support materials, photocatalyst immobilized on PVC and chitosan was compared with the powder photocatalyst. The experimental results (Fig. 7) showed that the activity decreased when the photocatalyst was immobilized on PVC and chitosan. Lower photocatalytic activity was observed when the photocatalyst was immobilized on chitosan. These results could be correlated with the method of preparation followed by surface morphologies obtained from FESEM.
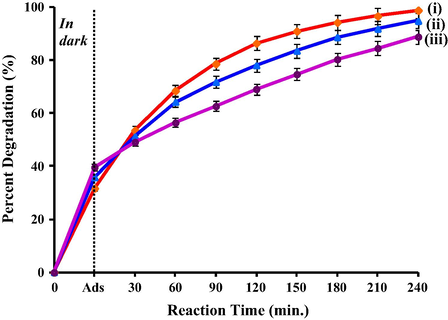
- Photocatalytic degradation of 1,2-dichlorobenzene using T950/SnO2/WO3 photocatalyst (i) in powder form, (ii) immobilized on PVC film and (iii) immobilized on chitosan in beads form.
Notably, the high activity was achieved when using powder photocatalyst which is due to the exposed surface that has large contact with the pollutants (Fig. 6b). However when the photocatalyst was spread on top of PVC film, the catalysts are now partially embedded in the film and not 100% of the surfaces are exposed (Fig. 6d). Thus, lesser amount of pollutants is in-contact with the exposed surface causing slight decline in the activity. On the other hand, during the immobilization with chitosan, the photocatalyst was mixed together with chitosan in acetic acid solution before forming chitosan beads. The chitosan polymer is highly porous material which is sufficiently packed to form beads (Guibal, 2005). In this case, the photocatalyst particles have been encapsulated within the chitosan polymer networks, therefore reducing large amount of catalyst with exposed surface (Fig. 6e). Hence, this observation could be directly related to the lower catalytic activity attained when photocatalyst in chitosan beads was employed. Nevertheless, the higher dark adsorption observed could be associated with the adsorption capability of the porous structured chitosan (Sabar et al., 2015). Consequently, this study highlights the importance of photocatalyst with exposed surface for the photodegradation of DCB. Thus, the following study on optimization was conducted using T950/SnO2/WO3 photocatalyst immobilized on PVC film.
3.3 Effect of calcination temperature
Calcination temperature plays an important role in surface morphology, structural defects and surface defects. Hence, in this study the effect of calcination temperature on one of the metal oxides was considered. As TiO2 exists in major portion in this trimetallic oxide, the effect of calcination temperature was evaluated using TiO2 calcined at different temperatures. Comparison with powder form photocatalyst was also conducted. The photocatalytic performances of the trimetallic oxide with different calcination temperatures are shown in Fig. 8. The photocatalyst in powder form demonstrated higher catalytic activity compared to when it was immobilized on PVC film. This could be correlated to the reduced number of exposed surface photocatalyst as discussed in Section 3.2. Both powder and immobilized photocatalyst exhibited the highest photodegradation of DCB when TiO2 calcined at 950 °C was used in the trimetallic oxide. The UV absorbance spectrum for degradation of DCB using T950/SnO2/WO3 photocatalyst immobilized on PVC is displayed in Fig. S3 (supporting document).
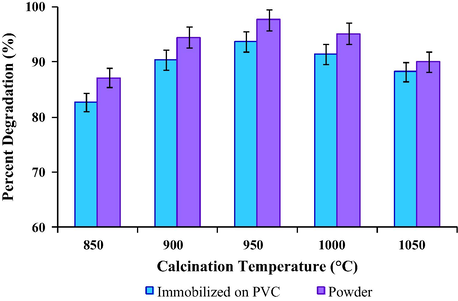
- Photocatalytic degradation of 1,2-dichlorobenzene using Tx/SnO2/WO3 photocatalyst in powder form and immobilized on PVC film (with x referred to calcination temperature of TiO2) conducted for 4 h under visible light.
The effect of calcination temperature on the photodegradation of DCB by using trimetallic oxide photocatalysts immobilized on PVC film over the reaction time is presented in Fig. 9. The photocatalytic activity of the single and binary metal oxides was included as well in Fig. 9 for comparison. It could be noted that all the metal oxides are active at visible light which is in agreement with UV–Vis absorption spectra (inset of Fig 3a). TiO2 calcined at 950 °C showed high catalytic activity compared to WO3 and SnO2. In this study, SnO2 showed fairly good catalytic activity under visible light which could be correlated to the existence of structural defect as depicted by XPS (Fig. 4b). The catalytic activity was improved when 10% of WO3 was mixed with TiO2. The enhancement of the photocatalytic activity was observed with the addition of 10% SnO2 forming trimetallic oxide T950/SnO2/WO3 (80:10:10). On the contrary, T850/SnO2/WO3 demonstrated lower catalytic activity then the binary metal oxide which could be associated with the effect of TiO2 calcined at 950 °C (T950). From Fig. 9, it could be noted that almost high adsorption of DCB was observed using the trimetallic oxides. The high adsorption of organic pollutant is essential for enhancing photocatalytic reaction. Nevertheless, even though TiO2 with different calcination temperature was used in the trimetallic oxide photocatalyst, the variation in the per cent of adsorbed DCB was small. This could be related to the identical ratio of WO3 in all the trimetallic oxides. As reported by Albonetti et al. (2008), in the presence of Lewis acid (TiO2 and SnO2) and Bronsted acid site (WO3), DCB would initially be adsorbed on Bronsted acid site. This phenomenon is also true for pure WO3 which has higher adsorption compared to TiO2 and SnO2. A comparison of the adsorption capability and photocatalytic reaction of DCB using T950/SnO2/WO3 for 4 h is displayed in Fig. S4 (supporting document).
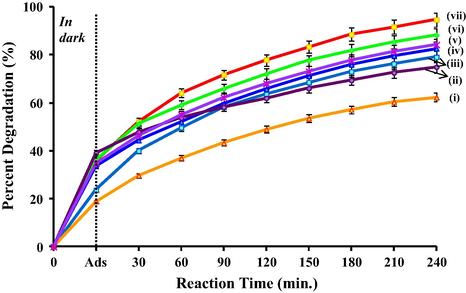
- Photocatalytic degradation of 1,2-dichlorobenzene under visible light using (i) SnO2, (ii) WO3, (iii) TiO2 calcined at 950 °C (T950), (iv) T850/SnO2/WO3, (v) T950/WO3 (90:10), (vi) T1050/SnO2/WO3 and (vii) T950/SnO2/WO3 photocatalysts immobilized on PVC film.
The influence of surface defects on the behaviour of the trimetallic oxides is of particular interest that contributed to the distinction in the photocatalytic activity. Based on XPS data, the presence of Ti3+ in different concentrations was observed in T950/SnO2/WO3 and T1050/SnO2/WO3; however, Ti3+ was not observed in T850/SnO2/WO3. The deviation in the concentration of Ti3+ in TiO2 was the effect of different calcination temperature. However, XPS analysis also revealed that all the trimetallic oxides have higher concentration of W5+ when compared to the pure WO3 calcined at 850 °C. This data showed the occurrence of charge transfer between the metal oxides and formation of heterojunction by the interaction of metal oxides which is proposed in Scheme 1. In this reaction, the enhanced photocatalytic activity of the trimetallic oxide compared to the pure metal oxides could be due to two factors: (i) the improved charge transfer and charge separation by multiple pathways as proposed in Scheme 1 and (ii) the presence of high concentration of oxygen vacancies. As the conduction band of SnO2 and WO3 is lower than TiO2, electrons in the conduction band of TiO2 can be transferred to that of SnO2 and WO3 under visible light irradiation. Meanwhile holes from the lower valence band of SnO2 and WO3 could be transferred to TiO2. This improves the separation and migration of photogenerated carriers and reduced the electron–hole recombination rate. A large number of electrons on WO3 generate more W5+ and increase the formation of
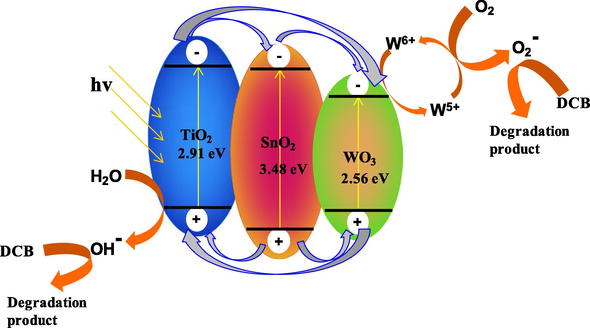
- Proposed schematic diagram for charge transfer in T950/SnO2/WO3 upon irradiation under visible light.
3.4 Effect of pH
Photocatalytic reactions for DCB in solution with different pH levels are presented in Fig. 10. As it could be perceived, the photocatalytic activity increased with the increase in value of pH. It has been suggested that the effect of solution pH on the photocatalytic degradation is a complex subject associated with the reaction mechanism and the adsorption characteristics of substrate onto photocatalyst surface. According to the principle of heterogeneous photocatalysis, the concentration of OH− ions is critical for the generation of •OH radicals. Thus at higher pH value, the formation of hydroxyl ion was favoured (Huang et al., 2014). Nevertheless, it should be noted that the lifetime of •OH radicals is very short and photocatalytic reactions can only take place at or near the surface of photocatalyst. Due to the non-ionic property of DCB, the neutral medium seems beneficial for the adsorption of DCB. As a result, considering the combined effect of the generation of OH− and the interaction between DCB and the surface of the photocatalyst, the photocatalytic degradation of DCB was most efficient in neutral medium (Wang and Ku, 2007).
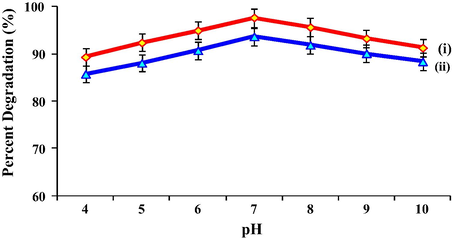
- Photocatalytic degradation of 1,2-dichlorobenzene with 0.1 g T950/SnO2/WO3 photocatalyst (i) in powder form and (ii) immobilized on PVC film at different pH range, conducted for 4 h under visible light.
3.5 Effect of catalyst loading
Typically it is known that photocatalytic activity increases with catalyst loading up to a certain value beyond which the activity decreases. Similar experimental result was attained in this study which is presented in Fig. 11. The degradation efficiency rapidly increased with loading of photocatalyst of up to 0.25 g for powder sample and after immobilized on PVC film. Above this point, the photocatalytic activity started to decline. The influence of catalyst loading on the photodegradation of DCB can be explained in terms of the accessible exposed surface site for the adsorption of DCB and illuminated area which are essential to promote the photocatalytic reaction. The higher loading of powder photocatalyst caused excess of particles and increased the thickness of the photocatalyst in slurry mode. According to Choi et al. (2000), light penetration is exponentially extinguished along the depth while reaction rate is proportional to the absorbed light intensity. Therefore, the increase in thickness of catalyst particles leads to shielding effect or reduction of light penetration and decrease in activity. On the other hand, the higher loading of photocatalyst immobilized on PVC causes reduction of exposed surface photocatalyst. During the immobilization of photocatalyst on PVC, higher loading caused some of the catalyst to sink into PVC liquid due to the increase in density. The photocatalyst that sank in the PVC during preparation got embedded in the PVC film upon drying and therefore reducing the exposed surface of catalyst. To justify this statement, the surface roughness of immobilized photocatalyst with different loading was evaluated using AFM.
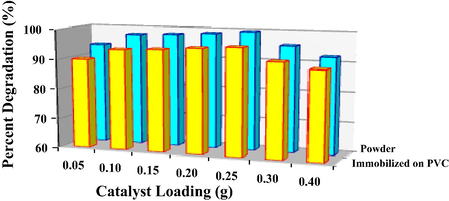
- Photocatalytic degradation of 1,2-dichlorobenzene with T950/SnO2/WO3 photocatalyst in powder form and immobilized on PVC film at different catalyst loading. The reaction was conducted under visible light for 4 h.
Fig. 12 portrays the three dimensional images of PVC film surface with photocatalyst loading of 0.25 g and 0.30 g. The differences in the surface roughness are shown by the images (Fig. 12) and by the root-mean-square roughness value (Rrms). The Rrms value for photocatalyst loading of 0.25 g is 7.293 nm and 4.630 nm for 0.30 g loading. The low Rrms value proves the reduction in the amount of catalyst exposed on surface (Stefanov et al., 2016). Consequently, the decrease in number of catalyst particles on PVC surface leads to reduction of adsorbed light intensity and the contact between photocatalyst and pollutant which caused the decline in activity.
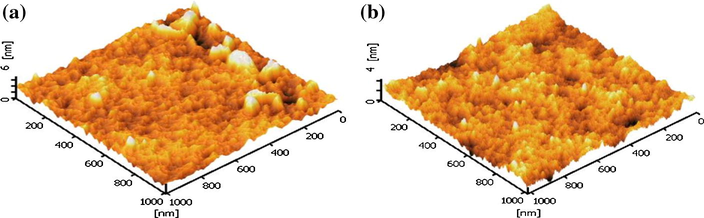
- AFM image of T950/SnO2/WO3 immobilized on PVC with catalyst loading of (a) 0.25 g and (b) 0.30 g.
3.6 Optimization of degradation conditions using RSM approach
For the response surface methodology involving Box–Behnken design, a total of 17 experiments were conducted for three factors at three levels (Table 1). The design used for the optimization and the responses observed and predicted for the 17 experiments are depicted in Table 3 (supporting document).
From the experimental design, an empirical second order polynomial equation was developed that correlates the response and the three different process variables as shown in Eq. (3):
The experimental data for the degradation of DCB were statistically analysed by analysis of variance (ANOVA) and the result is displayed in Table 4 (supporting document). The significance of the model was determined by the variation attributed from the model and the experimental error; which is performed by F-value. Meanwhile, adequacy of the model is evaluated from the difference between the observed and the predicted response value (residual error). Hence, the ANOVA of the second order quadratic polynomial model for the response with model F-value of 1000.50 and its corresponding p-values of <0.0001 showed that the model was significant. The property of the fit polynomial model was determined by the coefficient of determination (R2) that quantitatively evaluates the correlation between the experimental data and the predicted response (Khataee et al., 2010). The R2 value of 0.9992 is in good agreement with the adjusted R2 (0.9982) and predicted R2 (0.9971) which is considerably high, thus advocating a high correlation between the observed values and the predicted values. This indicates that the regression model provides an excellent explanation of the relationship between the independent variables and response. Furthermore the ANOVA result also showed that the lack of fit F-values of 0.24 implies that the lack of fit is not significant as the p-values were >0.05. The insignificant lack of fit confirmed the predictability of the model.
ANOVA results also indicate that all the independent variables of the quadratic polynomial model A, B and C, the interaction between calcination temperature with catalyst loading (AB) and the quadratic terms A2, B2 and C2 are statistically significant as their p-values are <0.05. The most significant factor that influences the degradation of DCB as estimated by ANOVA is the effect of calcination temperature on the catalyst, followed by catalyst loading. The pH has a minor effect on the overall catalytic activity. The ANOVA result exemplified that the two-variable interactions had no significant effect on the pH (p > 0.05). The interaction between the variables and the optimum condition was further evaluated from the 3D plot of response surface shown in Fig. 13. From the surface response model, the optimized per cent degradation was estimated as 95.86% with calcination temperature of TiO2 961.2 °C, catalyst loading of 0.22 g and pH 7.16. Verification of the estimated result at the proposed condition was carried out. The obtained experimental value of 95.84% is in good agreement with the predicted result of 95.89%, therefore validated the findings of response surface optimization. On the whole, ANOVA and the 3D plots illustrated that the calcination temperature plays the major role in structuring the catalyst surface and enhancing the photocatalytic activity at visible light.
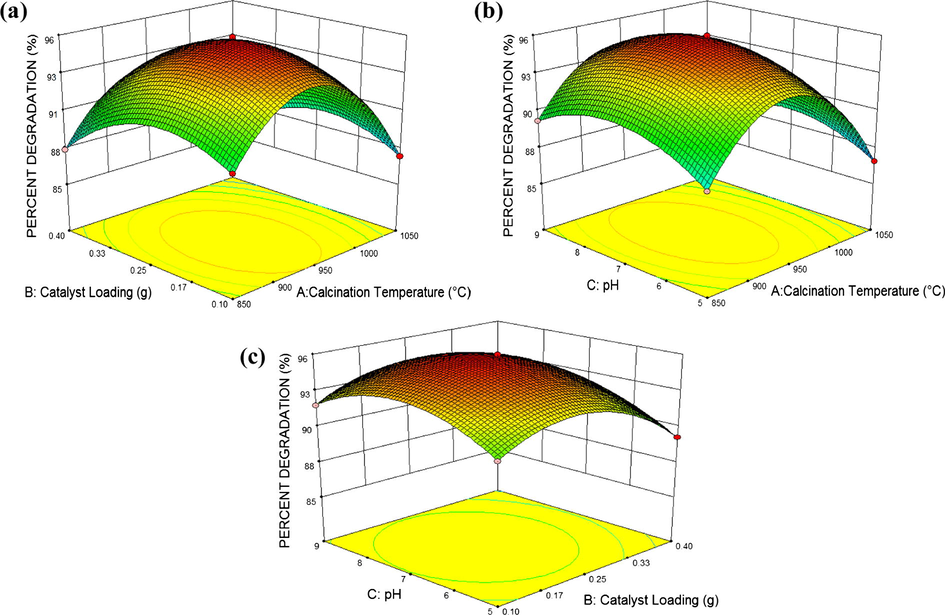
- DCB per cent degradation and the interaction between (a) calcination temperature vs loading, (b) calcination temperature vs pH, and (c) catalyst loading vs pH presented in 3D surface response plot.
3.7 Efficiency of the photocatalyst
For further affirmation of the efficiency of the photocatalyst, the decomposed by-product after 4 h of reaction was analysed by GC–MS (Fig. S5). After 4 h, the final by-product was identified as 4-chloro-4-hydroxy-3-butenal based on the mass spectra fragmentation ions (Scheme 2). Several other peaks obtained could not be identified from the MS spectra library. The removal of chloride ion was verified by the presence of AgCl white precipitate upon the addition of AgNO3 and by the reduction of pH from 7.0 to 6.7.
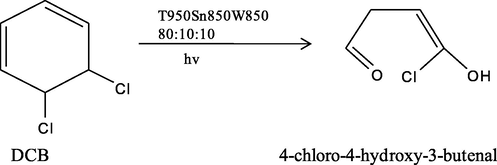
- By-product obtained from photodegradation of DCB using T950/SnO2/WO3 photocatalyst under visible light for 4 h.
It is also important to determine the stability and the reproducibility of the photocatalyst. Hence the photocatalyst was examined by repeating the reaction procedure. At the end of each reaction, the photocatalyst was cleansed using methanol and washed thoroughly using distilled water in order to get rid of any DCB compound or by-products adsorbed on the photocatalyst. The photocatalyst was then dried in oven at 80 °C for 30 min prior to reuse for the next reaction. Fig. 14 illustrates the performance of the T950/SnO2/WO3 photocatalyst after six consecutive runs with an average of 97% degradation which demonstrates the stability of the catalyst and thus its efficiency.
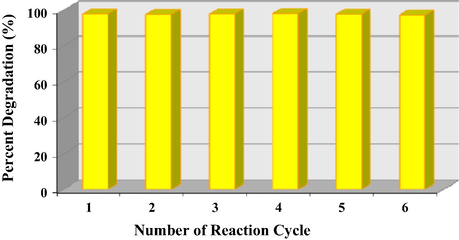
- Reproducibility efficiency of T950/SnO2/WO3 catalyst immobilized on PVC film.
4 Conclusions
The performance of trimetallic metal oxide TiO2/SnO2/WO3 in the ratio of 80:10:10 prepared from rutile TiO2 calcined at 950 °C, WO3 and SnO2 calcined at 850 °C showed high degradation of DCB in aqueous under visible light. Comparison of the activity on different support materials showed that powder form > immobilized with PVC film > chitosan beads. An conventional way optimization study was conducted on the effect of calcination temperature, pH and catalyst loading which demonstrated that the optimum condition was obtained when TiO2 calcined at 950 °C was used in the preparation of the trimetallic oxide with catalyst loading of 0.25 g and solution pH of 7. This result is in good agreement with optimization study using response surface methodology technique. ANOVA revealed that calcination temperature was the predominant factor influencing the photocatalytic activity which is related to the presence of surface defects. Therefore, in this study the enhanced photocatalytic activity was achieved due to the efficient charge transfer and the effect of large amount of oxygen vacancies. Meanwhile the detection of by-product during the reaction and the reproducibility of the photocatalyst T950/SnO2/WO3 verified the efficiency of the photocatalyst.
Acknowledgements
The authors are grateful to Universiti Teknologi Malaysia for financial support under GUP 04H97 and Ministry of Higher Education, Malaysia is acknowledged for the scholarship given to Renugambaal Nadarajan.
References
- The role of acidity in the decomposition of 1,2-dichlorobenzene over TiO2-based V2O5/WO3 catalysts. Appl. Catal. A Gen. 2008
- [CrossRef] [Google Scholar]
- Degradation studies on paraquat and malathion using TiO2/ZnO based photocalatyst. Malaysian J. Anal. Sci.. 2008;12:77-87.
- [Google Scholar]
- High-temperature calcination and hydrogen reduction of rutile TiO2: a method to improve the photocatalytic activity for water oxidation. Appl. Catal. B Environ.. 2014;158–159:202-208.
- [CrossRef] [Google Scholar]
- TiO2/WO3/Au nanoarchitectures’ photocatalytic activity “from degradation intermediates to catalysts’ structural peculiarities” Part II: aerogel based composites – fine details by spectroscopic means. Appl. Catal. B Environ.. 2014;148–149:589-600.
- [CrossRef] [Google Scholar]
- Efficient decomposition of organic pollutants over In2O3/TiO2 nanocomposite photocatalyst under visible light irradiation. J. Clust. Sci.. 2011;23:247-257.
- [CrossRef] [Google Scholar]
- Efficient conversion of 1,2-dichlorobenzene to mucochloric acid with ozonation catalyzed by V2O5 loaded metal oxides. Appl. Catal. B Environ.. 2012;117–118:18-28.
- [CrossRef] [Google Scholar]
- Photocatalytic degradation of polychlorinated dibenzo-p-dioxins on TiO2 film under UV or solar light irradiation. Environ. Sci. Technol.. 2000;34:4810-4815.
- [Google Scholar]
- Oxygen defect dependent variation of band gap, Urbach energy and luminescence property of anatase, anatase-rutile mixed phase and of rutile phases of TiO2 nanoparticles. Phys. E: Low-Dimens. Syst. Nanostruct.. 2014;56:364-371.
- [CrossRef] [Google Scholar]
- Low temperature catalytic combustion of 1,2-dichlorobenzene over CeO2–TiO2 mixed oxide catalysts. Appl. Catal. B Environ.. 2015;181:848-861.
- [CrossRef] [Google Scholar]
- A new approach to prepare Ti3+ self-doped TiO2 via NaBH4 reduction and hydrochloric acid treatment. Appl. Catal. B Environ.. 2014;160–161:240-246.
- [CrossRef] [Google Scholar]
- Fluorine-doped porous single-crystal rutile TiO2 nanorods for enhancing photoelectrochemical water splitting. Chemistry. 2014;20:11439-11444.
- [CrossRef] [Google Scholar]
- Synergistic effects in three-dimensional SnO2/TiO2/CdS multi-heterojunction structure for highly efficient photoelectrochemical hydrogen production. Sol. Energy Mater. Sol. Cells. 2015;141:101-107.
- [CrossRef] [Google Scholar]
- Surface properties and visible light activity of W-TiO2 photocatalysts prepared by surface impregnation and sol–gel method. Appl. Catal. B Environ.. 2012;117–118:351-359.
- [CrossRef] [Google Scholar]
- Heterogeneous catalysis on chitosan-based materials: a review. Prog. Polym. Sci.. 2005;30:71-109.
- [CrossRef] [Google Scholar]
- Optimization of 1D ZnO@TiO2 core–shell nanostructures for enhanced photoelectrochemical water splitting under solar light illumination. ACS Appl. Mater. Interfaces. 2014;6:12153-12167.
- [CrossRef] [Google Scholar]
- Hydrothermal synthesis of SrTiO3 nanocubes: characterization, photocatalytic activities, and degradation pathway. J. Taiwan Inst. Chem. Eng.. 2014;45:1927-1936.
- [CrossRef] [Google Scholar]
- Insights into the surface defect dependence of photoreactivity over CeO2 nanocrystals with well-defined crystal facets. ACS Catal.. 2015;5:4851-4858.
- [CrossRef] [Google Scholar]
- Optimization of photocatalytic performance of TiO2 coated glass microspheres using response surface methodology and the application for degradation of dimethyl phthalate. J. Photochem. Photobiol. A Chem.. 2013;262:7-13.
- [CrossRef] [Google Scholar]
- Characterization of oxygen vacancy associates within hydrogenated TiO2: a positron annihilation study. J. Phys. Chem. C. 2012;116:22619-22624.
- [CrossRef] [Google Scholar]
- Optimization of photocatalytic treatment of dye solution on supported TiO2 nanoparticles by central composite design: intermediates identification. J. Hazard. Mater.. 2010;181:886-897.
- [CrossRef] [Google Scholar]
- Nd3+-doped TiO2 nanoparticles prepared by sol-hydrothermal process. Mater. Sci. Forum. 2006;510–511:122-125.
- [CrossRef] [Google Scholar]
- Photocatalytic property of SiO2/TiO2 nanoparticles prepared by sol-hydrothermal process. J. Electroceram.. 2006;17:899-902.
- [CrossRef] [Google Scholar]
- SnOx–MnOx–TiO2 catalysts with high resistance to chlorine poisoning for low-temperature chlorobenzene oxidation. Appl. Catal. A Gen.. 2014;482:363-369.
- [CrossRef] [Google Scholar]
- Reusable adsorbents for dilute solution separation. 6. Batch and continuous reactors for the adsorption and degradation of 1,2-dichlorobenzene from dilute wastewater streams using titania as a photocatalyst. Sep. Purif. Technol.. 2002;28:87-102.
- [CrossRef] [Google Scholar]
- Creation of Cu2O@TiO2 composite photocatalysts with p–n heterojunctions formed on exposed Cu2O facets, their energy band alignment study, and their enhanced photocatalytic activity under illumination with visible light. ACS Appl. Mater. Interfaces. 2015;7:1465-1476.
- [CrossRef] [Google Scholar]
- One-pot synthesis of TiO2–WO3 composite nanocrystallites with improved photocatalytic properties under natural sunlight irradiation. Mater. Sci. Semicond. Process.. 2014;25:106-111.
- [CrossRef] [Google Scholar]
- UV and visible light photoelectrocatalytic bactericidal performance of 100% 111 faceted rutile TiO2 photoanode. Catal. Today. 2014;224:77-82.
- [CrossRef] [Google Scholar]
- Characteristics of nano Ti-doped SnO2 powders prepared by sol–gel method. Mater. Sci. Eng., A. 2006;426:274-277.
- [CrossRef] [Google Scholar]
- Synthesis, characterization and photocatalytic activity of WO3/TiO2 for NO removal under UV and visible light irradiation. Mater. Chem. Phys.. 2014;148:208-213.
- [CrossRef] [Google Scholar]
- Photocatalytic removal of NOx over visible light responsive oxygen-deficient TiO2. J. Phys. Chem. C. 2014;118:7434-7441.
- [CrossRef] [Google Scholar]
- Photolysis and photocatalysis of 1,4 dichlorobenzene using sputtered TiO2 thin films. Mater. Sci. Forum. 2012;734:215-225.
- [CrossRef] [Google Scholar]
- In situ preparation of a Ti3+ self-doped TiO2 film with enhanced activity as photoanode by N2H4 reduction. Angew. Chemie – Int. Ed.. 2014;53:10485-10489.
- [CrossRef] [Google Scholar]
- Enhancement of resistance to chlorine poisoning of Sn-modified MnCeLa catalysts for chlorobenzene oxidation at low temperature. RSC Adv.. 2015;5:10040-10047.
- [CrossRef] [Google Scholar]
- Williamson-Hall analysis in estimation of lattice strain in nanometer-sized ZnO particles. J. Theor. Appl. Phys.. 2012;6:6.
- [CrossRef] [Google Scholar]
- Controllable synthesis and tunable photocatalytic properties of Ti3+-doped TiO2. Sci. Rep.. 2015;5:10714.
- [CrossRef] [Google Scholar]
- WO3–TiO2 vs. TiO2 photocatalysts: effect of the W precursor and amount on the photocatalytic activity of mixed oxides. Catal. Today. 2013;209:28-34.
- [CrossRef] [Google Scholar]
- Photocatalytic removal of reactive Red 4 dye by immobilised layer-by-layer TiO2/cross-linked chitosan derivatives system. Desalin. Water Treat.. 2015;3994:1-7.
- [CrossRef] [Google Scholar]
- Highly dispersed phase of SnO2 on TiO2 nanoparticles synthesized by polyol-mediated route: photocatalytic activity for hydrogen generation. Int. J. Hydrogen Energy. 2009;34:3621-3630.
- [CrossRef] [Google Scholar]
- Absorption spectra of anatase TiO2 single crystals heat-treated under oxygen atmosphere. J. Phys. Chem. Solids 2000
- [CrossRef] [Google Scholar]
- Photocatalytic activity of Cu/TiO2 with oxidation state of surface-loaded copper. Bull. Korean Chem. Soc.. 1999;20:957-960.
- [Google Scholar]
- Gas-phase photocatalytic activity of sputter-deposited anatase TiO2 films: effect of (0 0 1) preferential orientation, surface temperature and humidity. J. Catal.. 2016;335:187-196.
- [CrossRef] [Google Scholar]
- Oxidation electronics: bond–band–barrier correlation and its applications. Prog. Mater Sci.. 2003;48:521-685.
- [CrossRef] [Google Scholar]
- Deactivation of the TiO2 photocatalyst by coupling with WO3 and the electrochemically assisted high photocatalytic activity of WO3. Langmuir. 2004;20:4665-4670.
- [Google Scholar]
- Photocatalytic behavior of WO3-loaded TiO2 in an oxidation reaction. J. Catal.. 2000;191:192-199.
- [CrossRef] [Google Scholar]
- Multi-heterojunction photocatalysts based on WO3 nanorods: structural design and optimization for enhanced photocatalytic activity under visible light. Chem. Eng. J.. 2014;237:29-37.
- [CrossRef] [Google Scholar]
- Synthesis of monodisperse walnut-like SnO2 spheres and their photocatalytic performances. J. Nanomater.. 2015;2015
- [CrossRef] [Google Scholar]
- Effect of solution pH on the adsorption and photocatalytic reaction behaviors of dyes using TiO2 and Nafion-coated TiO2. 2007;302:261-268.
- Photocatalytic properties of the transition metal doped TiO2 powder prepared by sol-gel process. Key Eng. Mater.. 2005;280–283:647-650.
- [CrossRef] [Google Scholar]
- Synergistic effect between MnO and CeO2 in the physical mixture: electronic interaction and NO oxidation activity. J. Rare Earths. 2013;31:1141-1147.
- [CrossRef] [Google Scholar]
- Crystal facet-dependent photocatalytic oxidation and reduction reactivity of monoclinic WO3 for solar energy conversion. J. Mater. Chem.. 2012;22:6746.
- [CrossRef] [Google Scholar]
- Understanding the effect of surface/bulk defects on the photocatalytic activity of TiO2: anatase versus rutile. Phys. Chem. Chem. Phys.. 2013;15:10978-10988.
- [CrossRef] [Google Scholar]
- Preparation and characterization of SnO2/ZnO/TiO2 composite semiconductor with enhanced photocatalytic activity. Appl. Surf. Sci.. 2012;258:8704-8712.
- [CrossRef] [Google Scholar]
- Enhanced photoelectrocatalytic performance of SnO2/TiO2 rutile composite films. J. Mater. Chem. A. 2013;1:10727.
- [CrossRef] [Google Scholar]
- Defect Chemistry of the metal cation defects in the p- and n-doped SnO2 nanocrystalline films. J. Phys. Chem. C. 2014;118:18097-18109.
- [Google Scholar]
- Hierarchical nanostructure of WO3 nanorods on TiO2 nanofibers and the enhanced visible light photocatalytic activity for degradation of organic pollutants. CrystEngComm. 2013;15:5986.
- [CrossRef] [Google Scholar]
- The role of Sn in enhancing the visible-light photocatalytic activity of hollow hierarchical microspheres of the Bi/BiOBr heterojunction. Phys. Chem. Chem. Phys.. 2015;17:8078-8086.
- [CrossRef] [Google Scholar]
- Effect of aspect ratio and surface defects on the photocatalytic activity of ZnO nanorods. Sci. Rep.. 2014;4:4596.
- [CrossRef] [Google Scholar]
- Synthesis, characterization, and photocatalytic properties of SnO2/Rutile TiO2/Anatase TiO2 heterojunctions modified by Pt. J. Phys. Chem. C. 2014;118:23117-23125.
- [Google Scholar]
- Hydrogenated titania: synergy of surface modification and morphology improvement for enhanced photocatalytic activity. Chem. Commun.. 2012;48:5733.
- [CrossRef] [Google Scholar]
- Ordered mesoporous black TiO2 as highly efficient hydrogen evolution photocatalyst. J. Am. Chem. Soc.. 2014;136:9280-9283.
- [CrossRef] [Google Scholar]
Appendix A
Supplementary material
Supplementary data associated with this article can be found, in the online version, at https://doi.org/10.1016/j.arabjc.2016.03.006.
Appendix A
Supplementary material