Translate this page into:
Research for reducing minimum miscible pressure of crude oil and carbon dioxide and miscible flooding experiment by injecting citric acid isopentyl ester
⁎Corresponding author. 1259248282@qq.com (Guangjuan Fan)
-
Received: ,
Accepted: ,
This article was originally published by Elsevier and was migrated to Scientific Scholar after the change of Publisher.
Peer review under responsibility of King Saud University.
Abstract
The minimum miscible pressure is one of the key factors to realize miscible flooding. As the minimum miscible pressure in the research area is higher than the formation fracture pressure, miscible flooding cannot be formed. To address this problem, it is necessary to find a way to reduce the minimum miscible pressure. Citric acid isopentyl ester can not only be dissolved in crude oil, but also be dissolved in carbon dioxide. Therefore, citric acid isopentyl ester was chosen to reduce the minimum miscible pressure in this research. The effect of citric acid isopentyl ester on reducing the minimum miscible pressure was measured by the method of long slim tube displacement experiment. The minimum miscible pressure in the research area was 29.6 MPa. The experimental results show that the minimum miscible pressure could decrease significantly with increasing the injected slug size of citric acid isopentyl ester, but the decrease became smaller and smaller. The optimum injected slug size of the chemical reagent is 0.003 PV. Under the condition of the slug size, the minimum miscible pressure was 24.1 MPa. The reduction was 5.5 MPa. The reduction rate was 18.58%. The research results have important guiding significance for enhancing oil recovery in the research area.
Keywords
Minimum miscible pressure
Long-slim-tube displacement experiment
Miscible flooding experiment
Carbon dioxide flooding
Enhanced oil recovery
1 Introduction
Enhanced oil recovery (EOR) has always been the focus of attention of researchers and countries all over the world. The carbon dioxide injection technology has become an enhanced oil recovery technology that is widely applied in the world (Azizkhani and Gandomkar, 2020; Mogensen, 2016; Sun et al., 2020; Liu et al., 2020; Tohar et al., 2016). Compared to other oil displacement technology, carbon dioxide injection has the advantages of wide application, low cost, significantly improved oil recovery (Razavi et al., 2016; Chen et al., 2020; Birdja et al., 2019; Ahmadi et al., 2017; Zhang et al., 2020; Mutailipu et al., 2019). In addition, carbon dioxide can be miscible at low pressure compared with other gases, and the use of carbon dioxide injection can save a lot of hydrocarbon gas (Gong et al., 2020; Wu et al., 2020; Shao et al., 2020; Kaufmann and Connelly, 2020; Zhang et al., 2020). At the same time, carbon dioxide flooding can solve the problem of carbon dioxide sequestration to a certain extent, reduce greenhouse gas emissions into the atmosphere, and alleviate the pressure of the environmental pollution (Yang et al., 2019; Fathinasab et al., 2018; Guo et al., 2017; Qin et al., 2015; Song et al., 2014; Hawthorne et al., 2014). So carbon dioxide flooding has a broad application prospect.
The laboratory experiment and field test show that the effect of carbon dioxide miscible flooding to improve oil recovery of light to medium viscosity crude oil is better than that of the immiscible flooding (Han et al., 2016; Yang et al., 2015; Moghaddam and Dehaghani, 2017). However, According to the result of the laboratory miscible experiment, the minimum miscible pressure in the research area was 29.6 MPa, which was higher than the reservoir pressure, so that the miscible flooding cannot be formed in the research area. Therefore, the research for reducing the minimum miscible pressure can carve out a new way for comprehensive realization of miscible flooding to significantly improve oil recovery in research area.
The following are the main methods to reduce the minimum miscible pressure of CO2 flooding (Wu et al., 2015; Jing, 2014).
Miscible solvent method. In this method, the miscible solvent is injected into the ground and diffused in the formation to dissolve with the crude oil to form a miscible flooding zone; the injected CO2 gas contacts with the miscible zone to achieve miscibility at low pressure, so as to improve the oil recovery (Zhang et al., 2013; Peng et al., 2012). Zhang et al. carried out a large number of experiments on single-component methanol, ethanol, n-hexane, n-octane, and multi-component petroleum ether, light oil and other miscible solvents (Zhang et al., 2013). Peng et al. injected a certain ratio of liquefied gas into CO2 to study its influence on the miscible pressure of CO2 flooding (Peng et al., 2012). But the miscible volume method has a large injection volume and high cost, and its application is limited by hydrocarbon gas, which may escape from CO2 gas during reservoir displacement, leading to that miscible displacement cannot be formed.
Supercritical CO2 microemulsion method. In this method, the minimum miscible pressure is reduced by using the surfactant dissolved in supercritical CO2 to form nanoscale aggregates (Lou et al., 2013; Dong et al., 2013). Both the supercritical and microemulsion technologies are needed for the supercritical CO2 microemulsion technology to reduce the miscible pressure of CO2 flooding. Dong Zhaoxia and other scholars obtained the supercritical CO2 microemulsion using the Di-(2-ethylhexyl) sodium sulfosuccinate (AOT) as the surfactant and ethanol as the auxiliary, and the minimum miscible pressure between supercritical CO2 microemulsion and alkane was investigated, including alkane carbon number, temperature, surfactant addition and other factors (Dong et al., 2013). Guo et al. studied the minimum miscible pressure between supercritical CO2 microemulsion and Daqing heavy oil (Dong et al., 2013). But the supercritical CO2 microemulsion can only reduce the miscible pressure and improve the oil recovery to some extent.
Low-molecular-weight non-ionic surfactant method. Guo et al. selected CAE and CAF as non-ionic surfactants to reduce the minimum miscible pressure, and investigated the oil solubility, solubility in supercritical CO2 and viscosity reduction of the two reagents. The results show that such surfactants can reduce the minimum miscible pressure (Guo et al., 2012). But low-molecular-weight non-ionic surfactants are poorly compatible with supercritical CO2, which limits the solubility of CO2 and polar macromolecular substances, resulting in the insignificant oil displacement effect.
CO2-philic non-ionic surfactant method. Such surfactants proposed in domestic and foreign literatures are mainly siloxanes and fluorides (Harrison et al., 1994; Eastoe and Gold, 2005; Rocha et al., 2003). Due to the high solubility in CO2, the fluoride surfactant has good affinity with CO2. For example, the perfluoroalkylpolyether (PEPE) surfactant (Fink et al., 1999; Zhang and Zhong, 2005), which can reduce the surface tension between CO2 and water to a very low level, is the most effective CO2-philic surfactant at present. Hoefling and other scholars discovered that PEPE has better solubility in supercritical CO2 (Hoefling et al., 1992). DeSimone and Keiper found that polyacrylic acid 1,1-dihydroperfluorooctanoic acid (PFOA) has strong CO2-philic performance, then synthesized the block polymer surfactant of PFOA and polystyrene (PS), and developed the supercritical CO2 cleaning technology (DeSimone and Keiper, 2001). But the fluoride surfactant in the CO2-philic non-ionic surfactant method contains toxic fluorinated compounds, which are difficult to degrade and may pollute the environment. These problems greatly limit the application of fluoride surfactants. Compared with fluorinated compounds, silicon-containing compounds are less toxic, but expensive and difficult to degrade.
Citric acid isopentyl ester is an oil-soluble surfactant, which is insoluble in water, but can be mutually soluble with crude oil, alcohol and other organic solvents. It is non-toxic, tasteless, low volatile, non-bacterial, non-irritating, flame retardant and degradable. As environmentally friendly reagents, citric acid isopentyl ester can not only be dissolved in crude oil to reduce viscosity, but also be dissolved in carbon dioxide to reduce the interfacial tension between crude oil and carbon dioxide, thus reducing the minimum miscible pressure between crude oil and carbon dioxide (Chen et al., 2012; Torabi et al., 2012; Doughty et al., 2001). Therefore, citric acid isopentyl ester was chosen to reduce the minimum miscible pressure of carbon dioxide and the crude oil in the research area.
2 Minimum miscible pressure determination and injection ratio optimization
2.1 Experimental material
The crude oil used in the experiment is the simulated crude oil prepared by the ground crude oil and natural gas in the research area according to the formation conditions and fluid characteristics. The experiment temperature was 108.5 °C. The viscosity of crude oil was 1.88 mPa·s at 108.5 °C and 23.8 MPa (the temperature and the pressure of the formation). The molecular weight of C7+ was 347.29 g/mol, and the density of C7+ is 0.8971 g/cm3. The composition data of crude oil components is shown in Table 1.
Component
Mole fraction (%)
Component
Mole fraction (%)
CO2
0.025
n-C4
1.220
N2
0.971
i-C5
0.166
C1
28.739
n-C5
1.417
C2
1.035
C6
2.318
C3
0.809
C7+
63.178
i-C4
0.122
The purity of carbon dioxide gas was 99.9%, and the properties were exactly the same as that of carbon dioxide injected in the research area. The reagent injected was citric acid isopentyl ester.
2.1.1 Preparation of the formation oil sample
The experimental formation oil was prepared with ground oil and natural gas (Choubineh et al., 2016; Li et al., 2019; Ebrahimi and Khamehchi, 2014). Preparation process: Put a certain amount of ground oil into the high pressure physical instrument PVT barrel, seal it and heat it up to the formation temperature, then put the gas into the PVT barrel at the saturation pressure, stir and increase pressure to the formation pressure, measure the saturation pressure of crude oil and the dissolved gas-oil ratio. If the saturation pressure and the actual formation oil saturation pressure are not equal, we should adjust the amount of dissolved gas in the PVT barrel until the saturation pressure and gas-oil ratio measured are equal to that of the formation oil.
2.1.2 Preparation of citric acid isopentyl ester
Citric acid isopentyl ester was an oil-soluble surfactant. It was prepared by the reaction of citric acid and corresponding alcohols under the condition of catalyst. The following is the synthesis process of citric acid isopentyl ester.
2.1.2.1 Experimental materials and apparatus for preparing citric acid isopentyl ester
Main experimental materials: citric acid, isopentyl alcohol, p-toluene sulfonic acid, anhydrous sodium sulfate, sodium bicarbonate, sodium chloride, and so on.
Main experimental apparatus: round-bottom flask, upper water separator, reflux condensing tube and separating funnel, and so on.
2.1.2.2 Experimental process for preparing citric acid isopentyl ester
Adding chemicals: add citric acid and excessive isopentyl alcohol to the round-bottom flask.
Adding catalyst: heat the reaction mixture to 95 °C while stirring, and add p-toluene sulfonic acid into the mixture after citric acid was dissolved.
Heating: heat to 110 °C. The water produced was discharged through the manifold. The reaction began when water was discharged from the condensing tube, and ended when water discharge stopped in the condensing tube. P-toluene sulfonic acid as a catalyst can be used for a long time. The chemical reaction product of citric acid and isopentyl alcohol is shown in Fig. 1. The chemical reaction equation of citric acid and isopentyl alcohol is shown in Fig. 2.
Filtration: after the reaction was cooled, filter out the catalyst, which can be washed and dried for reuse.
Filtering out citric acid: add saturated sodium bicarbonate solution and saturated sodium chloride solution. The mixture was separated with the separatory funnel when the temperature reduced to 25 °C. The mixture after adding saturated sodium bicarbonate is shown in Fig. 3. The mixture after adding saturated sodium chloride is shown in Fig. 4.
Water removal: the organic layer was filtered with anhydrous sodium sulfate.
Distillation: heat to 131.2 °C. After isopentyl alcohol was evaporated, we got citric acid isopentyl ester. The esterification rate and purity of citric acid isopentyl ester can reach 98%. The citric acid isopentyl ester obtained after distillation is shown in Fig. 5.
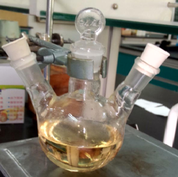
- The chemical reaction product of citric acid and isopentyl alcohol.
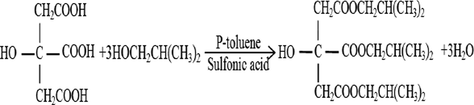
- The chemical reaction equation of citric acid and isopentyl alcohol.
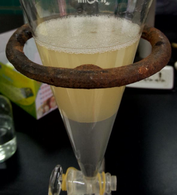
- The mixture after adding saturated sodium bicarbonate.
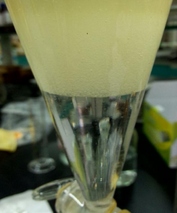
- The mixture after adding saturated sodium chloride.
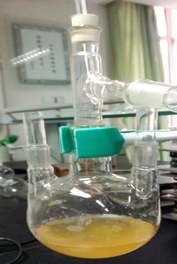
- The citric acid isopentyl ester obtained after distillation.
2.2 Experimental apparatus
The long slim tube displacement experimental apparatus mainly included: the piston container of carbon dioxide, the piston container of simulated crude oil, the piston container of formation water, slim tube filled with quartz sand particles, liquid flowmeter, gas flowmeter, back pressure control valve, etc. The sketch of experimental apparatus and flow chart are shown in Fig. 6.The sketch of long slim tube displacement experimental apparatus.
The injection pump was ISCO full automatic pump. The working pressure range was 0–70 MPa and the accuracy was 0.01 ml. The working pressure range of back pressure control valve was −70 MPa. The maximum temperature of constant temperature box was 200 °C. The accuracy of gas flowmeter was 1 ml. The long slim tube was a one-dimensional artificial porous medium spiral stainless steel coil tightly filled with approximately 200-mesh pure quartz sand. The experimental parameters are shown in Table 2.
Main parameters
Numerical values
Length (cm)
2000
Inner diameter (cm)
0.387
Outer diameter (cm)
0.637
Filler (pure quartz sand) (mesh)
185–230
Permeability measured with gas (μm2)
5.97
Porosity (%)
43.26
Maximum pressure (MPa)
70
Maximum temperature (°C)
200
2.3 The determination of the minimum miscible pressure
Experimental preparation
① Temperature setting: Turn on the power switch of the constant temperature box and heat up. When the temperature was close to the formation temperature, start the constant temperature controller. After 3 h, the temperature can be completely maintained at the predetermined value.
② Tube cleaning: Saturate the slim tube model adequately with petroleum ether, and use high-pressure air to dry the petroleum ether.
③ Carbon dioxide introduction: Open the pump and push the piston back to the top of the piston container to vent the gas from the container; inject the displacing gas carbon dioxide into the piston container and close the valve.
④ The long slim tube should be vacuumized. Then saturate the formation water into the sand-filled slim tube and calculate the porosity.
⑤ Clean the formation water in the sand-filled slim tube with toluene. Dry it in a thermostat to evaporate the toluene.
⑥ Saturate the simulated oil into the sand-filled slim tube.
Inject the simulated oil into the slim tube model with the ISCO constant-pressure and constant-speed pump. Stop the injection when the 1.5 PV simulated oil was injected. Calculate the injection and output of simulated oil, and determine the amount of saturated oil injected into the tube according to the volume difference. Then end the process and prepare for a displacement experiment.
Start the ISCO constant-pressure and constant-speed pump to increase the pressure of gas in the piston container, and make it 1–3 MPa lower than the displacement pressure.
Raise the back pressure to the predetermined displacement pressure through a manual pump.
Open the pump to inject the reagent (citric acid isopentyl ester).
Open the pump to inject carbon dioxide at a constant pressure, open the slim tube outlet valve, and adjust the gas pressure to make it equal to or slightly higher than the displacement pressure.
In the process of displacement, determine oil production, gas volume and pump reading regularly as required, and check oil sample saturation. When the cumulative volume of the gas drive was greater than 1.2 PV, stop the displacement and calculate the recovery under this pressure.
At the end of the displacement process, start the cleaning process, inject the petroleum ether is directly into the slim tube, and keep the appropriate back pressure. The flow rate of petroleum ether in the slim tube should not be too fast, so as not to affect the cleaning effect. Close the outlet for 1–2 h, and make the petroleum ether and residual oil fully contact each other at high pressure. Dissolve the residual oil in the petroleum ether, and release it from the outlet. Repeatedly, the mixture of petroleum ether and residual oil was discharged. When the mixture and the component of pure petroleum ether were basically the same, the residual oil in the model was determined to be completely removed and fully saturated with petroleum ether. Then, high-pressure air was injected from the inlet, the remaining petroleum ether in the slim tube was blown into the oil–gas separator, and the pipeline was dried for the next experiment.
Follow the above steps for displacement at the next pressure point.
In general, the minimum miscible pressure can be analyzed by measuring the recovery at more than 5 pressure points. (Ghorbani et al., 2019; Yu et al., 2020; Berneti and Varaki, 2018; Ma et al., 2018) The experimental back pressures in experiment were 10 MPa, 15 MPa, 20 MPa, 25 MPa, 30 MPa, 35 MPa and 40 MPa. The slug sizes of the reagent were 0.001 PV, 0.002 PV, 0.003 PV, 0.004 PV, respectively. The recoveries at different pressures were measured after the reagent with different slug sizes was injected. Then the minimum miscible pressure was calculated for different slug sizes, and the optimal slug size was determined according to the relationship between the minimum miscible pressure and the slug size.
2.4 The results after injecting citric acid isopentyl ester slug
The experimental results of the minimum miscible pressure when the injected citric acid isopentyl ester slug size were 0.001 PV, 0.002 PV, 0.003 PV, 0.004 PV are shown in Table 3.
Pressure (MPa)
Recovery (%)
0.001 PV
0.002 PV
0.003 PV
0.004 PV
10
59.7
60.0
62.9
65.1
15
68.4
69.1
72.6
74.2
20
76.2
79.3
82.2
83.3
25
84.9
88.1
90.2
90.3
30
90.2
90.5
90.8
90.9
35
91.1
91.5
91.6
91.7
40
91.6
91.9
92.1
92.5
The relation curves between the recovery and the experimental pressure under different injection slug sizes are shown in Figs. 7–10.Relationship diagram between pressure and recovery at 0.001 PV slug size.
Relationship diagram between pressure and recovery at 0.002 PV slug size.
Relationship diagram between pressure and recovery at 0.003 PV slug size.
Relationship diagram between pressure and recovery at 0.004 PV slug size.
As seen from Figs. 7–10, when the citric acid isopentyl ester with slug sizes of 0.001 PV, 0.002 PV, 0.003 PV and 0.004 PV was injected, the minimum miscible pressures of crude oil and carbon dioxide measured were 28.2 MPa, 25.9 MPa, 24.1 MPa and 23.7 MPa. Compared with the minimum miscible pressure of 29.6 MPa without injected citric acid isopentyl ester, the reductions of the minimum miscible pressure were 1.4 MPa, 3.7 MPa, 5.5 MPa and 5.9 MPa. The reduction rates were 4.73%, 12.50%, 18.58% and 19.93%, respectively. From this, we can obtain the relationship between the minimum miscible pressure and the slug size of citric acid isopentyl ester (Table 4 and Fig. 11).
Slug Size (PV)
0.001
0.002
0.003
0.004
MMP (MPa)
28.2
25.9
24.1
23.7
Relationship diagram between the MMP and the injected slug size of citric acid isopentyl ester.
As seen from Fig. 11, the minimum miscible pressure of crude oil and carbon dioxide measured gradually decreased with the increase of the injected slug of citric acid isopentyl ester, but the decrease became smaller and smaller. The optimal injected slug size of citric acid isopentyl ester was 0.003 PV. In this case, the minimum miscible pressure measured was 24.1 MPa. Compared with the minimum miscible pressure of 29.6 MPa without injected citric acid isopentyl ester, the reduction range was 5.5 MPa.
3 Miscible flooding experiment with citric acid isopentyl ester
3.1 Experimental materials of miscible flooding
Experimental fluid: the simulated oil for the experiment was prepared from the ground crude oil and natural gas in the research area according to the formation conditions and fluid characteristics. The chemical reagent injected was citric acid isopentyl ester. The purity of carbon dioxide was 99.9%, and the properties were exactly the same as that of carbon dioxide injected in the research area. The experimental temperature was 108.5 °C.
Experimental core: the specific parameters are shown in Table 5.
Scheme number
Core number
Permeability (10−3 µm2)
Core length (cm)
Core diameter (cm)
Porosity (%)
1
A-1
4.13
30
2.5
12.06
2
A-2
4.21
30
2.5
12.11
3
A-3
4.26
30
2.5
12.13
4
A-4
4.45
30
2.5
12.24
3.2 Experimental apparatus of miscible flooding
Experimental apparatus of miscible flooding between crude oil and carbon dioxide are shown in Fig. 12.The sketch of core displacement experimental apparatus. 1-ISCO pump; 2-ISCO pump; 3-a piston container of CO2; 4-a piston container of chemicals; 5, 8-core holder; 6-six-port; 7-pressure gauge; 9-back-pressure valve; 10-liquid metering device; 11-gas metering device; 12-constant temperature box.
3.3 Experimental schemes of miscible flooding
The experimental schemes of miscible flooding are shown in Table 6.
Scheme number
Content of scheme
1
Water drive to water content of 98%+
—
+1.2 PV CO2
2
0.001 PV citric acid isopentyl ester
3
0.002 PV citric acid isopentyl ester
4
0.003 PV citric acid isopentyl ester
3.4 Experimental procedure of miscible flooding
The artificial simulated core was saturated with simulated formation water and the porosity was measured.
The saturated core was saturated with simulated oil, and oil flooding was carried out until no water flowed out at the core outlet end. Then the irreducible water saturation was calculated. The core saturated with simulated oil was connected to the experimental apparatus according to the diagram of carbon dioxide miscible flooding experimental apparatus.
The CO2 injection pressure was adjusted to the displacement pressure. The experimental back pressure was set as 24.1 MPa, the minimum miscible pressure of crude oil and carbon dioxide when the injected slug of citric acid isopentyl ester was 0.003 PV. The displacement pressure difference was 2 MPa. The constant pressure displacement experiment was carried out according to each experimental scheme until the water cut reached 98%. During this process, the volume of the displaced oil was measured and the final recovery was calculated.
3.5 Experimental results of miscible flooding
Experimental results of miscible flooding are shown in Table 7.
Scheme number
Core number
Recovery of water flooding (%)
Recovery of miscible flooding (%)
Final recovery (%)
1
A-1
35.7
5.2
40.9
2
A-2
36.1
11.6
47.7
3
A-3
34.8
13.7
48.5
4
A-4
36.8
15.5
52.3
As shown from the Table 7, the final recoveries of each scheme were 40.9%, 47.7%, 48.5%, 52.3%, respectively; the recoveries in the water flooding stage were 35.7%, 36.1%, 34.8%, 36.8%; the recoveries in the miscible flooding stage were 5.2%, 11.6%, 13.7%, 15.5%. After adding the citric acid isopentyl ester slug, the final recovery increased significantly. The final recoveries of citric acid isopentyl ester were 6.8%, 7.6%, and 11.4% higher than that of the Scheme 1 when the injected slug sizes were 0.001 PV, 0.002 PV and 0.003 PV. The recoveries in the miscible flooding stage of Schemes 2, 3 and 4 were 6.4%, 8.5% and 10.3% higher than that of Scheme 1. The results of miscible flooding experiments show that the addition of citric acid isopentyl ester slug can significantly improve the oil displacement effect of carbon dioxide miscible flooding. Under the same experimental conditions, the injection of citric acid isopentyl ester can reduce the minimum miscible pressure, expand the range of miscible flooding, and thus significantly improve the recovery.
4 Conclusions
The minimum miscible pressure experimental schemes for injection of citric acid isopentyl ester with different slug sizes were established and completed. The experimental results show that with the increase of the injected slug size of citric acid isopentyl ester, the minimum miscible pressure gradually decreased, but the decrease became smaller and smaller. The optimal size of the injected slug is 0.003 PV. At such slug size, the minimum miscible pressure measured after injection of citric acid isopentyl ester slug was 24.1 MPa, 5.5 MPa lower than 29.6 MPa without slug injection.
The experimental schemes of miscible flooding after injection of citric acid isopentyl ester slug were developed and completed. The experimental results show that in conventional carbon dioxide miscible flooding scheme without citric acid isopentyl ester, the recovery in the miscible flooding stage was 5.2%, the recovery in the water flooding stage was 35.7%, and the total recovery was 40.9%. Scheme 4 showed the highest recovery in the miscible flooding stage: The water cut was 98% by water flooding, the citric acid isobutyl ester was injected with slug size of 0.003 PV, and CO2 was injected with 1.2 PV. The recovery of this scheme in miscible flooding stage was 15.5%, which was 10.3% higher than 5.2% of the conventional carbon dioxide miscible flooding scheme. The research findings apply to the oilfields with similar properties of crude oil and injected CO2.
Acknowledgements
This work was supported by the National Natural Science Foundation of China (42002154, 51834005), the Natural Science Foundation of Heilongjiang Province (E2016008), the Youths Science Foundation of Northeast Petroleum University (2019QNL-15) and the Open Fund project of Heilongjiang Key Laboratory of Oil and Gas Reservoir Formation Mechanism and Resource Evaluation (KL20190101). The authors would like to thank the editor and the reviewers of this paper for their valuable comments that helped improve the quality of the paper.
Data availability statement
The data that supports the findings of this study are available within the article.
Declaration of Competing Interest
The authors declare that they have no known competing financial interests or personal relationships that could have appeared to influence the work reported in this paper.
References
- A reliable strategy to calculate minimum miscibility pressure of CO2-oil system in miscible gas flooding processes. Fuel. 2017;208:117-126.
- [Google Scholar]
- A novel method for application of nanoparticles as direct asphaltene inhibitors during miscible CO2 injection. J. Petrol. Sci. Eng.. 2020;185:106661.
- [CrossRef] [Google Scholar]
- Development of ε-in sensitive smooth support vector regression for predicting minimum miscibility pressure in CO2 flooding. Songklanakarin J. Sci. Technol.. 2018;40(1):53-59.
- [Google Scholar]
- Advances and challenges in understanding the electrocatalytic conversion of carbon dioxide to fuels. Nat. Energy. 2019;4:732-745.
- [Google Scholar]
- Chen, F., He, J., Guo, P., 2012. A method used for reducing the minimum miscible pressure between oil and CO2 in miscible flooding. Chinese Patent 102337874A, 2.
- An improved correlation to determine minimum miscibility pressure of CO2–oil system. Green Energy Environ.. 2020;5:97-104.
- [Google Scholar]
- Estimation of the CO2-oil minimum miscibility pressure for enhanced oil recovery. Petrol. Sci. Technol.. 2016;34(22):1847-1854.
- [Google Scholar]
- Surfactants and self-assembly in carbon dioxide. Curr. Opin. Solid State Mater. Sci.. 2001;5(4):333-341.
- [Google Scholar]
- MMP of supercritical carbon dioxide microemulsion and alkanes. J. Petrochem. Univ.. 2013;26(1):40-44.
- [Google Scholar]
- A study of the mechanism of enhancing oil recovery using supercritical carbon dioxide microemulsions. Pet. Sci.. 2013;10(1):91-96.
- [Google Scholar]
- Capacity investigation of brine-bearing sands of the Frio formation for geologic storage of CO2. J. Oil Gas. 2001;12(9):11-15.
- [Google Scholar]
- The use of optimization procedures to estimate minimum miscibility pressure. Petrol. Sci. Technol.. 2014;32(8):947-957.
- [Google Scholar]
- Minimum miscibility pressure and interfacial tension measurements for N2 and CO2 gases in contact with W/O emulsions for different temperatures and pressures. Fuel. 2018;225:623-631.
- [Google Scholar]
- Toward the development of “CO2-philic” hydrocarbons. 1. Use of side-chain functionalization to lower the miscibility pressure of polydimethylsiloxanes in CO2. J. Phys. Chem. B. 1999;103(31):6441-6444.
- [Google Scholar]
- Describing a strategy to estimate the CO2-heavy oil minimum miscibility pressure based on the experimental methods. Energy Source Part A. 2019;41(17):2083-2093.
- [Google Scholar]
- Enhanced shale oil recovery by the huff and puff method using CO2 and cosolvent mixed fluids. Energy Fuels. 2020;34:1438-1446.
- [Google Scholar]
- Optimization and oil displacement efficiency of non-ionic low molecular surfactant. Oil Drill. Prod. Technol.. 2012;34(2):81-84.
- [Google Scholar]
- Use of oil-soluble surfactant to reduce minimum miscibility pressure. Petrol. Sci. Technol.. 2017;35(4):345-350.
- [Google Scholar]
- Main control factors of carbon dioxide on swelling effect of crude hydrocarbon components. Acta Petrolei Sinica. 2016;37(3):392-398.
- [Google Scholar]
- Water-in-carbon dioxide microemulsions with a fluorocarbon-hydrocarbon hybrid surfactant. Langmuir. 1994;10(10):3536-3541.
- [Google Scholar]
- A rapid method for determining CO2/oil MMP and visual observations of CO2/oil interactions at reservoir conditions. Energy Procedia. 2014;63:7724-7731.
- [Google Scholar]
- The incorporation of a fluorinated ether functionality into a polymer or surfactant to enhance CO2-solubility. J. Supercrit. Fluids. 1992;5(4):237-241.
- [Google Scholar]
- Solubility of nonionic surfactants used in CO2 miscible flooding. Dalian University of Technology; 2014. Master Thesis
- Oil price regimes and their role in price diversions from market fundamentals. Nat. Energy. 2020;5:141-149.
- [Google Scholar]
- Four methods to estimate minimum miscibility pressure of CO2-oil based on machine learning. Chin. J. Chem.. 2019;37:1271-1278.
- [Google Scholar]
- The design and analysis of a coaxial casing heat exchanger used in geothermal energy systems. Energy Sources, Part A: Recov. Utiliz. Environ. Effects 2020
- [CrossRef] [Google Scholar]
- Comparison and dynamic models of supercritical CO2 extraction enhanced by different technologies. Modern Food Sci. Technol.. 2013;29(8):1921-1925.
- [Google Scholar]
- In situ fabrication and reactivation of highly selective and stable Ag catalysts for electrochemical CO2 conversion. ACS Energy Lett.. 2018;3:1301-1306.
- [Google Scholar]
- A novel protocol for estimation of minimum miscibility pressure from slimtube experiments. J. Petrol. Sci. Eng.. 2016;146:545-551.
- [Google Scholar]
- Modeling of asphaltene precipitation in calculation of minimum miscibility pressure. Ind. Eng. Chem. Res.. 2017;56:7375-7383.
- [Google Scholar]
- CO2 and alkane minimum miscible pressure estimation by the extrapolation of interfacial tension. Fluid Phase Equilibr.. 2019;494:103-114.
- [Google Scholar]
- A new method of reducing the miscible-phase pressure of CO2 flooding. J. Chongqing Univ. Sci. Technol. (Nat. Sci. Ed.). 2012;14(1):48-51.
- [Google Scholar]
- Application and enlightenment of carbon dioxide flooding in the United States of America. Petrol. Explor. Dev.. 2015;42(2):209-216.
- [Google Scholar]
- Numerical simulation of CO2 separation from gas mixtures in membrane modules: Effect of chemical absorbent. Arab. J. Chem.. 2016;9(1):62-71.
- [CrossRef] [Google Scholar]
- Stubby surfactants for stabilization of water and CO2 emulsions: trisiloxanes. Langmuir. 2003;19(8):3114-3120.
- [Google Scholar]
- Flexible porous silicone rubber-nanofiber nanocomposit- es generated by supercritical carbon dioxide foaming for harvesting mechanical energy. Nano Energy. 2020;67:104290.
- [CrossRef] [Google Scholar]
- D-optimal design for rapid assessment model of CO2 flooding in high water cut oil reservoirs. J. Nat. Gas Sci. Eng.. 2014;21(11):764-771.
- [Google Scholar]
- Molecular dynamics of methane flow behavior through realistic organic nanopores under geologic shale condition: Pore size and kerogen types. Chem. Eng. J.. 2020;398:124341.
- [Google Scholar]
- Chemical constituents and nitric oxide inhibitory activity of supercritical carbon dioxide extracts from Mitragyna speciosa leaves. Arab. J. Chem.. 2016;12(3):350-359.
- [CrossRef] [Google Scholar]
- Comparative evaluation of immiscible, near miscible and miscible CO2 huff-n-puff to enhance oil recovery from a single matrix-fracture system (experimental and simulation studies) Fuel. 2012;93(4):443-453.
- [Google Scholar]
- Studies on the chemical agent system for reducing in the minimal miscibility pressure of CO2 flooding. China Sci. Pap.. 2015;10(18):2161-2164.
- [Google Scholar]
- Influence of confinement effect on recovery mechanisms of CO2–enhanced tight-oil recovery process considering critical properties shift, capillarity and adsorption. Fuel. 2020;262:116569.
- [CrossRef] [Google Scholar]
- Physical simulation of staged-fracturing horizontal wells using CO2 huff and puff in tight oil reservoirs. Acta Petrolei Sinica. 2015;36(6):724-729.
- [Google Scholar]
- Reducing the minimum miscibility pressure of CO2 and crude oil using alcohols. Colloid. Surf. A. 2019;568:105-112.
- [Google Scholar]
- Determination of minimum near miscible pressure region during CO2 and associated gas injection for tight oil reservoir in Ordos Basin. China. Fuel. 2020;263:116737.
- [CrossRef] [Google Scholar]
- An investigation on phase behaviors and displacement mechanisms of gas injection in gas condensate reservoir. Fuel. 2020;268
- [CrossRef] [Google Scholar]
- Study on reducing miscibility pressure in CO2 flooding by miscible solvent. Special Oil Gas Reserv.. 2013;20(2):115-117.
- [Google Scholar]
- Performance evaluation and mechanism with different CO2 flooding modes in tight oil reservoir with fractures. J. Petrol. Sci. Eng.. 2020;188:106950.
- [CrossRef] [Google Scholar]